04 Atomic Definition of Young's Modulus
Summary
TLDR本视频讲解了弹性行为和弹性变形的概念。通过弹性带的例子,说明了材料在弹性区域内加载后卸载能够恢复原状的特性。视频中探讨了原子层面的解释,将原子模拟为硬球体并通过弹簧连接,展示了原子间的净力与原子间距的关系。强调了杨氏模量与原子间力-间距曲线在平衡间距处的一阶导数成正比,表明杨氏模量仅取决于原子类型,与材料结构无关,是材料固有的属性。
Takeaways
- 🔍 弹性行为指的是材料在外力作用下发生形变,移除外力后能够恢复到原始形状的特性。
- 📏 胡克定律描述了材料在弹性范围内,应力与应变之间的线性关系。
- 🔵 弹性带是一个展示弹性行为的直观例子,它在被拉伸后能够恢复到原始形状。
- 🔬 弹性变形可以被理解为材料在卸载后能够恢复到原始几何形状的现象。
- 🧠 弹性应变也被称为可恢复应变,因为材料在弹性区域内卸载后应变会恢复到零。
- 🌐 原子层面上,弹性行为意味着当外力移除后,原子能够回到它们原始的位置。
- 🎾 通过将原子模拟为硬球体,并用弹簧连接,可以建立一个机械模型来模拟原子间的相互作用。
- 📉 原子间的净力可以通过绘制原子间力与原子间距的关系曲线来表示,其中包括吸引力和排斥力。
- 🔄 当原子处于平衡状态时,净力为零,此时的原子间距被称为平衡原子间距。
- 🔗 杨氏模量与原子间力-间距曲线在平衡原子间距处的一阶导数成正比,表明它仅取决于原子类型,与材料的结构无关。
Q & A
什么是弹性行为?
-弹性行为指的是材料在外力作用下发生形变,当外力移除后,材料能够恢复到原始几何形状的特性。
胡克定律是什么?
-胡克定律是描述材料在弹性范围内,应力与应变成正比关系的定律。
弹性变形和弹性区域在材料科学中意味着什么?
-弹性变形是指材料在卸载后能够恢复到原始几何形状的变形。弹性区域是指材料在受到应力作用时,应力与应变呈线性关系,且卸载后能够完全恢复的区域。
什么是可恢复应变?
-可恢复应变,也称为弹性应变,是指材料在卸载后能够恢复到原始形状的应变,通常与弹性行为相关联。
原子层面上,弹性变形意味着什么?
-在原子层面上,弹性变形意味着当外力移除后,原子能够回到它们原始的位置。
如何用机械模型来模拟原子间的相互作用?
-可以通过将原子视为硬球体,并在它们之间放置一个代表原子间净力的弹簧来模拟原子间的相互作用。
什么是原子间的平衡距离?
-原子间的平衡距离是指在没有外力作用下,原子之间自然保持的距离,也称为平衡原子间距。
原子间的净力与原子间距有什么关系?
-原子间的净力与原子间距的关系可以通过一个曲线来描述,其中净力是吸引力和排斥力的总和,且在平衡间距附近近似为线性关系。
杨氏模量与原子间力-分离曲线有什么关系?
-杨氏模量与原子间力-分离曲线的关系是直接成比例的,具体来说,杨氏模量是该曲线在平衡原子间距处的一阶导数。
为什么说杨氏模量是结构独立的?
-杨氏模量是结构独立的,因为它只取决于材料中原子的类型,而与材料的宏观结构、化学组成或微观结构无关。
材料的弹性行为如何影响其杨氏模量?
-材料的弹性行为直接影响其杨氏模量,因为杨氏模量是描述材料在弹性范围内应力与应变关系的物理量。
Outlines
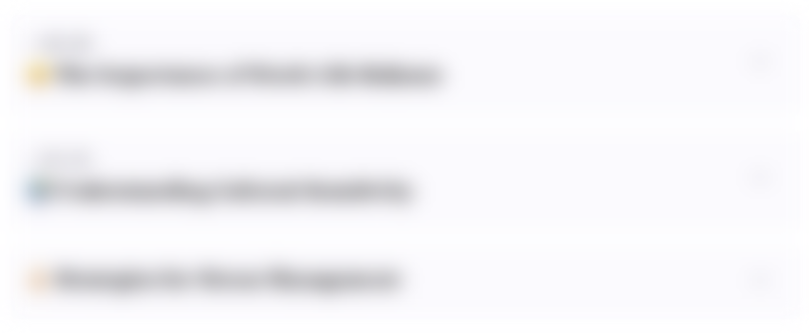
Этот раздел доступен только подписчикам платных тарифов. Пожалуйста, перейдите на платный тариф для доступа.
Перейти на платный тарифMindmap
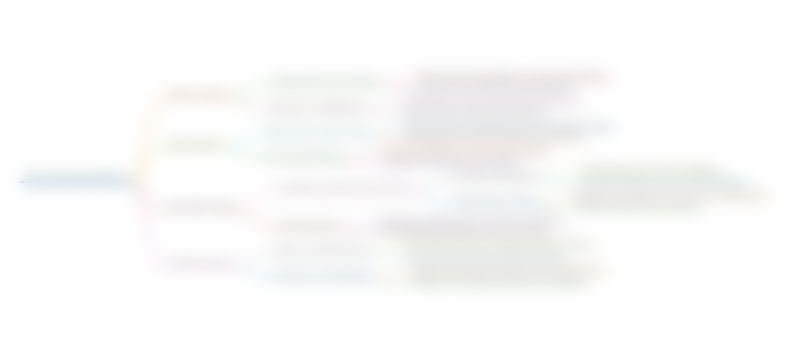
Этот раздел доступен только подписчикам платных тарифов. Пожалуйста, перейдите на платный тариф для доступа.
Перейти на платный тарифKeywords
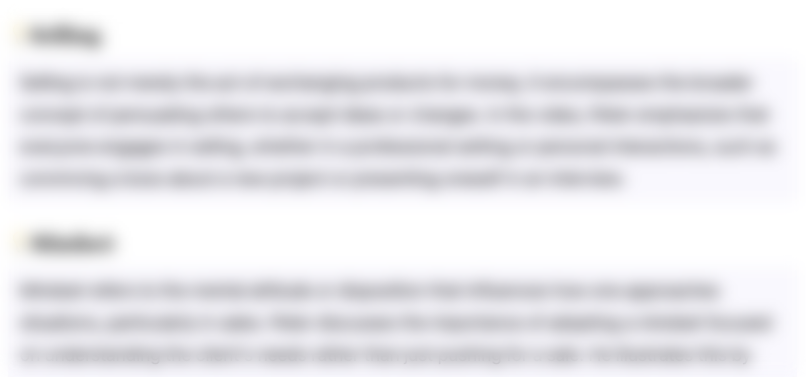
Этот раздел доступен только подписчикам платных тарифов. Пожалуйста, перейдите на платный тариф для доступа.
Перейти на платный тарифHighlights
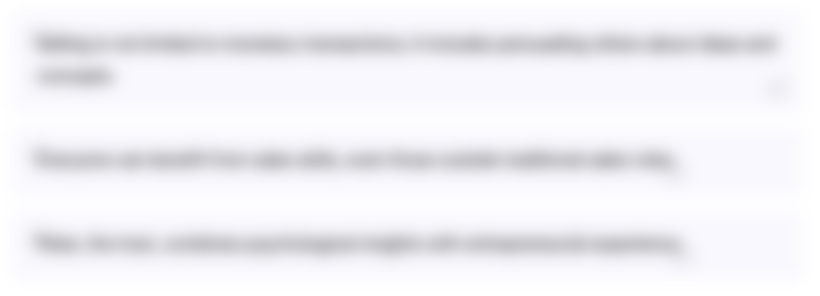
Этот раздел доступен только подписчикам платных тарифов. Пожалуйста, перейдите на платный тариф для доступа.
Перейти на платный тарифTranscripts
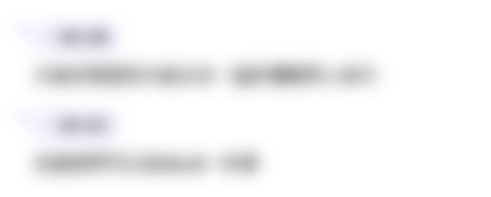
Этот раздел доступен только подписчикам платных тарифов. Пожалуйста, перейдите на платный тариф для доступа.
Перейти на платный тарифПосмотреть больше похожих видео

CCIR - Quantum Physics: Information, Foundations And Gravity - Lecture Session #2
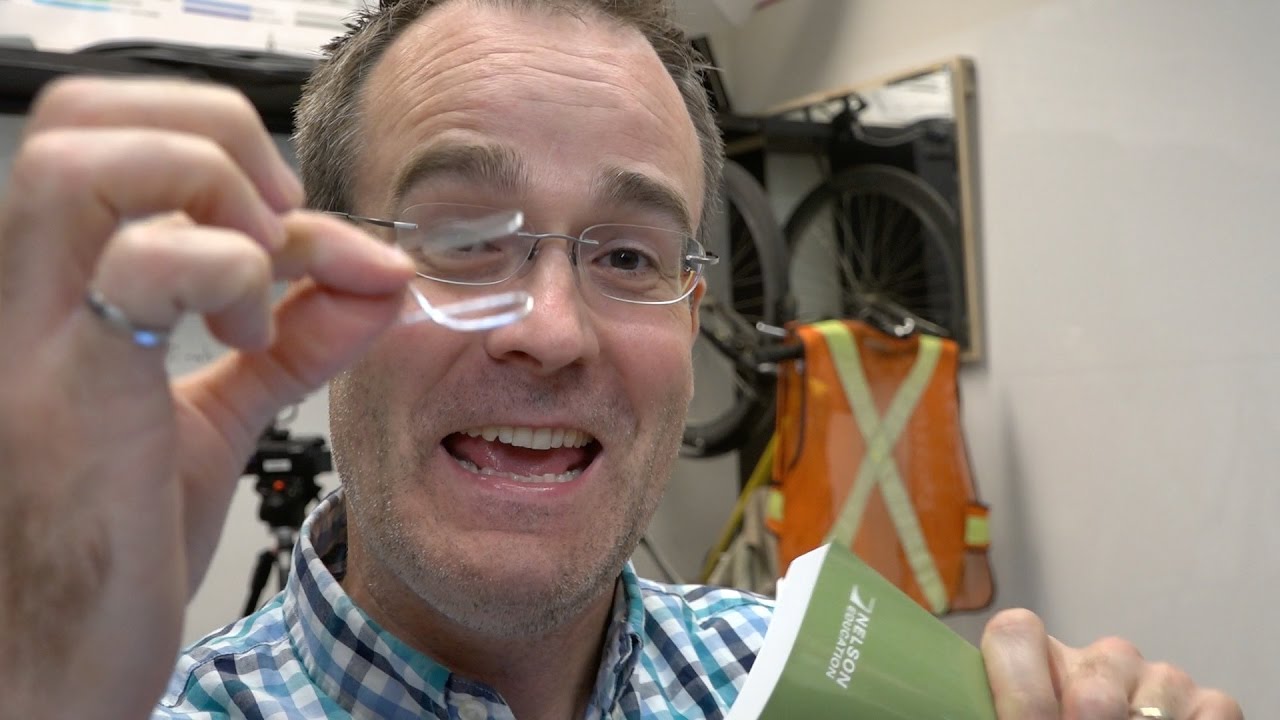
07 Introduction to Permanent Deformation
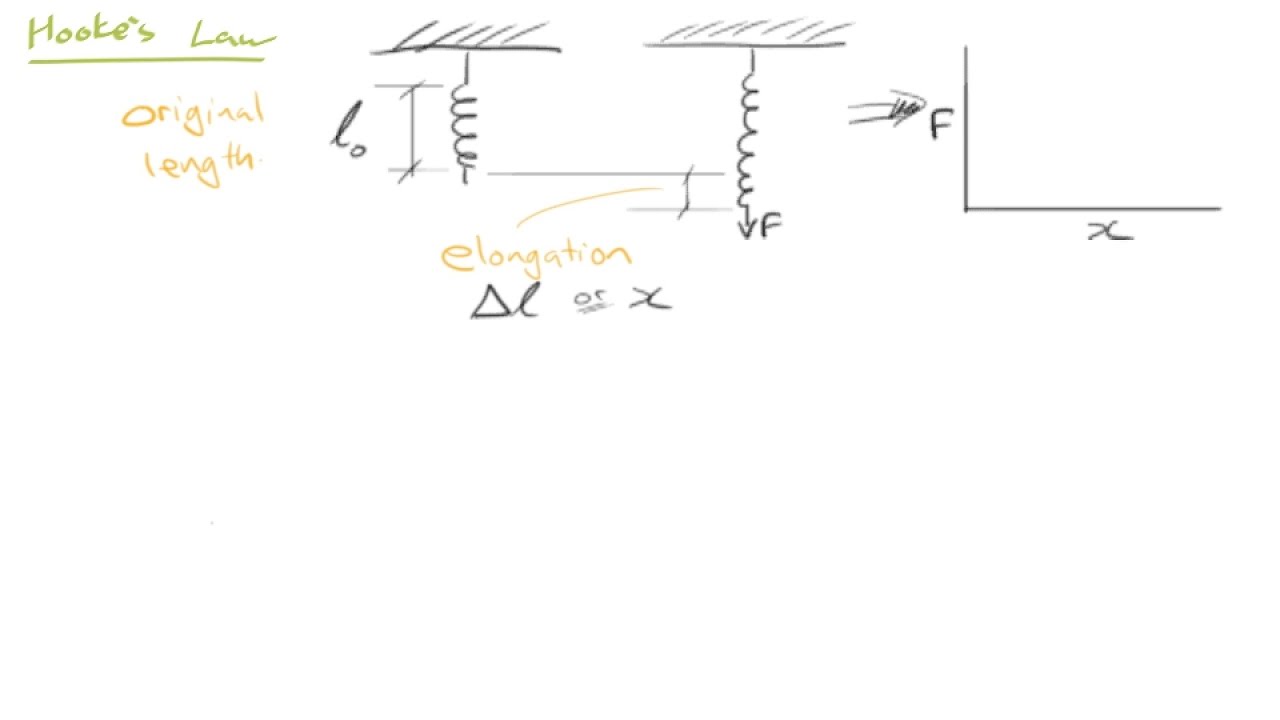
02 Hooke's Law

Physics of TIME and the SUPERNATURAL [PART 2]

What does the second derivative actually do in math and physics?
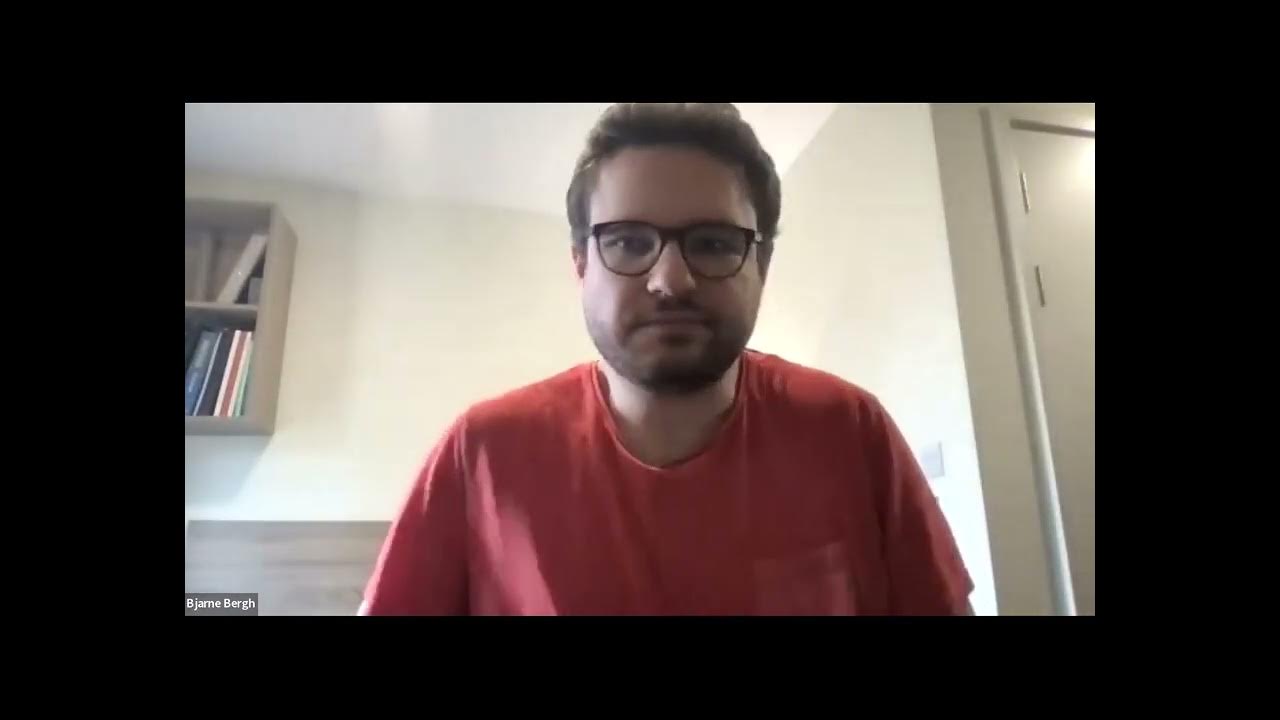
CCIR - Quantum Physics: Information, Foundations And Gravity - TA Session #1
5.0 / 5 (0 votes)