Gibbs Phase Rule
Summary
TLDRThis script delves into the concept of chemical equilibrium, explaining how the chemical potential of components equates across phases during equilibrium. It explores the constraints on the number of thermodynamic properties that can be specified simultaneously, focusing on single and multi-component systems. The Gibbs Phase Rule is introduced, providing a formula to calculate degrees of freedom in systems with multiple components and phases, crucial for understanding phase diagrams and equilibrium conditions in complex systems.
Takeaways
- 🔍 The chemical potential of each component in a system at equilibrium must be equal in all phases.
- 📊 Equilibrium conditions help determine the coexistence lines on a phase diagram and constrain the number of thermodynamic properties that can be specified simultaneously.
- 🌡️ For a single-component system, the number of degrees of freedom is 3 minus the number of phases, meaning two degrees of freedom for a single phase system (temperature and pressure).
- 🤔 In a multi-component system, the degrees of freedom are more complex to determine and depend on the number of components and phases present.
- 🌪️ An example of a two-component, single-phase system is air, composed of nitrogen and oxygen, where three degrees of freedom can be specified: temperature, pressure, and composition.
- 💧 The introduction of a second phase, such as in carbonated water, complicates the degrees of freedom due to additional constraints from phase coexistence and composition.
- 🧪 In a two-component, two-phase system like carbonated water, only two degrees of freedom can be independently specified due to the constraints of phase equilibrium and composition.
- 🔄 The phase equilibrium imposes constraints where the chemical potentials of components must be equal across phases, affecting the degrees of freedom.
- 📐 The Gibbs Phase Rule (d = c - φ + 2) can be used to calculate the degrees of freedom for any multi-component system with multiple phases, where 'c' is the number of components, 'φ' is the number of phases, and 'd' is the degrees of freedom.
- 📝 The total number of potential degrees of freedom is the sum of thermodynamic variables and composition variables (c*φ + 2), but this number is reduced by compositional and phase equilibrium constraints.
- 📉 The degrees of freedom are ultimately determined by the constraints that reduce the number of independently specifiable variables in a system at equilibrium.
Q & A
What is the significance of chemical potential being equal in each phase at equilibrium?
-The equality of chemical potential in each phase at equilibrium is crucial because it not only helps describe the coexistence lines on a phase diagram but also places constraints on the number of properties that can be specified simultaneously for a system.
What is the relationship between the number of degrees of freedom and the number of phases in a single-component system?
-For a single-component system, the number of degrees of freedom is equal to 3 minus the number of phases. This means for a single-phase system, you can specify two degrees of freedom (temperature and pressure), while for a system with phase coexistence, you can only specify one (either temperature or pressure).
How does the number of components in a system affect the degrees of freedom?
-In a multi-component system, the degrees of freedom are affected by the additional constraints imposed by the composition of the mixture. You can specify the composition (mole fraction) of each component, but these cannot be specified independently due to the constraint that the sum of mole fractions must equal 100%.
What is an example of a two-component, single-phase system, and how many degrees of freedom does it have?
-An example of a two-component, single-phase system is air, considered as a mixture of nitrogen (N2) and oxygen (O2). This system has three degrees of freedom, which can be the temperature, pressure, and the mole fraction of one of the components, since the other is determined by the constraint that the mole fractions must sum to one.
How does the presence of multiple phases in a system affect the degrees of freedom?
-In a system with multiple phases, the degrees of freedom are reduced due to the additional constraints imposed by the phase equilibrium. For example, in a two-component, two-phase system like carbonated water, you can only independently specify two variables because the partial pressures and mole fractions are interdependent.
What is the Gibbs Phase Rule, and how does it help in predicting the degrees of freedom in a system?
-The Gibbs Phase Rule is a formula that predicts the degrees of freedom (F) in a system with multiple components and phases. It is given by F = C - Φ + 2, where C is the number of components and Φ is the number of phases. This rule helps in understanding how many independent variables can be specified in a system at equilibrium.
What are the types of constraints that reduce the degrees of freedom in a multi-component, multi-phase system?
-The constraints that reduce the degrees of freedom include compositional constraints (e.g., mole fractions or partial pressures summing to a total) and phase equilibrium constraints (e.g., chemical potentials being equal across phases for each component).
Can you explain the compositional constraints in a multi-component system?
-Compositional constraints in a multi-component system are rules that state the sum of mole fractions of all components in a phase must equal one, or the sum of partial pressures in a vapor phase must equal the total pressure. These constraints limit the independent specification of the composition variables.
What is the significance of the phase equilibrium constraint in a multi-component system?
-The phase equilibrium constraint is significant because it ensures that the chemical potentials of the same component in different phases are equal at equilibrium. This constraint links the properties of different phases and reduces the number of independent variables that can be specified.
How does the presence of a solid phase in a system affect the degrees of freedom?
-The presence of a solid phase adds another dimension to the system's constraints. For each component, there would be an additional constraint equating its chemical potential in the solid phase with those in the other phases. This further reduces the degrees of freedom in the system.
Can you provide a practical example illustrating the application of the Gibbs Phase Rule?
-Consider a system with three components (A, B, and C) and three phases (solid, liquid, and gas). According to the Gibbs Phase Rule, the degrees of freedom would be F = 3 - 3 + 2 = 2. This means you can independently specify only two variables for the system, such as temperature and pressure, while all other properties are determined by these and the system's composition.
Outlines
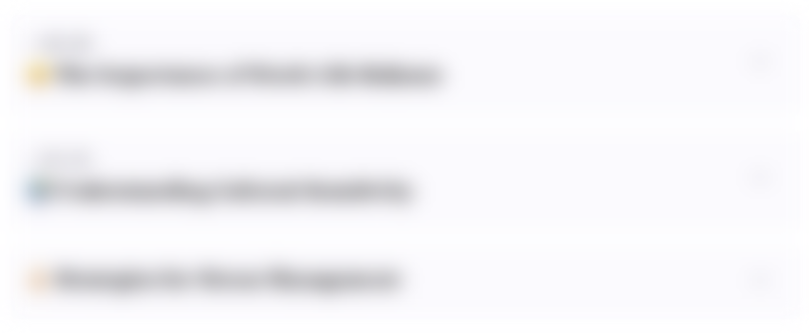
Esta sección está disponible solo para usuarios con suscripción. Por favor, mejora tu plan para acceder a esta parte.
Mejorar ahoraMindmap
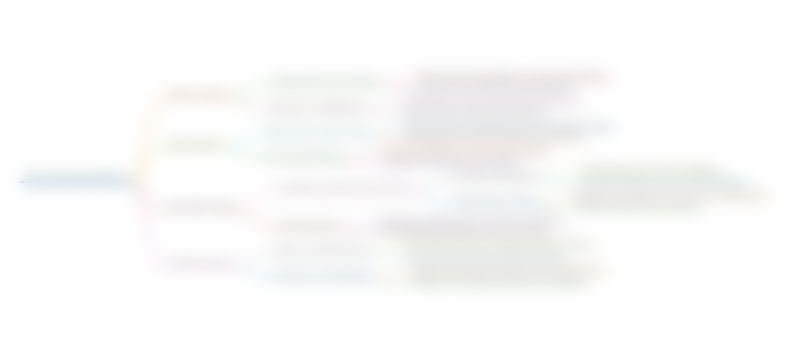
Esta sección está disponible solo para usuarios con suscripción. Por favor, mejora tu plan para acceder a esta parte.
Mejorar ahoraKeywords
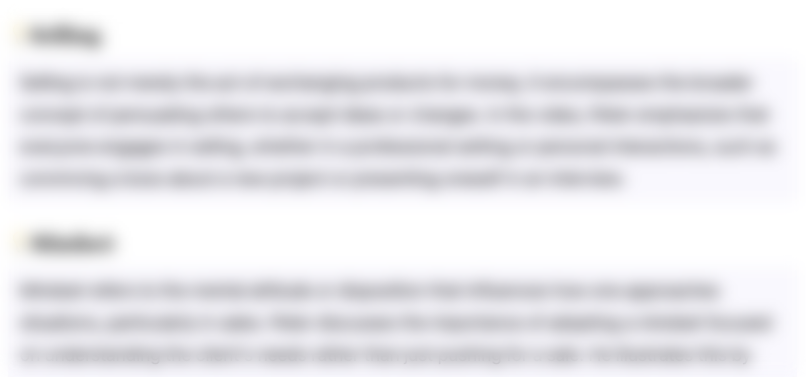
Esta sección está disponible solo para usuarios con suscripción. Por favor, mejora tu plan para acceder a esta parte.
Mejorar ahoraHighlights
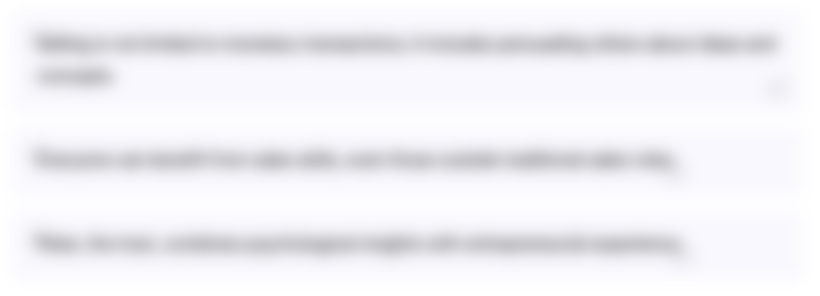
Esta sección está disponible solo para usuarios con suscripción. Por favor, mejora tu plan para acceder a esta parte.
Mejorar ahoraTranscripts
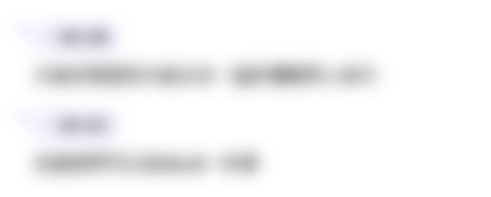
Esta sección está disponible solo para usuarios con suscripción. Por favor, mejora tu plan para acceder a esta parte.
Mejorar ahoraVer Más Videos Relacionados
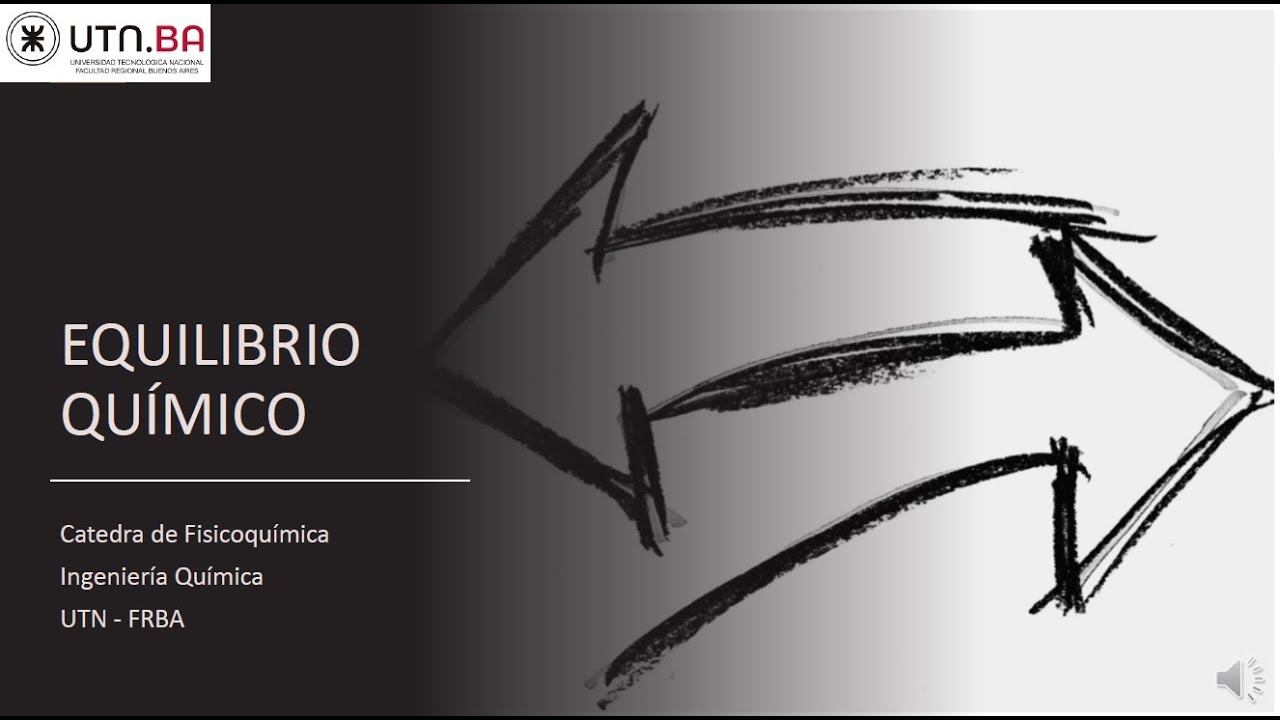
Clase nº 3 - 1. Equilibrio quimico: el avance de reacción y la constante de equilibrio

DERAJAT DISOSIASI REAKSI KESETIMBANGAN
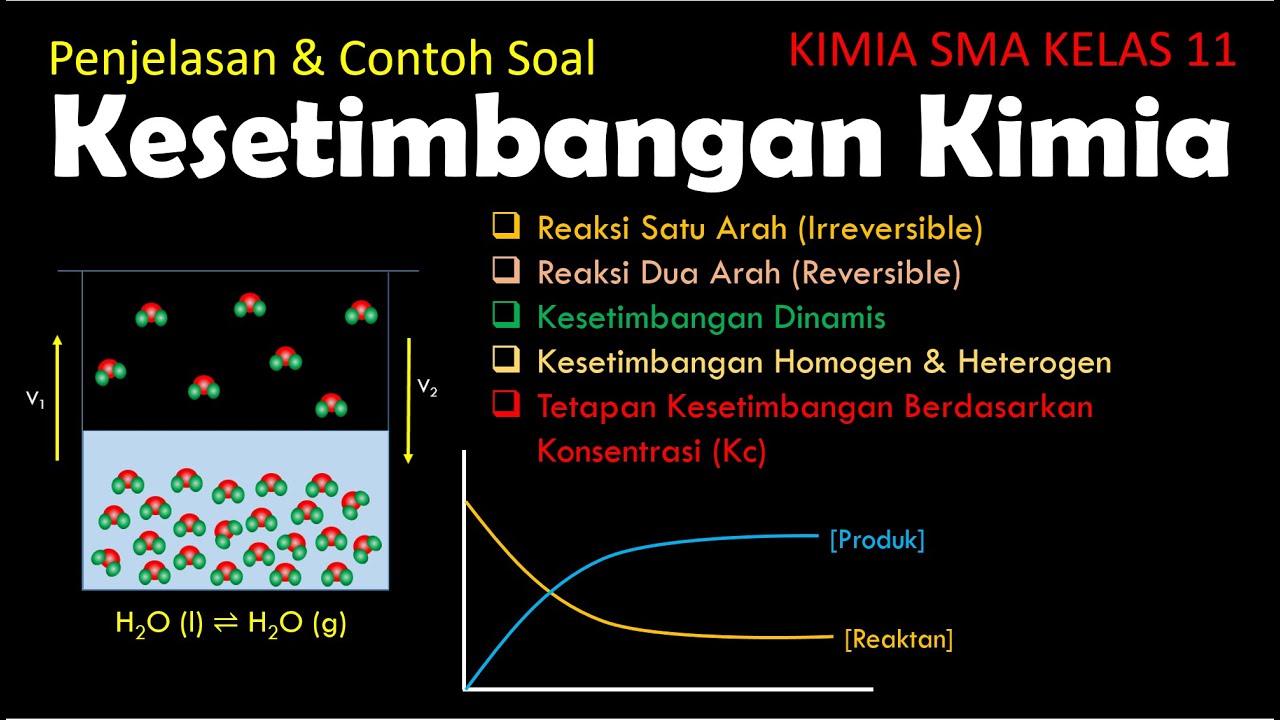
KESETIMBANGAN KIMIA ( KIMIA SMA KELAS 11 )
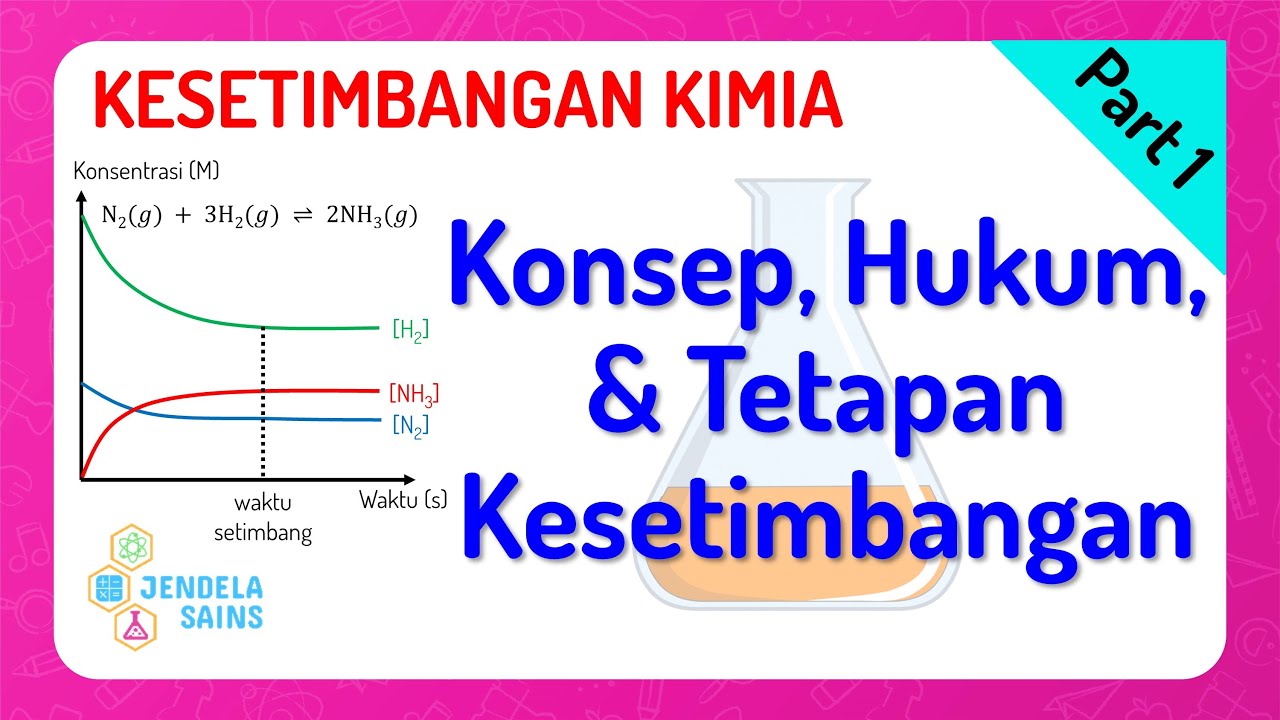
Kesetimbangan Kimia • Part 1: Konsep, Hukum, Tetapan Kesetimbangan Kc dan Kp
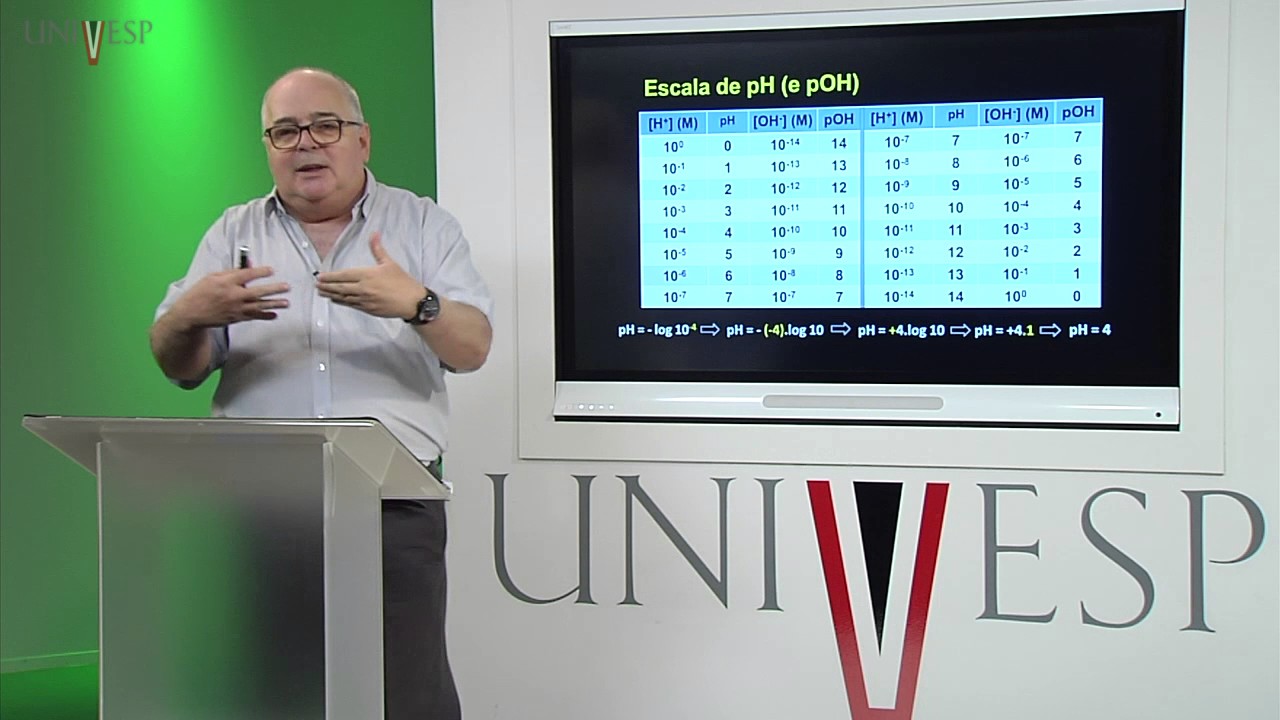
Bioquímica - Aula 03 - Alguns conceitos químicos importantes - 2
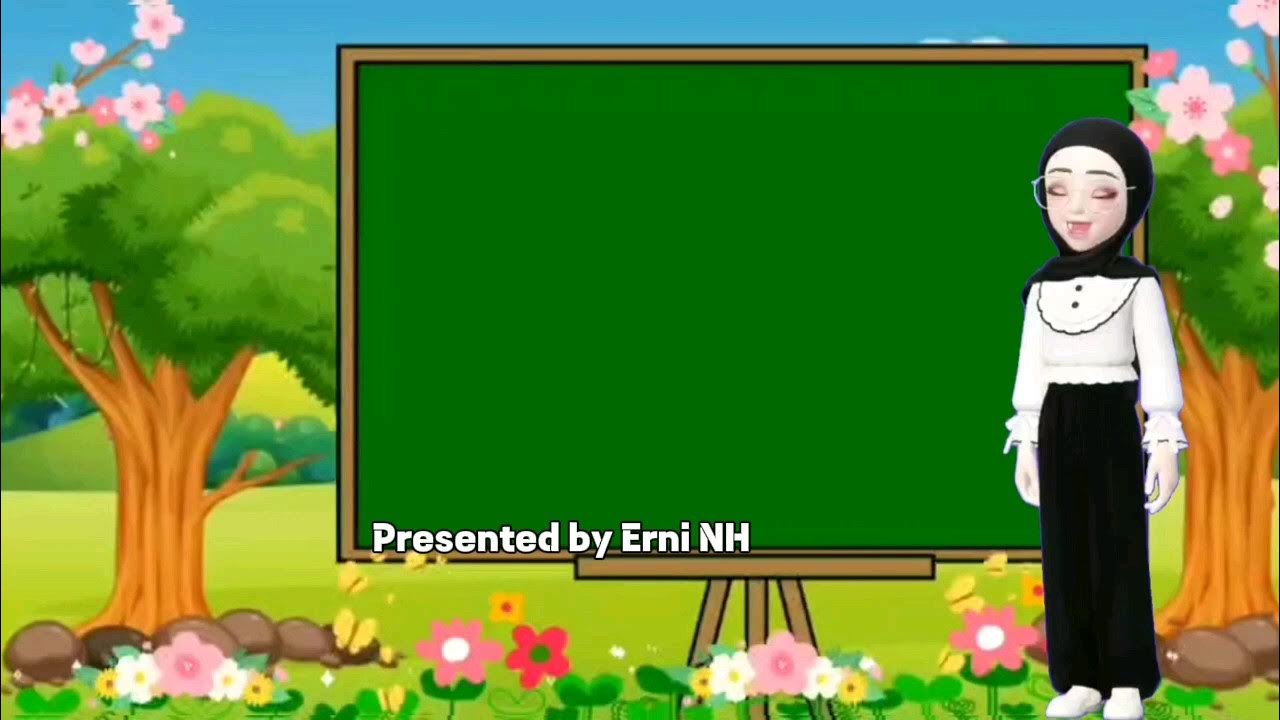
Animasi Kesetimbangan Kimia dalam Lingkup Kehidupan Sehari-hari
5.0 / 5 (0 votes)