Minority charge carriers in extrinsic semiconductors | Class 12 (India) | Physics | Khan Academy
Summary
TLDRThe script delves into the nuances of semiconductor doping, explaining how the addition of impurities like phosphorus (for n-type) or boron (for p-type) dramatically alters the material's conductive properties. It addresses questions about the band structure changes due to doping and clarifies why the intrinsic band structure remains applicable. The script also explores the math behind doping, showing how even a low concentration of dopants significantly increases free electrons and decreases holes, leading to a million-fold change in carrier concentration and emphasizing the unique properties of semiconductors that enable such manipulation.
Takeaways
- 🌟 Semiconductors can be altered by adding impurities, creating either n-type (with more electrons) or p-type (with more holes) materials.
- 🔬 Phosphorus, a group 15 element, when added to a semiconductor like silicon, donates an extra electron, resulting in an n-type semiconductor.
- 🔬 Boron, a group 13 element, when added, accepts electrons, creating more holes and resulting in a p-type semiconductor.
- 📚 The band structure of a semiconductor is influenced by the material's composition, but the addition of a low concentration of impurities does not significantly change the overall band structure.
- 🔍 Even though phosphorus atoms are added, their atomic orbitals do not overlap significantly due to the low doping concentration, maintaining the discrete energy level.
- 🔢 The doping concentration is typically very low, with about one phosphorus atom for every million silicon atoms, often referred to as 1 ppm (parts per million).
- 📈 The effect of doping is dramatic; a low concentration of impurities can drastically increase the number of charge carriers, such as electrons in n-type semiconductors.
- 🔄 In intrinsic semiconductors, the number of electrons and holes are equal, but in extrinsic semiconductors, the number of electrons can increase significantly while the number of holes decreases.
- 🧩 The recombination rate, which is the product of the number of electrons and holes, must balance the generation rate to maintain thermal equilibrium in semiconductors.
- 📉 The addition of impurities like phosphorus not only increases the number of electrons but also significantly reduces the number of holes, leading to a drastic change in the semiconductor's properties.
- 🚀 The ability to manipulate semiconductor properties through doping is unique to semiconductors and is a key reason for their use in various electronic devices and technologies.
Q & A
What is an intrinsic semiconductor?
-An intrinsic semiconductor is a pure semiconductor material that is undoped, meaning it does not have any impurities added to it. It has an equal number of electrons and holes, making it a neutral conductor with limited conductivity that can be manipulated through doping.
What is the role of phosphorus in an n-type semiconductor?
-Phosphorus, being a group 15 element with five valence electrons, is added to a semiconductor like silicon to create an n-type semiconductor. It donates its extra electron, increasing the number of negatively charged conduction electrons significantly compared to holes.
How does doping with boron create a p-type semiconductor?
-Boron, a group 13 element with three valence electrons, is added to a semiconductor to create a p-type semiconductor. It can accept electrons, leading to an increase in the number of holes, which are positive charge carriers.
Why does the band structure of an extrinsic semiconductor remain similar to the pure material?
-The band structure of an extrinsic semiconductor remains similar to the pure material because the doping concentration is kept very low. This means that the impurity atoms are sparsely distributed among the semiconductor atoms, and their effect on the overall band structure is minimal.
Why don't we consider the band structure of the dopant like phosphorus in an n-type semiconductor?
-The band structure of the dopant is not considered because the dopant atoms are so far apart that their atomic orbitals hardly overlap. The effect of each dopant atom is discrete and localized, justifying the use of a single donor level rather than a band.
What is the significance of the donor level being close to the conduction band in n-type semiconductors?
-The donor level being close to the conduction band allows the extra electrons from the dopant atoms to easily jump into the conduction band, increasing the number of free electrons available for conduction and enhancing the semiconductor's conductivity.
How does the number of electrons change when a semiconductor is doped to become an n-type?
-When a semiconductor is doped to become an n-type, the number of electrons increases dramatically due to the addition of dopant atoms like phosphorus, each contributing an extra electron. Even at a low doping concentration, this results in a significant increase in the number of conduction electrons.
What happens to the number of holes in an n-type semiconductor?
-In an n-type semiconductor, the number of holes decreases significantly due to the increased number of electrons. The higher electron concentration leads to a higher recombination rate, destroying many electron-hole pairs and reducing the hole concentration.
Why is the effect of doping so dramatic even with a low concentration of dopant atoms?
-The effect of doping is dramatic even at low concentrations because the intrinsic semiconductor starts with a relatively low number of charge carriers. Adding even a small number of dopant atoms can significantly alter the balance between electrons and holes, leading to a substantial change in the semiconductor's electrical properties.
How does the ratio of electrons to holes change in an extrinsic semiconductor?
-In an extrinsic semiconductor, the ratio of electrons to holes changes dramatically due to the addition of dopant atoms. For an n-type semiconductor, the number of electrons increases while the number of holes decreases, resulting in a very high ratio of electrons to holes, which can be in the order of millions or more.
Outlines
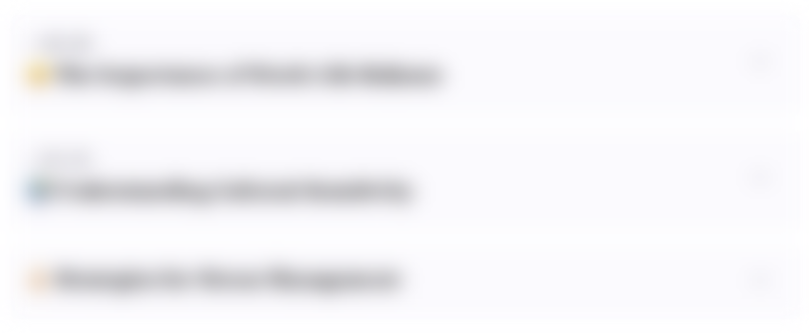
This section is available to paid users only. Please upgrade to access this part.
Upgrade NowMindmap
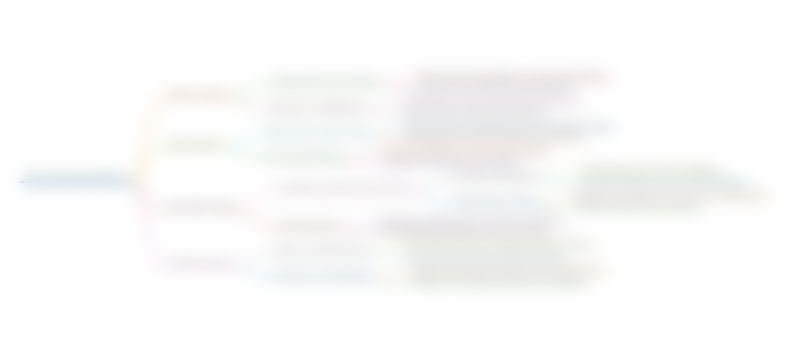
This section is available to paid users only. Please upgrade to access this part.
Upgrade NowKeywords
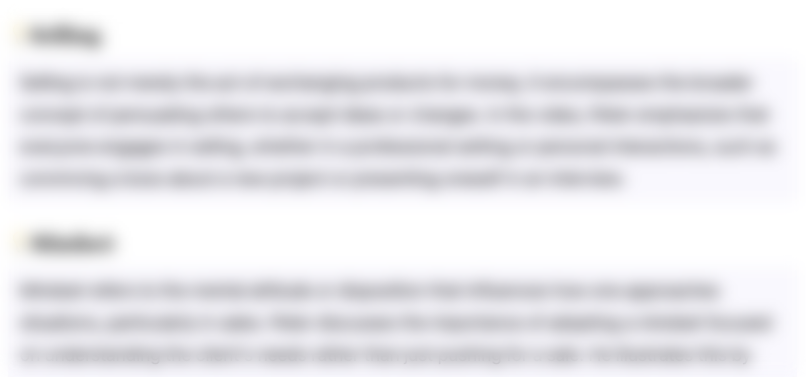
This section is available to paid users only. Please upgrade to access this part.
Upgrade NowHighlights
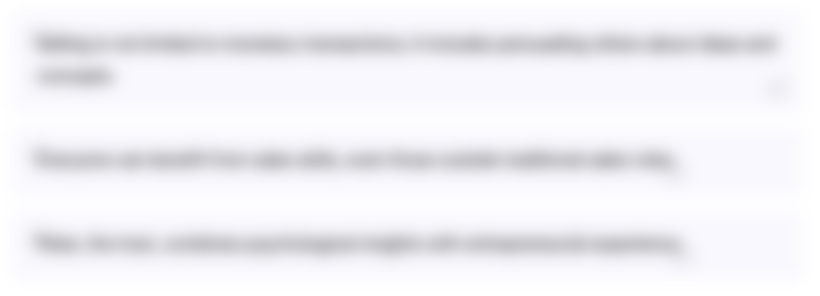
This section is available to paid users only. Please upgrade to access this part.
Upgrade NowTranscripts
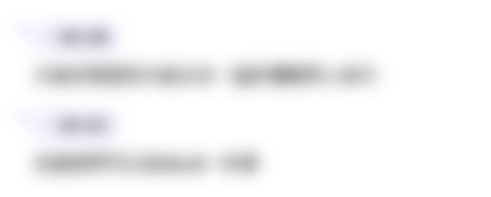
This section is available to paid users only. Please upgrade to access this part.
Upgrade NowBrowse More Related Video
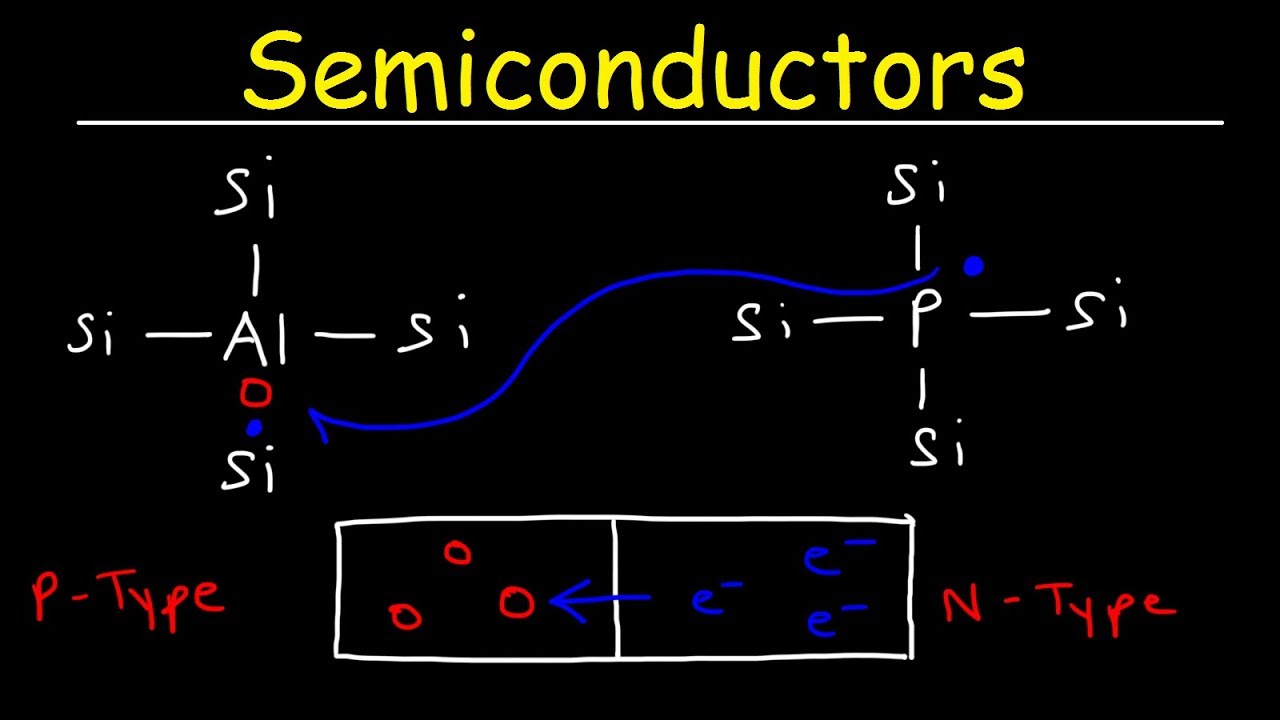
Semiconductors, Insulators & Conductors, Basic Introduction, N type vs P type Semiconductor

2 Semikonduktor Semikonduktor Tipe P dan N

Semiconductors - Physics inside Transistors and Diodes
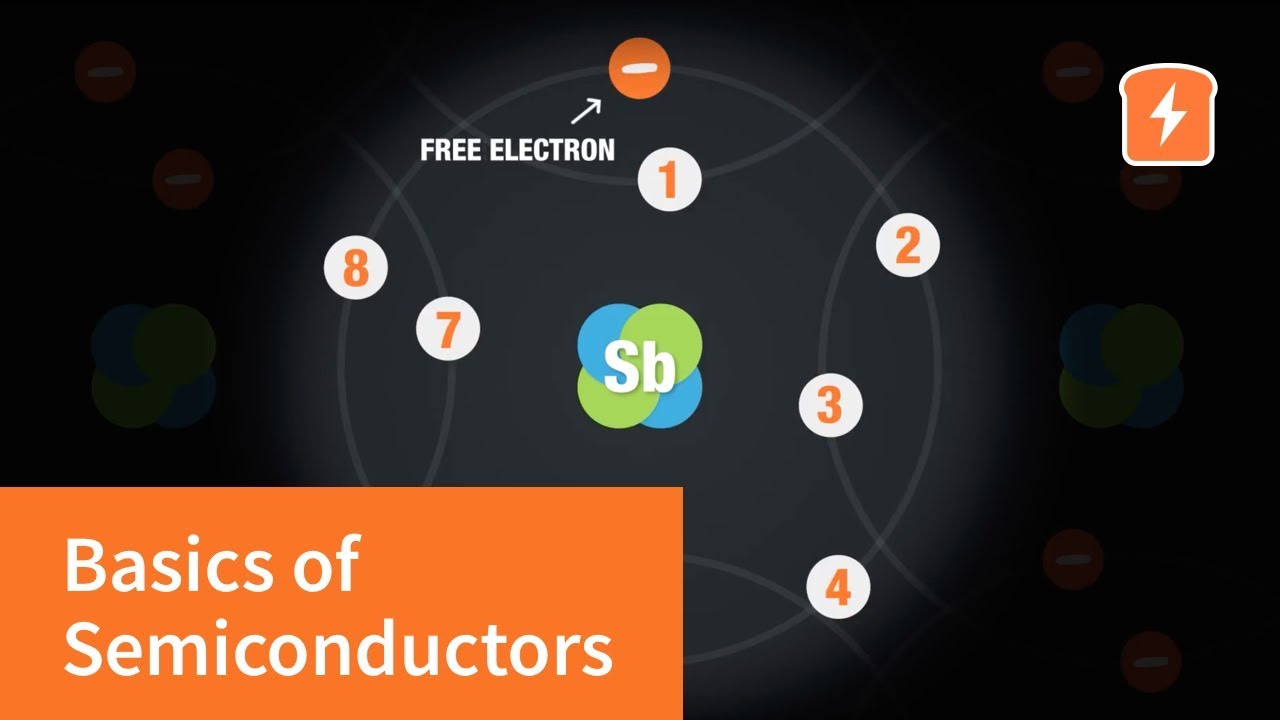
How do semiconductors work? (with animation) | Intermediate Electronics

Classification of Semiconductors (Intrinsic/Extrinsic, P-Type/N-Type)

Types of Semiconductor Materials | Intrinsic & Extrinsic Semiconductor | Engineering Funda
5.0 / 5 (0 votes)