AKTU - QUANTUM MECHANICS for Engineering Physics
Summary
TLDRIn this lecture on Quantum Mechanics for first-year engineering physics students, the instructor covers key topics from the AKTU syllabus. The lecture begins with an introduction to Black Body Radiation, explaining the concept and its relation to Stephen's Law, which states that the energy radiated by a black body is proportional to the fourth power of its absolute temperature. The instructor then delves into the wave-particle duality of light and matter, emphasizing the importance of understanding the time-dependent and independent Schrödinger wave equations. The lecture concludes with a discussion on Compton Effect, providing the formula for the variation in wavelength when a particle undergoes this effect. The instructor also guides students on how to approach numerical problems in quantum physics, ensuring they understand the derivations and applications of these fundamental concepts.
Takeaways
- 📚 The lecture focuses on Unit 3 of the AKTU Engineering Physics syllabus, specifically on quantum mechanics.
- 🌑 Black body radiation is a central concept, with black bodies absorbing all radiation and emitting none.
- 🔢 Several important laws related to energy were discussed: Stefan's Law, Wien's Law, Rayleigh-Jeans Law, and Planck's Law, each with its own formula and principles.
- ⚛️ Wave-particle duality highlights that light behaves both as a wave and a particle under different conditions.
- 📊 Wien's Law explains how the wavelength of maximum energy emitted by a black body shifts towards shorter wavelengths as temperature increases.
- ✍️ Planck's oscillator and its average energy were derived, emphasizing the need to understand this for exams.
- ⚙️ The Schrödinger wave equation is crucial, and students should know both the time-dependent and time-independent versions.
- 💡 Compton effect and its impact on wavelength changes when a particle undergoes the effect were also covered.
- 🔑 Students are advised to memorize key laws and equations, particularly Planck's Radiation Law, which has been tested in exams.
- 📝 The lecture concluded with a review of Stefan's Law, the dual nature of light, and advice on solving numericals in quantum mechanics for exams.
Q & A
What are the five laws related to energy covered in the quantum mechanics unit of the AKTU Engineering Physics syllabus?
-The five laws related to energy in the quantum mechanics unit are Black Body Radiation, Stephen's Law, Wayne's Law, Rayleigh's Law, and Planck's Law.
What is the significance of the term 'black body' in the context of quantum mechanics?
-A black body is an idealized object that absorbs all incident radiation and emits no radiation. It is used as a model for understanding thermal radiation.
What is Stephen's Law and how is it expressed mathematically?
-Stephen's Law states that the total radiant energy emitted by a black body is proportional to the fourth power of its absolute temperature. Mathematically, it is expressed as E = σT^4, where E is the energy, σ is Stephen's constant (5.67 x 10^-8 W/m^2K^-4), and T is the temperature in Kelvin.
What does the graph of energy versus wavelength according to Stephen's Law show?
-The graph of energy versus wavelength according to Stephen's Law shows that at the peak value of energy, the wavelength is minimum, and as the energy drops, the wavelength increases.
What is the significance of Wien's Displacement Law in quantum mechanics?
-Wien's Displacement Law states that the product of the wavelength corresponding to maximum energy (λm) and the temperature (T) is a constant. This law helps in understanding how the peak of the spectral distribution curve shifts towards shorter wavelengths as the temperature increases.
What is the mathematical expression for Rayleigh-Jeans Law?
-Rayleigh-Jeans Law is given by Eλ = (8πkT) / (λ^4), where Eλ is the energy per unit wavelength, k is the Boltzmann constant, T is the temperature in Kelvin, and λ is the wavelength.
How is the average energy of a Planck's oscillator derived?
-The average energy of a Planck's oscillator is derived by considering the total energy of the oscillators and dividing it by the total number of oscillators. The formula is given by ⟨E⟩ = (hν / (e^(hν/kT) - 1)), where h is Planck's constant, ν is the frequency, k is the Boltzmann constant, and T is the temperature.
What is Planck's radiation formula and how is it used?
-Planck's radiation formula is given by u(ν)dν = (8πhν^2/c^3) dν / (e^(hν/kT) - 1), where u(ν) is the energy density per unit frequency, h is Planck's constant, ν is the frequency, c is the speed of light, and kT is the product of Boltzmann's constant and temperature. This formula is used to calculate the spectral radiance of a black body at a given temperature.
What is the Compton Effect and what is its significance in quantum mechanics?
-The Compton Effect is the increase in wavelength of X-rays when they scatter off electrons. It demonstrates the particle nature of light and is significant in quantum mechanics as it provides evidence for the quantization of energy and momentum.
How does the lecturer suggest students approach numerical problems in quantum physics?
-The lecturer suggests that students should approach numerical problems by showing each step of the solution, including formulating the problem, unit conversions, and applying the correct equations, to ensure they receive marks for each part of the solution.
Outlines
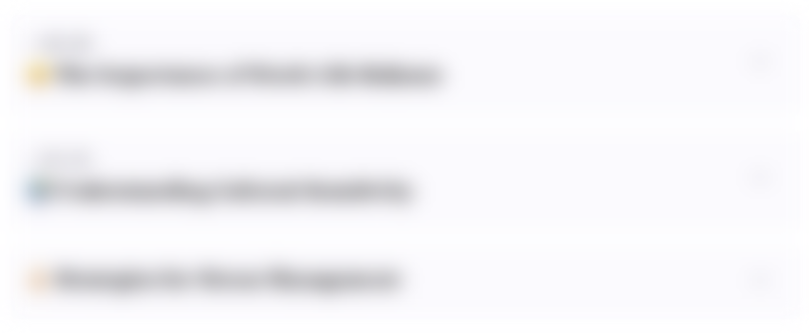
This section is available to paid users only. Please upgrade to access this part.
Upgrade NowMindmap
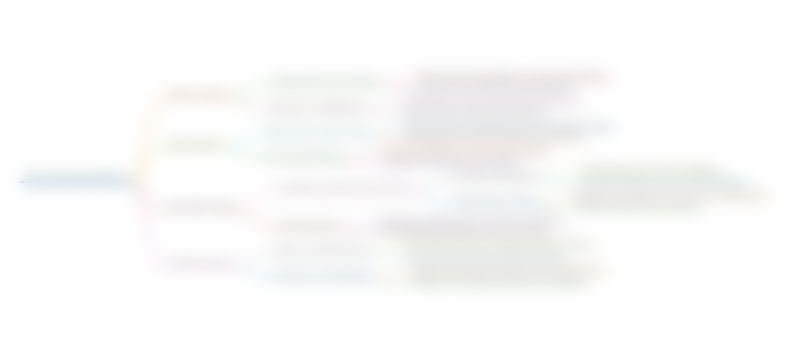
This section is available to paid users only. Please upgrade to access this part.
Upgrade NowKeywords
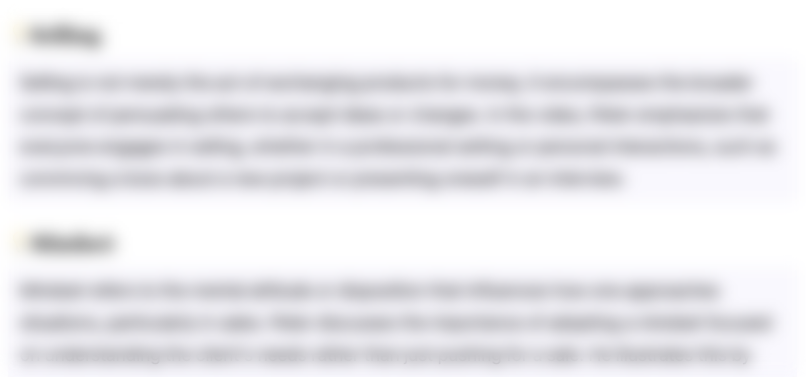
This section is available to paid users only. Please upgrade to access this part.
Upgrade NowHighlights
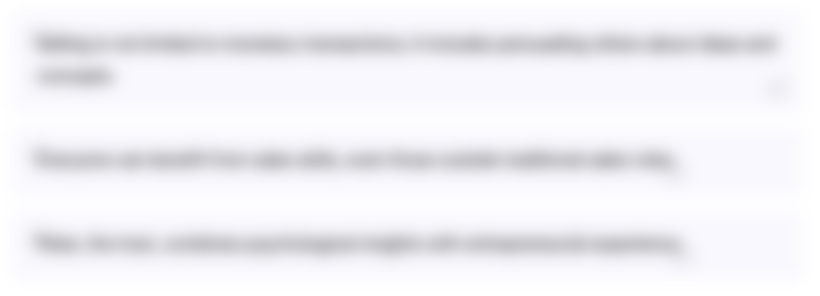
This section is available to paid users only. Please upgrade to access this part.
Upgrade NowTranscripts
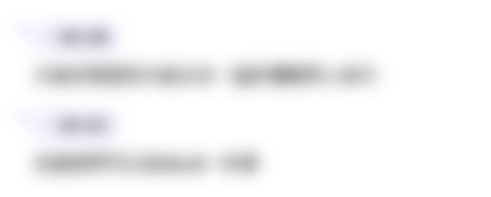
This section is available to paid users only. Please upgrade to access this part.
Upgrade NowBrowse More Related Video

QUANTUM PHYSICS | S-1 | ENGINEERING PHYSICS | FIRST YEAR ENGINEERING | SAURABH DAHIVADKAR
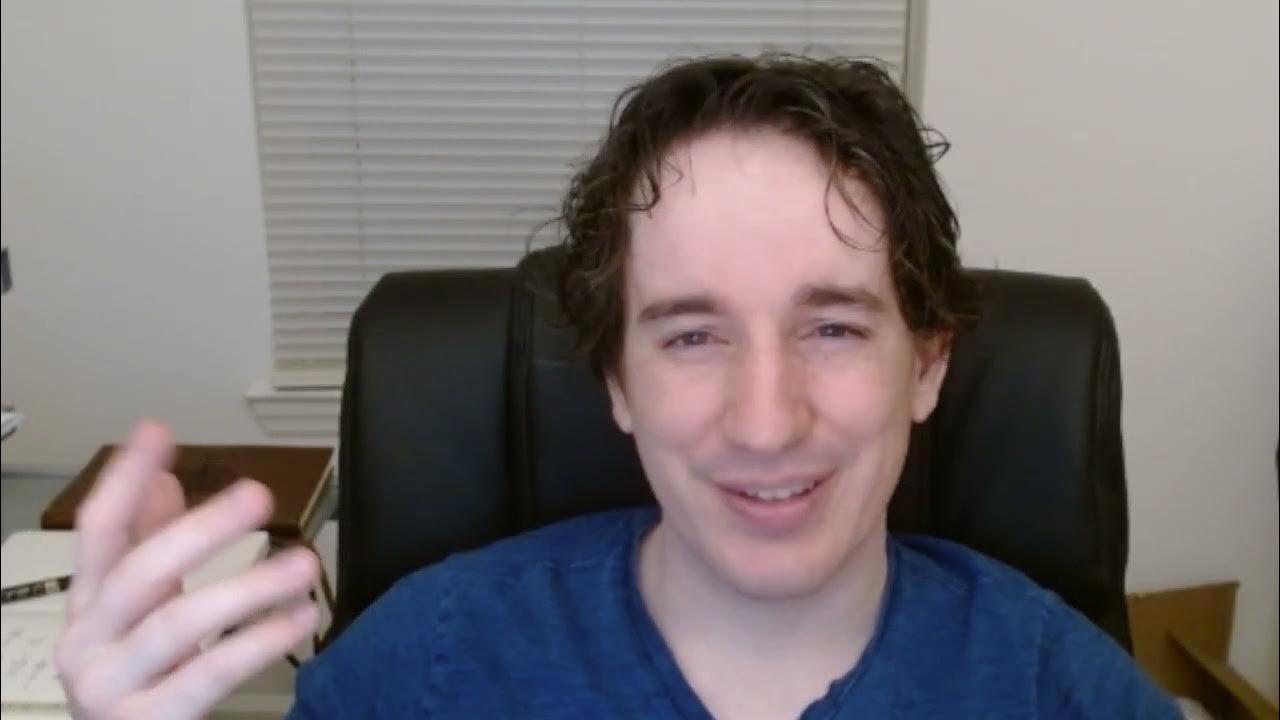
Physics GRE prep
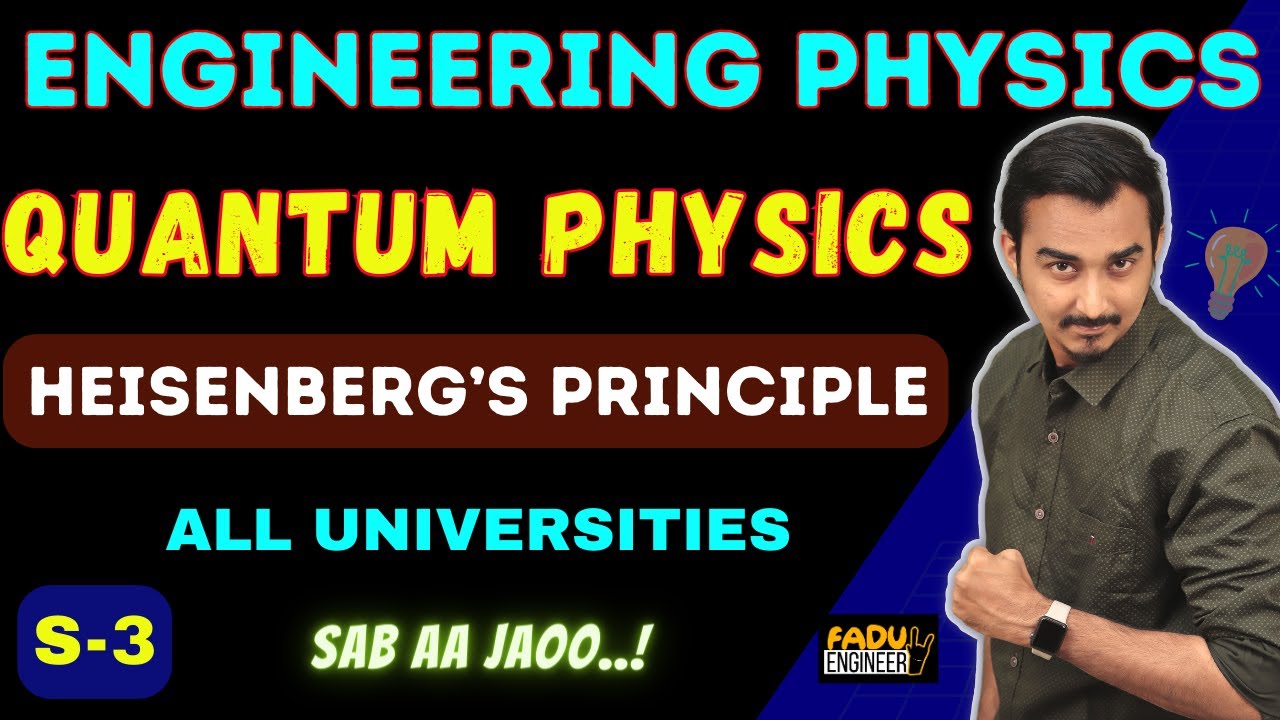
QUANTUM PHYSICS | S-3 | ENGINEERING PHYSICS | FIRST YEAR ENGINEERING | SAURABH DAHIVADKAR

QUANTUM PHYSICS | S-2 | ENGINEERING PHYSICS | FIRST YEAR ENGINEERING | SAURABH DAHIVADKAR

MODERN PHYSICS MIMP QUESTIONS FOR GTU EXAM || SEM 1 PHYSICS || UNIT 5 | SEMICONDUCTOR DEVICE #gtu
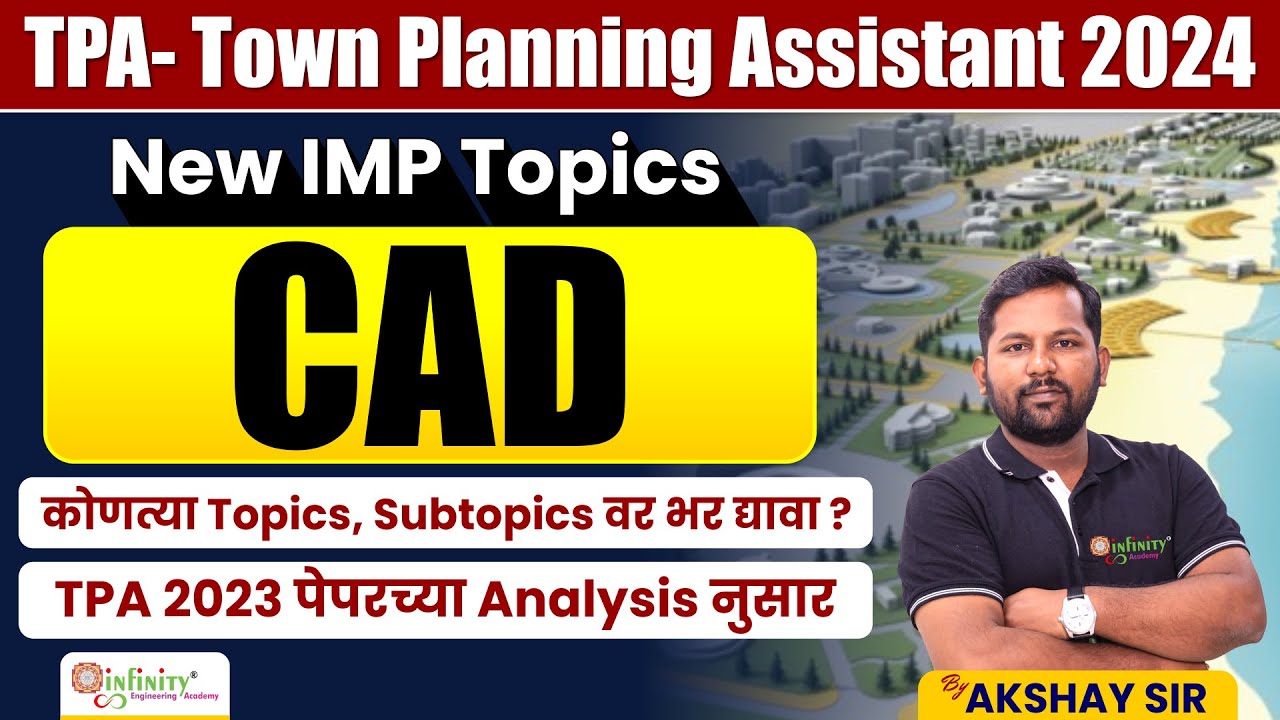
computer aided drawing | TPA New Topics | TPA Syllabus | TPA Recruitment | Town Planning Assistant
5.0 / 5 (0 votes)