DNA Chromo Replication 2c'
Summary
TLDRThis lecture delves into the intricate structure and replication of DNA, the genetic material. It explains the chromatin structure, highlighting the role of histone proteins in packaging DNA into a compact form. The lecture then transitions into DNA replication, discussing the semiconservative model supported by the Meselson-Stahl experiment. It further explores the process in prokaryotic cells, detailing the initiation at a specific origin, the role of helicases, topoisomerases, and primase in the replication machinery, and the bidirectional nature of the process.
Takeaways
- 🧬 Chromatin structure is composed of DNA and proteins, including histones that form a 'beads on a string' arrangement.
- 🌀 The 30 nm fiber is formed by the coiling of the DNA around histones, which can further organize into loops anchored to the nuclear matrix.
- 🔬 Electron micrographs illustrate the transition from the 30 nm fiber to loop domains, highlighting the role of scaffold proteins.
- 🧪 The Meselson-Stahl experiment provided evidence for semiconservative DNA replication, where each new DNA molecule consists of one old and one new strand.
- 🧵 DNA replication is not random; it initiates at specific origins of replication and proceeds bidirectionally.
- 🔄 Helicases play a crucial role in replication by unwinding the DNA double helix, creating template strands for new strand synthesis.
- 🌀 Topoisomerases relieve the strain caused by the supercoiling of DNA that occurs during replication.
- 🔬 Single-stranded binding proteins prevent reannealing of separated DNA strands and protect them from nucleases.
- 🧬 Primase is essential for replication as it synthesizes RNA primers to which DNA polymerases can add nucleotides, initiating new strand growth.
- 📚 The process of DNA replication is more complex in eukaryotic cells due to larger genomes and involves multiple origins of replication.
Q & A
What is the nucleosome structure described in the lecture?
-The nucleosome structure consists of an octomer of four histones forming a 'beads on a string' configuration, where DNA wraps around the histones 1 and 3/4 turns.
What are the two models that explain the further compaction of the 30 nm fiber into a more condensed chromatin structure?
-The two models are the solenoid model and the zigzag model, which help to form loops of DNA that are anchored to proteins called a scaffold or nuclear matrix.
What are scaffold attachment regions (SARS) and how do they relate to chromatin structure?
-Scaffold attachment regions (SARS), also known as matrix attachment regions (MARs), are the sites where the DNA loops are anchored to the scaffold proteins, contributing to the higher-order folding of chromatin.
How does the removal of histone proteins affect the appearance of DNA as seen under an electron microscope?
-Upon removal of histone proteins with high salt, the DNA appears as loose strands that are no longer organized into the structured chromatin fibers, indicating the role of histones in maintaining chromatin structure.
What is the significance of the Meselson-Stahl experiment in understanding DNA replication?
-The Meselson-Stahl experiment provided evidence supporting the semiconservative model of DNA replication, where each new DNA molecule consists of one original and one newly synthesized strand.
What are the differences between the three models of DNA replication: semiconservative, conservative, and dispersive?
-Semiconservative replication results in each new DNA molecule having one old and one new strand. Conservative replication would result in two old strands staying together and two new strands forming together. Dispersive replication would mix old and new segments in both new DNA molecules.
How does the use of nitrogen isotopes in the Meselson-Stahl experiment help to differentiate between replication models?
-The use of nitrogen-15 (heavy) and nitrogen-14 (light) isotopes allowed for the visualization of DNA strands based on their density after centrifugation, which helped to demonstrate that DNA replication is semiconservative.
What is the role of DNA helicase in the process of DNA replication?
-DNA helicase unwinds the DNA double helix into single strands by breaking hydrogen bonds between the bases, creating a replication bubble with two replication forks.
Why is topoisomerase necessary during DNA replication?
-Topoisomerase is necessary to relieve the strain caused by the supercoiling that occurs as the DNA helicase unwinds the DNA strands, preventing the replication fork from becoming too tight to continue replication.
What is the function of single-stranded binding proteins in DNA replication?
-Single-stranded binding proteins bind to the separated DNA strands during replication to prevent their reannealing and degradation, thus maintaining the integrity of the single-stranded DNA template.
Why can't DNA polymerase initiate DNA synthesis on its own, and what is the solution to this limitation?
-DNA polymerase cannot initiate DNA synthesis de novo because it requires a pre-existing strand to add nucleotides to. Primase solves this by synthesizing a short RNA primer that provides a starting point for DNA polymerase to begin DNA synthesis.
Outlines
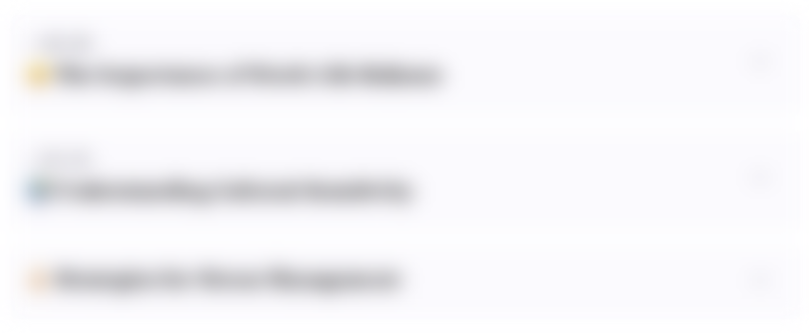
This section is available to paid users only. Please upgrade to access this part.
Upgrade NowMindmap
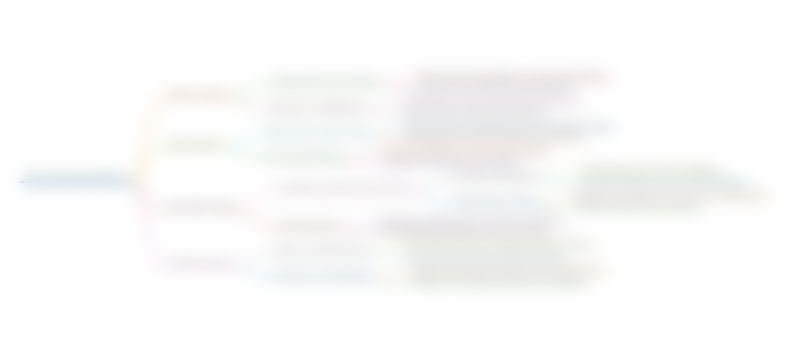
This section is available to paid users only. Please upgrade to access this part.
Upgrade NowKeywords
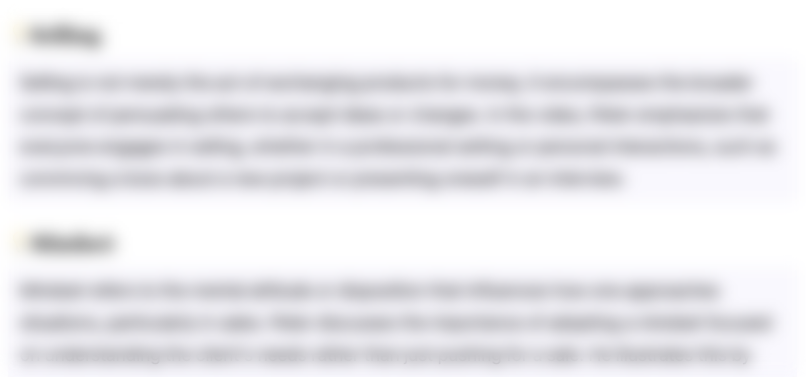
This section is available to paid users only. Please upgrade to access this part.
Upgrade NowHighlights
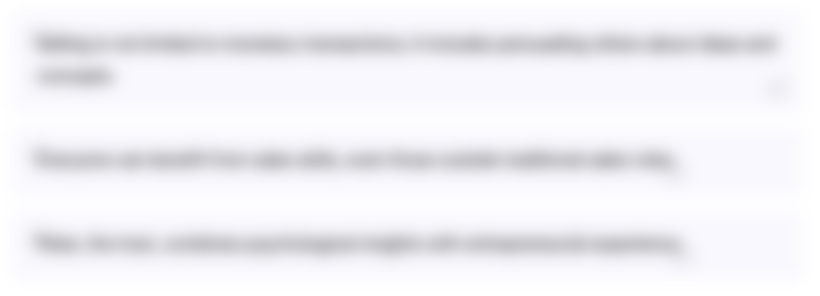
This section is available to paid users only. Please upgrade to access this part.
Upgrade NowTranscripts
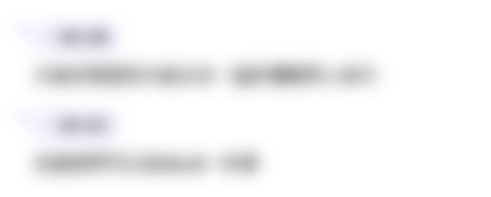
This section is available to paid users only. Please upgrade to access this part.
Upgrade NowBrowse More Related Video

DNA Replication
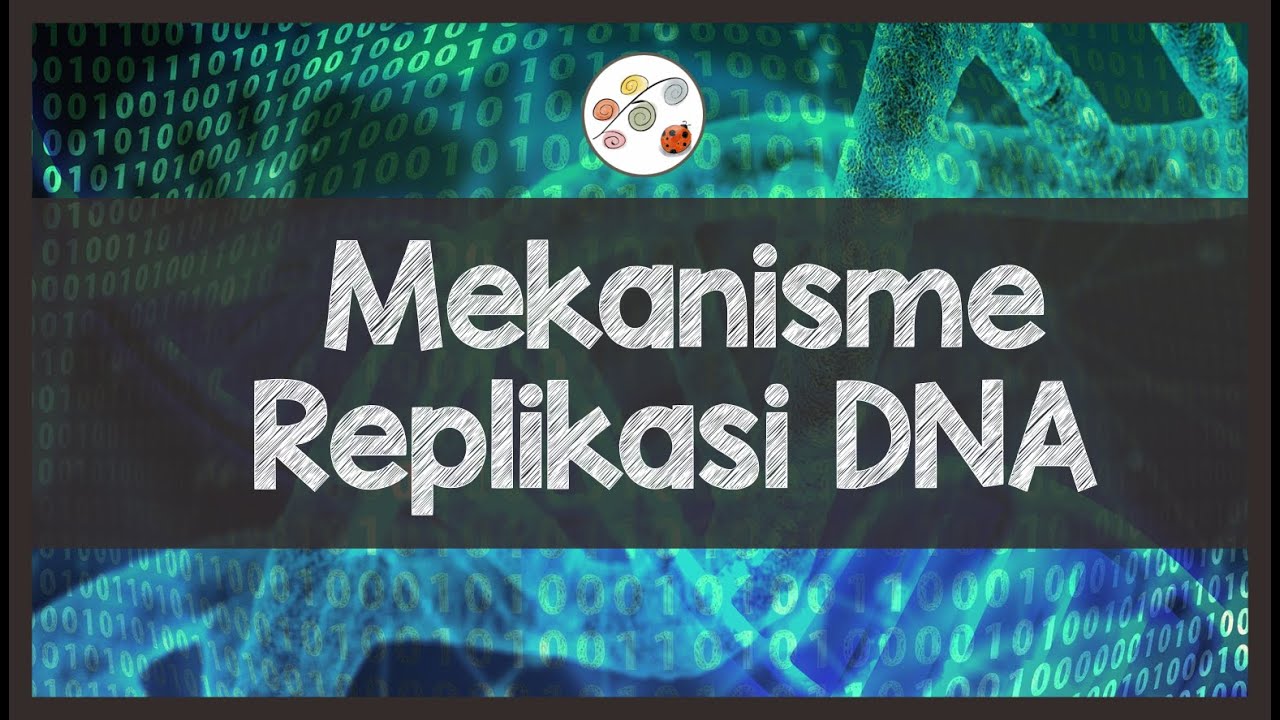
Proses Replikasi DNA - Proses Duplikasi atau Penggandaan DNA

Substansi genetika part 2 (Replikasi DNA dan Sintesis protein) - Biologi kelas 12 SMA
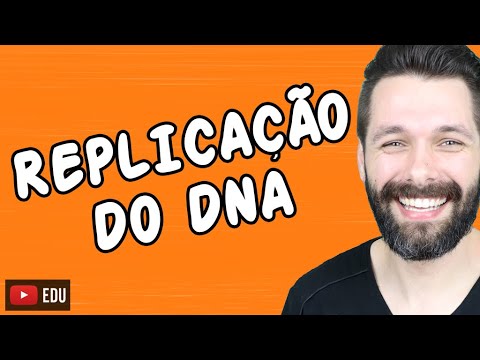
REPLICAÇÃO (DUPLICAÇÃO) DO DNA | Biologia com Samuel Cunha
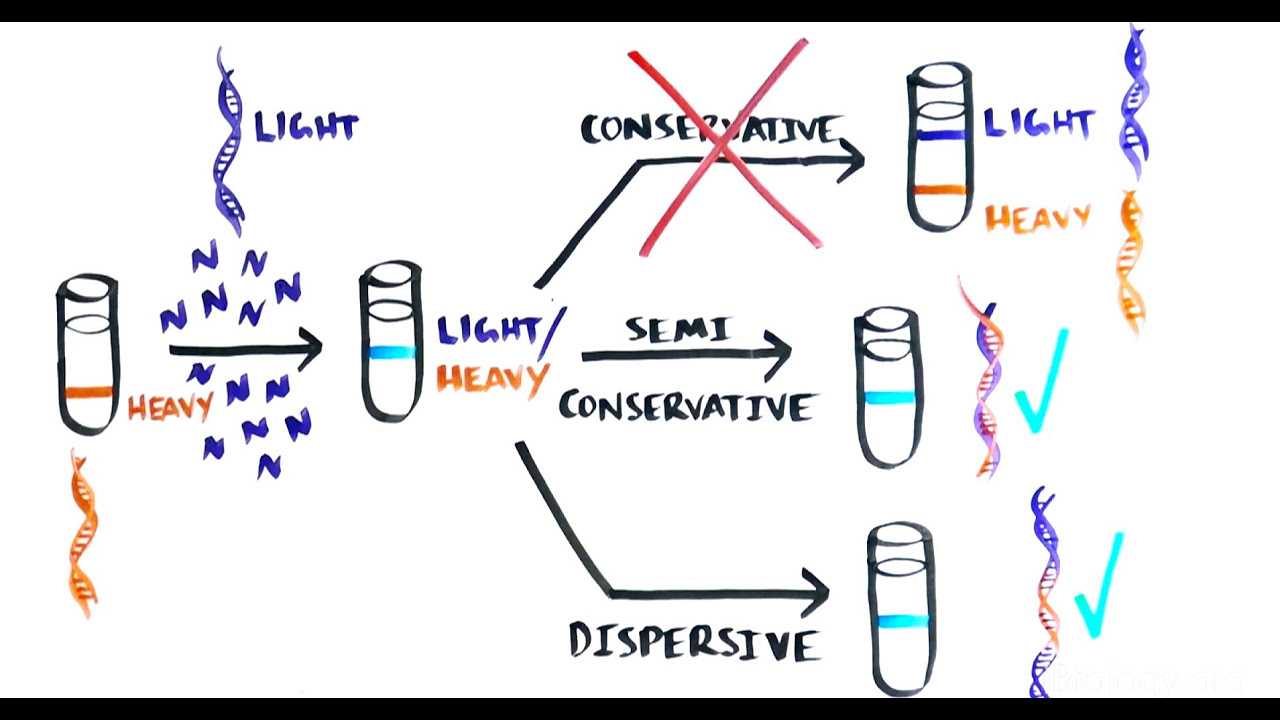
THE MOST BEAUTIFUL EXPERIMENT IN BIOLOGY: Meselson & Stahl, The Semi-Conservative Replication of DNA

DNA Replication In Eukaryotes | Initiation
5.0 / 5 (0 votes)