10-Minute Neuroscience: Action Potentials
Summary
TLDRThis neuroscience video delves into the fascinating world of action potentials, the electrical impulses that travel along neurons, triggering neurotransmitter release. It begins by explaining the resting state of a neuron, highlighting the crucial role of ions and ion channels in creating the conditions for action potentials. The video outlines the process of depolarization, where neurotransmitters cause a positive ion influx, leading to the firing of an action potential. It then describes the propagation of this electrical signal down the neuron's axon, facilitated by the opening of sodium and potassium channels. The importance of myelin sheaths and nodes of Ranvier in speeding up signal transmission is also discussed. The video concludes by explaining the refractory periods that prevent immediate reactivation and ensure action potentials relate to the intensity of stimulation, adhering to the all-or-none law.
Takeaways
- π Neurons use action potentials to communicate, which are electrical impulses that travel along the neuron and trigger neurotransmitter release.
- π At rest, neurons have a specific electrical setup with a membrane potential of about -70 millivolts, due to differing concentrations of ions inside and outside the cell.
- π Key ions involved are sodium (Na+) and potassium (K+), with Na+ primarily outside and K+ inside the neuron.
- π‘οΈ Ion channels and the sodium-potassium pump are crucial in maintaining the ion balance across the neuron's membrane.
- π¨ Action potentials start with depolarization, where the membrane potential becomes less negative if the neuron's depolarization reaches a certain threshold.
- π₯ Voltage-gated sodium channels play a vital role; opening these channels causes Na+ to rush into the cell, further depolarizing it and propagating the action potential.
- π¨ The peak of an action potential is when the internal voltage swings to positive, driven by the inflow of sodium ions.
- π¬ Repolarization follows as potassium ions flow out, resetting the membrane potential back towards the resting state.
- π¬ Myelin sheaths enhance the speed and efficiency of action potential propagation along the axon through a process called saltatory conduction.
- π After an action potential, neurons experience a refractory period where they cannot fire again immediately, ensuring the action potential only moves in one direction.
Q & A
What are action potentials and why are they important for the nervous system?
-Action potentials are electrical impulses that travel down neurons, causing the release of neurotransmitters. They are critical for neural communication and essential to the function of the nervous system.
What is the resting state of a neuron in terms of its electrical properties?
-At rest, a neuron has a cell membrane that separates the intracellular environment from the extracellular environment. The resting state involves an unequal distribution of ions, with more positively charged sodium ions outside the neuron and more potassium ions inside.
How do ion channels contribute to the distribution of ions across the neuron's membrane?
-Ion channels are tubelike pores in the cell membrane that allow specific ions to pass through. They are selective for certain ions, and some are always open (leak channels), while others open only in response to stimuli such as neurotransmitter binding.
What is the role of the sodium-potassium pump in maintaining ion concentrations?
-The sodium-potassium pump is an enzyme that continuously pumps sodium ions out of the cell and potassium ions into the cell, helping to maintain a higher concentration of potassium ions inside and sodium ions outside the cell.
How does the resting membrane potential of a neuron typically measure?
-The resting membrane potential of a neuron is typically about -70 millivolts, indicating that the inside of the cell is more negatively charged than the outside.
What is depolarization and how does it relate to the generation of an action potential?
-Depolarization is the process where the membrane potential moves closer to 0, becoming less negative. It occurs when neurotransmitters bind to receptors, causing positively charged ions to flow into the neuron. If the depolarization reaches a threshold, an action potential will fire.
How do voltage-gated sodium channels contribute to the rising phase of an action potential?
-When a neuron is depolarized to threshold, voltage-gated sodium channels open, allowing positively charged sodium ions to rush into the cell. This influx further depolarizes the cell, causing more sodium channels to open, leading to a rapid change in membrane potential known as the rising phase.
What is the role of potassium ions and channels in ending an action potential?
-As the neuron's membrane potential reaches its peak, voltage-gated sodium channels close, and voltage-gated potassium channels open, allowing potassium to rush out of the cell. This helps to repolarize the neuron, moving it back to its resting membrane potential and ending the action potential.
How does myelin increase the speed of action potential propagation along the axon?
-Myelin is an insulating material that wraps around the axons of neurons. It prevents current from leaking out and has nodes of Ranvier, which are gaps in the myelin rich in voltage-gated sodium channels. This allows for the action potential to regenerate quickly at each node, increasing the speed of propagation.
What are the absolute and relative refractory periods, and how do they affect the firing of action potentials?
-The absolute refractory period is when voltage-gated sodium channels are unresponsive and the neuron cannot fire another action potential. The relative refractory period follows, during which the neuron is briefly hyperpolarized and requires stronger stimulation to fire another action potential. These periods ensure that action potential firing is related to the intensity of stimulation.
What is the all-or-none law regarding the amplitude of action potentials?
-The all-or-none law states that action potentials do not vary in size based on the intensity of a stimulus. They either fire or do not fire, and when they do, they are of a consistent amplitude or size.
How can more intense stimuli affect the frequency of action potential firing?
-More intense stimuli can cause more frequent firing of action potentials because they can overcome the relative refractory period in neurons, allowing for a higher rate of action potential generation despite the refractory periods.
Outlines
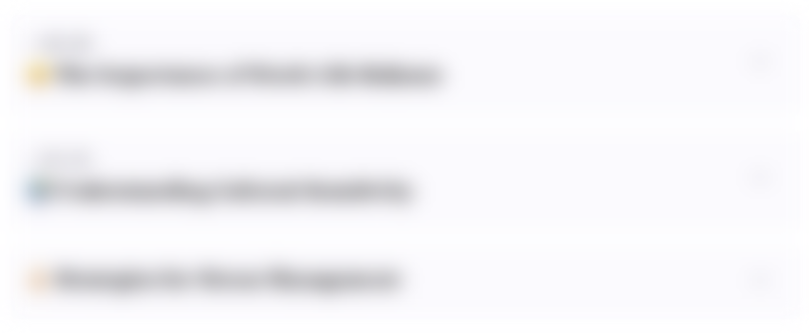
This section is available to paid users only. Please upgrade to access this part.
Upgrade NowMindmap
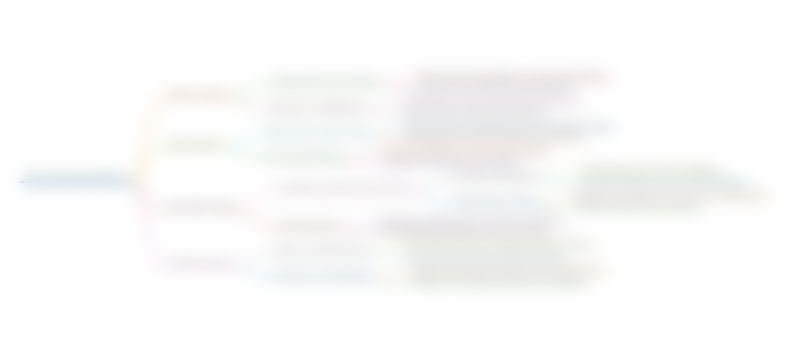
This section is available to paid users only. Please upgrade to access this part.
Upgrade NowKeywords
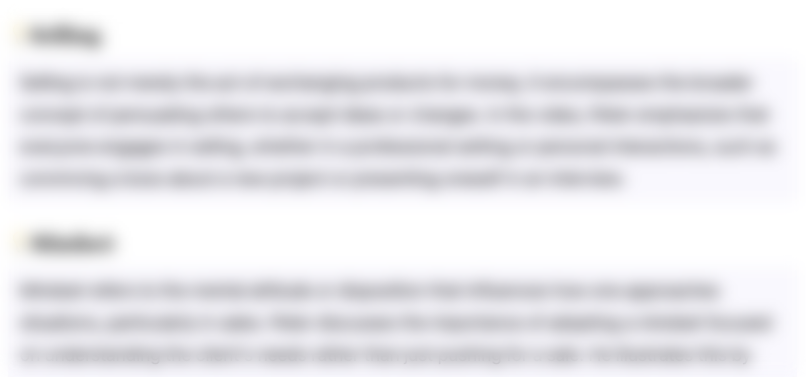
This section is available to paid users only. Please upgrade to access this part.
Upgrade NowHighlights
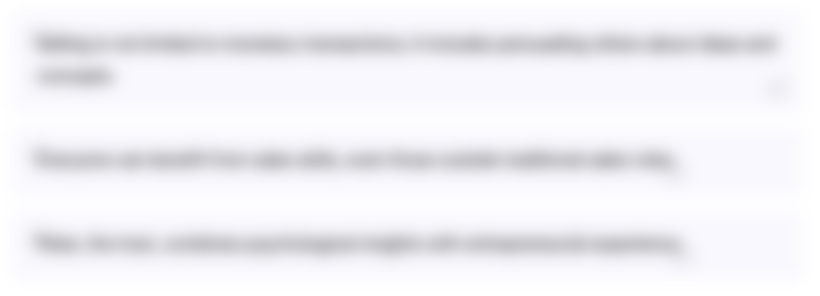
This section is available to paid users only. Please upgrade to access this part.
Upgrade NowTranscripts
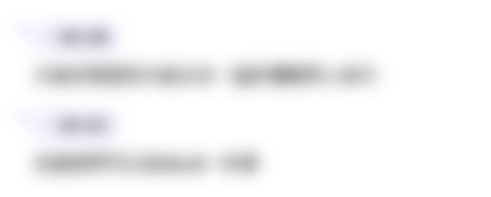
This section is available to paid users only. Please upgrade to access this part.
Upgrade NowBrowse More Related Video
5.0 / 5 (0 votes)