5.1 Forward and reverse biased PN junctions
Summary
TLDRThis lecture delves into the behavior of PN junctions under external bias, contrasting forward and reverse biases. It explains how forward bias reduces the barrier for carrier diffusion, allowing current flow, while reverse bias increases the barrier, halting diffusion and emphasizing drift current. The lecture also introduces the concept of energy band diagrams, illustrating how applied voltage shifts Fermi levels and alters the depletion region's width. The instructor emphasizes the importance of understanding these principles for grasping semiconductor device operation, with a promise to explore quantitative aspects and minority carrier behavior in upcoming sessions.
Takeaways
- 🔬 The lecture discusses the behavior of PN junctions under different bias conditions, focusing on the changes in the depletion layer and electric field.
- 🔋 When no external voltage is applied, the PN junction is in equilibrium with a uniform Fermi level across it, and there is no current flow due to the potential barrier.
- ⚡ In forward bias, a positive voltage is applied to the P-type semiconductor, which reduces the potential barrier and allows majority carriers to diffuse into the depletion region, leading to a decrease in space charge density and depletion width.
- 🔌 Under reverse bias, a negative voltage is applied to the P-type semiconductor, increasing the potential barrier and causing majority carriers to move out of the PN junction, increasing the space charge density and depletion width.
- 📉 The energy band diagram of a PN junction changes with the application of voltage, with the Fermi level shifting down in forward bias and up in reverse bias.
- 🔑 The lecture emphasizes the importance of understanding the energy band diagrams to grasp the fundamental physics of how PN junctions operate.
- 🚫 In forward bias, the diffusion of majority carriers is dominant, and the drift current of minority carriers can be considered negligible.
- 📈 In reverse bias, the drift current becomes significant because the increased potential barrier prevents significant carrier diffusion.
- 📚 The lecture provides a recipe for drawing energy band diagrams under different biases, which is crucial for understanding the behavior of PN junctions.
- 🔮 Upcoming lectures will delve into the quantitative aspects of PN junctions, including the analysis of minority carrier distributions and their impact on current flow.
Q & A
What is a PN junction and what happens when an external bias is applied?
-A PN junction is a semiconductor device formed by joining P-type and N-type semiconductors. When an external bias is applied, it affects the potential barrier, allowing for the movement of majority carriers into the depletion region under forward bias, or out of the junction under reverse bias.
How does the depletion layer change when a forward bias is applied to a PN junction?
-Under forward bias, the depletion layer width decreases because the external voltage reduces the potential barrier, allowing majority carriers to diffuse into the depletion region, leading to a reduction in space charge density.
What is the effect of reverse bias on the depletion region of a PN junction?
-Reverse bias increases the depletion region width and the potential barrier. This causes majority carriers to move out of the PN junction, increasing the space charge density and leaving behind uncompensated ionized dopants.
What is the built-in potential (qVbi) and how does it relate to the Fermi level?
-The built-in potential (qVbi) represents the potential barrier at the PN junction when no external bias is applied. It is related to the Fermi level, which is uniform across the junction in equilibrium, and is defined as the built-in voltage times the charge, giving the potential barrier in electron volts.
Why is there no current flow in a PN junction without an external voltage?
-Without an external voltage, the potential barrier prevents the diffusion of majority carriers across the junction. Electrons cannot move from the N-type to the P-type, and holes cannot move from the P-type to the N-type, resulting in no current flow.
How does the Fermi level shift when a positive voltage is applied to a semiconductor?
-When a positive voltage is applied to a semiconductor, the Fermi level shifts lower because it is analogous to removing electrons from the semiconductor, similar to lowering the water level in a tank.
What is the significance of the energy band diagram in understanding the operation of a PN junction?
-The energy band diagram is crucial for visualizing the changes in the semiconductor's energy levels under different biases. It helps to understand how the applied voltage alters the potential barrier, allowing or preventing the diffusion of carriers.
What are the two types of current in semiconductors and how do they relate to the operation of a PN junction?
-The two types of current in semiconductors are diffusion current, which occurs due to a gradient in carrier concentration, and drift current, which is caused by an electric field. In a PN junction, diffusion current is significant in forward bias, while drift current becomes important in reverse bias.
Why can the drift current of minority carriers be neglected in forward bias but not in reverse bias?
-In forward bias, the majority carrier diffusion is dominant due to the reduced potential barrier, making the drift current of minority carriers negligible. In reverse bias, the potential barrier is increased, preventing majority carrier diffusion, thus making the drift current of minority carriers significant.
What will be discussed in the next video regarding the quantitative aspects of PN junctions?
-The next video will delve into the quantitative aspects of PN junctions, including the study of how minority carrier distribution changes under different biases and the derivation of expressions for these distributions.
Outlines
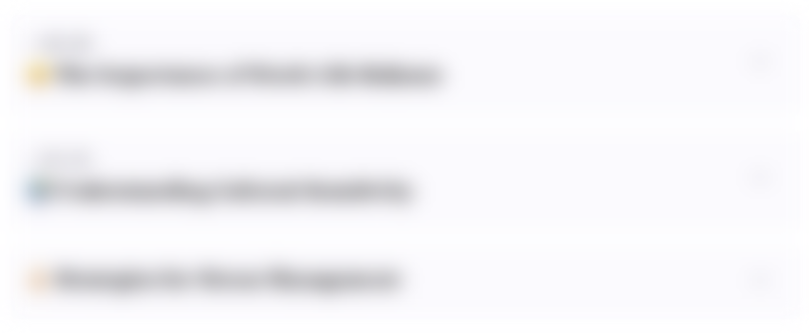
This section is available to paid users only. Please upgrade to access this part.
Upgrade NowMindmap
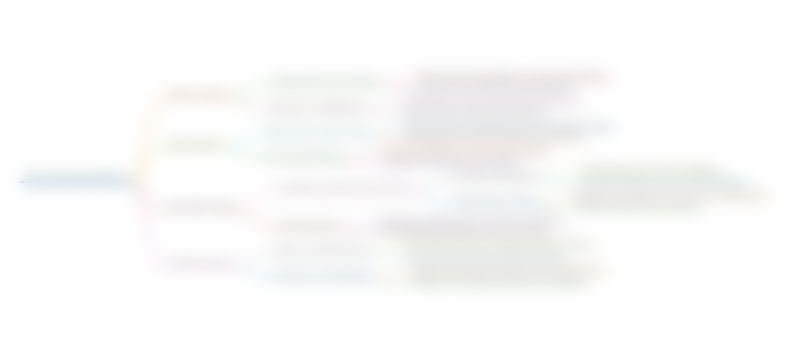
This section is available to paid users only. Please upgrade to access this part.
Upgrade NowKeywords
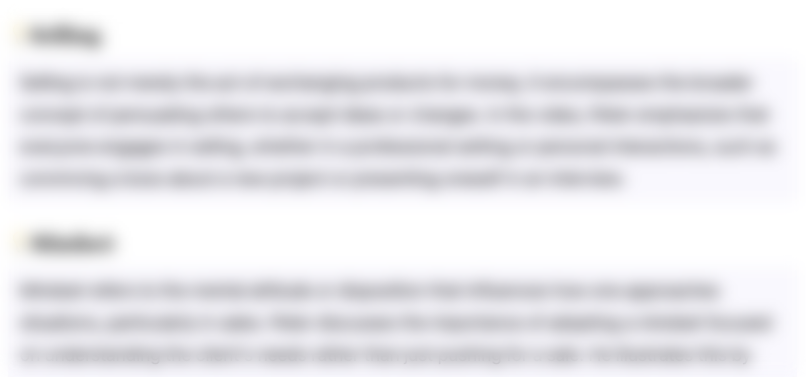
This section is available to paid users only. Please upgrade to access this part.
Upgrade NowHighlights
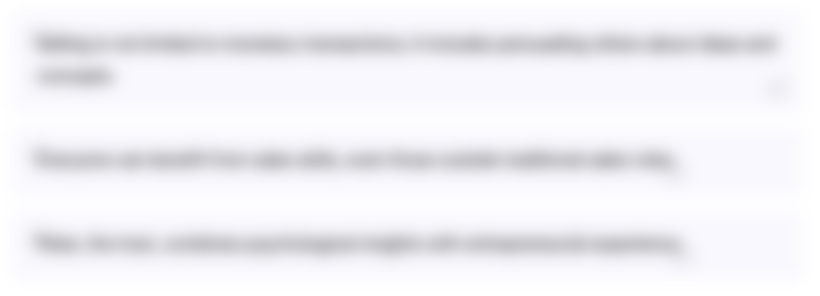
This section is available to paid users only. Please upgrade to access this part.
Upgrade NowTranscripts
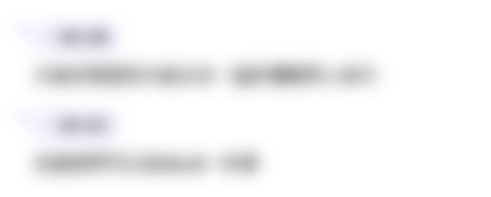
This section is available to paid users only. Please upgrade to access this part.
Upgrade NowBrowse More Related Video
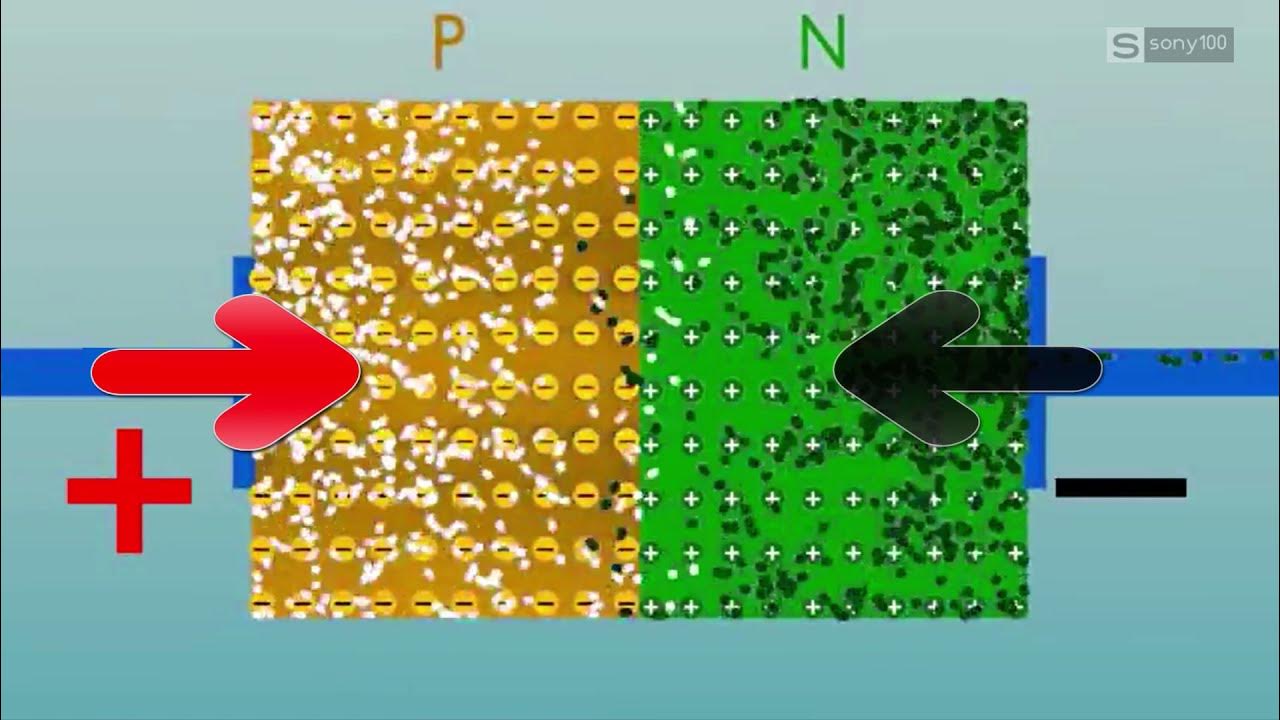
Hoạt động của tiếp xúc P-N | Chất bán dẫn là gì?

PN junction Diode Explained | Forward Bias and Reverse Bias
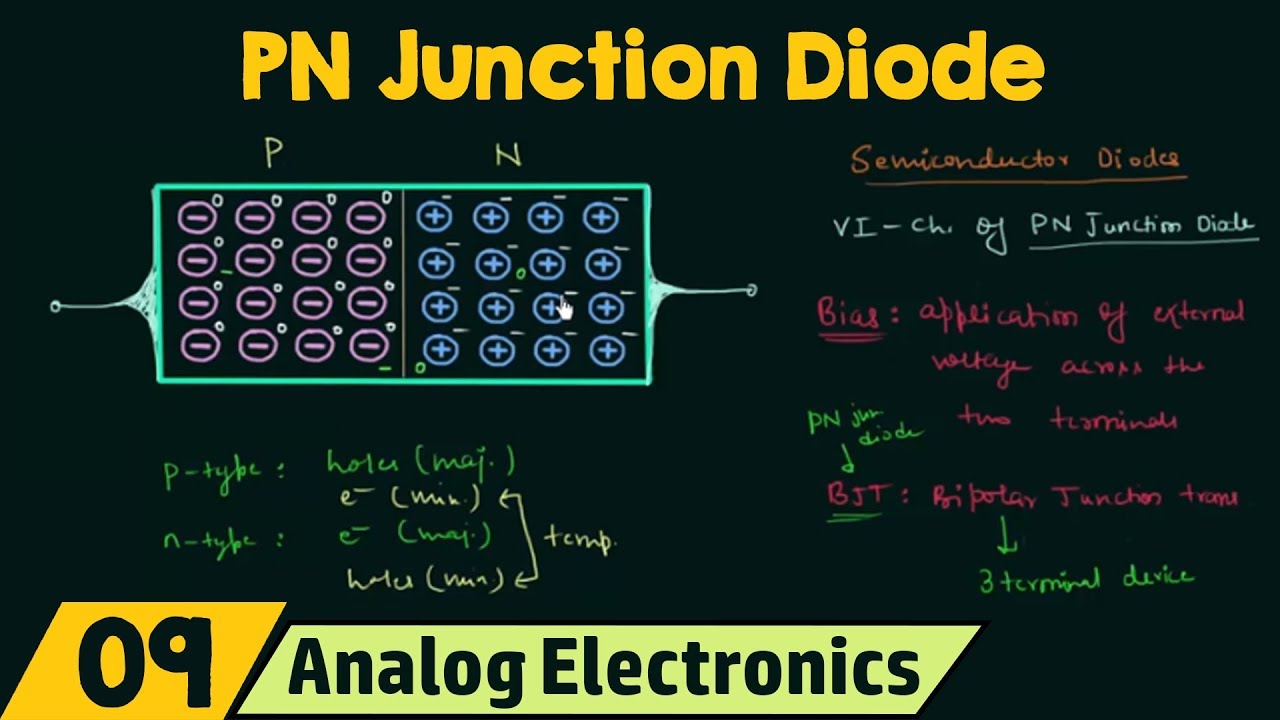
PN Junction Diode (No Applied Bias)

U1_L2_P-N Junction Diode | Electronics Engineering (BEC101/201)| Hindi

Cara Kerja Dioda PN Junction | Kuliah Fisika Semikonduktor
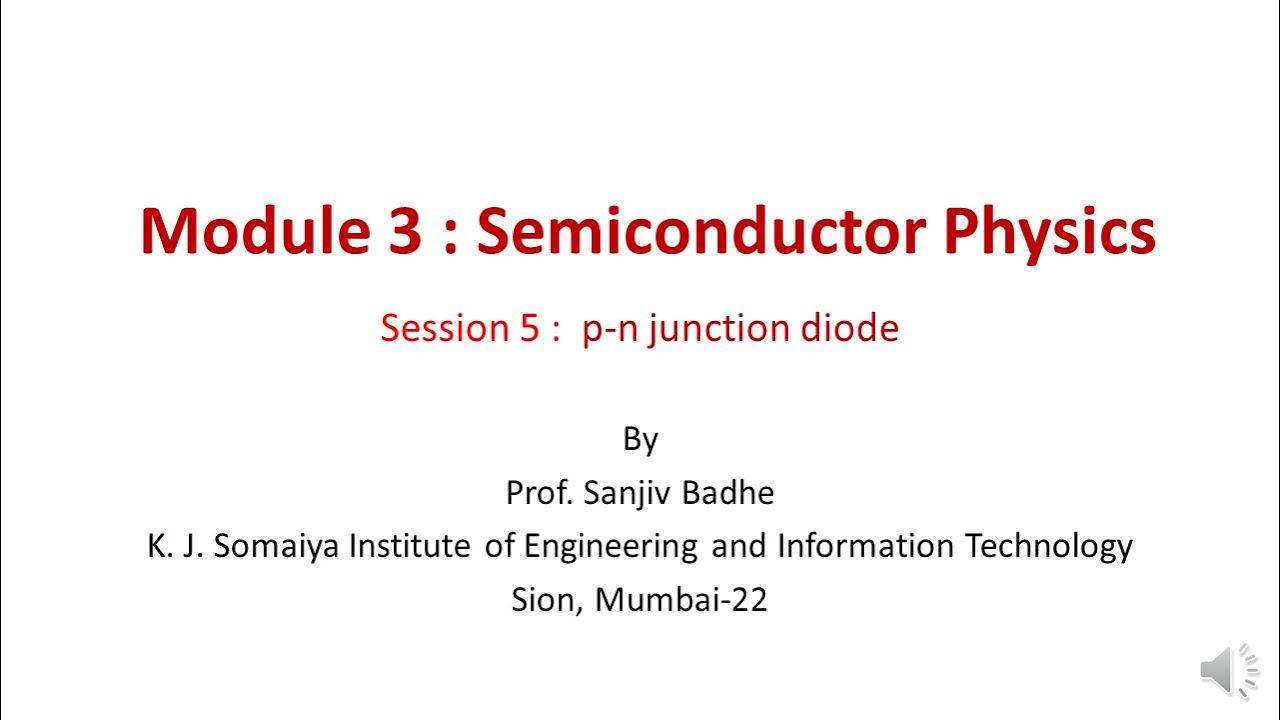
Semiconductor Physics Session 5 (p-n junction diode)
5.0 / 5 (0 votes)