Steel Metallurgy - Principles of Metallurgy
Summary
TLDRThis video script delves into the composition and properties of steel, highlighting the role of alloying elements like carbon, manganese, and chromium in affecting strength, hardness, and other characteristics. It also discusses the significance of the Iron Carbon Equilibrium Diagram, Continuous Cooling Transformation, and Time Temperature Transformation diagrams in understanding steel's behavior during solidification and heat treatment.
Takeaways
- 🔨 Steel is primarily composed of iron with up to 1% carbon and other alloying elements, which can make up to 50% in some steels.
- 🔧 Alloying additions in steel, such as carbon, silicon, manganese, and others, significantly affect properties like strength, hardness, toughness, ductility, and more.
- 🌡️ The Iron Carbon Equilibrium Diagram is crucial for understanding steel structures at different temperatures and carbon contents, influencing the behavior of complex steel alloys.
- 🌡️ The A1 and A3 temperatures are key points in steel transformation, marking the transition from austenite to pearlite and from ferrite to austenite, respectively.
- 🧪 Alloying elements like manganese, chromium, and molybdenum enhance hardenability, allowing for deeper hardening throughout the steel's thickness.
- 🌡️ The Continuous Cooling Transformation (CCT) and Time Temperature Transformation (TTT) diagrams are essential tools in metallurgy for determining steel structures at various cooling rates.
- 🔬 The structure of steel can vary from pearlite with ferrite or cementite (soft), to martensite (hard), to bainite (intermediate), depending on the cooling process.
- 🚀 Carbon is a key element in steel, affecting both strength and hardness, and is essential for the formation of microstructures.
- 🔍 The hardenability of steel is distinct from hardness, with hardenability describing the depth of hardening achievable in the steel, influenced by alloying elements.
- 🛠️ Strengthening mechanisms in steel, such as grain size reduction, cold working, solid solution strengthening, and dispersion or precipitation strengthening, work to impede dislocation movement, thereby increasing strength.
Q & A
What is steel primarily composed of?
-Steel is primarily composed of iron with up to 1% carbon, plus other alloying additions which generally total less than 5%, but can be as great as 50% in some steels.
How do alloying additions affect the properties of steel?
-Alloying additions can affect various properties of steel such as strength, hardness, toughness, ductility, fatigue, formability, machinability, weldability, and corrosion resistance, each in a different way depending on the type and amount added.
What is the role of carbon in steel?
-Carbon is the most important element for strength and hardness in steel. As the level of carbon increases, the tensile strength and hardness also increase. It is essential for the formation of microstructures.
Why is phosphorus usually considered an impurity in steel?
-Phosphorus is considered an impurity because it significantly reduces the toughness and ductility of steel. However, it can be used as a solid solution strengthener.
What is the impact of sulphur on steel properties?
-Sulphur is generally an impurity that reduces the ductility, toughness, and weldability of steel. It can form iron sulphide, which can cause the steel to break up during hot working, a phenomenon known as hot shortness.
How does manganese affect steel?
-Manganese has a great effect on hardenability and can strengthen steel through solid solution strengthening. It also combines with sulphur to prevent hot shortness.
What is the significance of chromium in steel?
-Chromium increases the hardenability of steel and forms very stable carbides with carbon, which are excellent for wear and abrasion resistance. It is used in high levels in stainless steels for corrosion resistance by creating a protective oxide film on the surface.
Why is molybdenum added to steel?
-Molybdenum increases the hardenability of steels. When combined with chromium and nickel, it has a strong multiplicative effect on hardenability and is used in both alloy and stainless steels.
What is the role of nickel in steel?
-Nickel increases the hardenability of steel and can increase the toughness of steels, particularly at low temperatures. It is also present in large amounts in stainless steels.
How does the Iron Carbon Equilibrium Diagram help in understanding steel?
-The Iron Carbon Equilibrium Diagram is used to understand what structures will be formed at what temperatures and carbon contents, and to calculate how much liquid and solid will be present at a given temperature. It helps in understanding the behavior of complex steel alloys.
What are the differences between the Continuous Cooling Transformation (CCT) diagram and the Time Temperature Transformation (TTT) diagram?
-The CCT diagram shows the structures achievable by continuously cooling from the austenitization temperature at a constant rate, while the TTT diagram shows how long it would take for a structure to be achieved by holding at a given temperature. Both diagrams help in selecting the optimum steel and process parameters for achieving a given set of properties.
How does hardenability relate to the depth of hardness achieved in steel?
-Hardenability describes how deep into the steel hardening can be achieved. A steel with high hardenability will have the same hardness throughout the thickness of the product, which is important for large components.
What are the different strengthening mechanisms in steel?
-Strengthening mechanisms in steel include reducing grain size, cold working, solid solution strengthening, and dispersion or precipitation strengthening. These mechanisms work by reducing the ability of dislocations to move through the steel.
Outlines
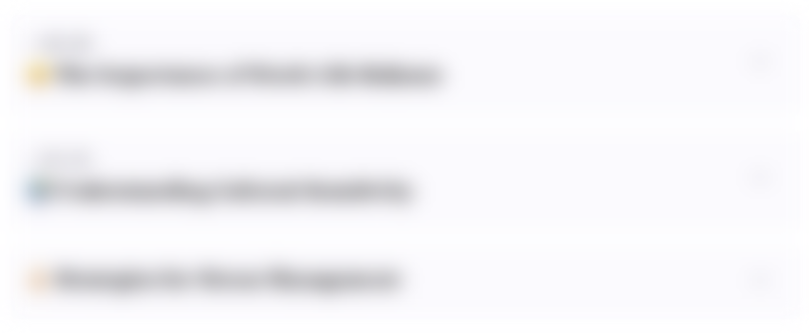
このセクションは有料ユーザー限定です。 アクセスするには、アップグレードをお願いします。
今すぐアップグレードMindmap
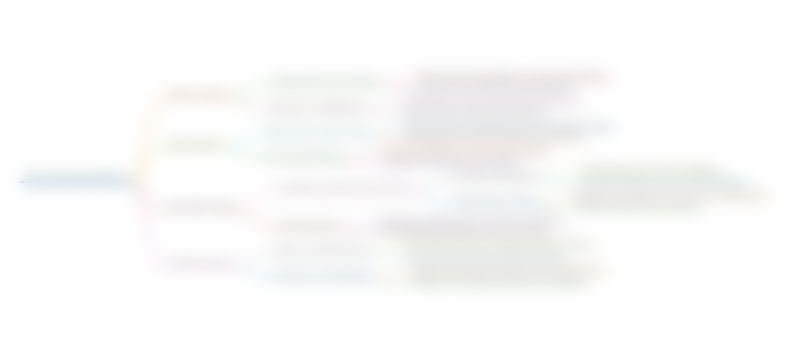
このセクションは有料ユーザー限定です。 アクセスするには、アップグレードをお願いします。
今すぐアップグレードKeywords
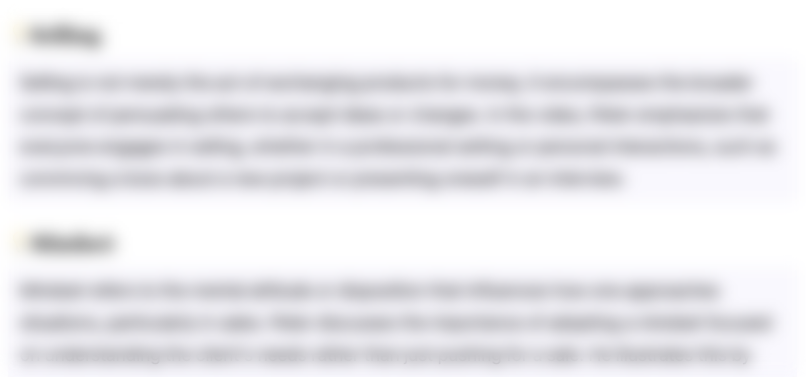
このセクションは有料ユーザー限定です。 アクセスするには、アップグレードをお願いします。
今すぐアップグレードHighlights
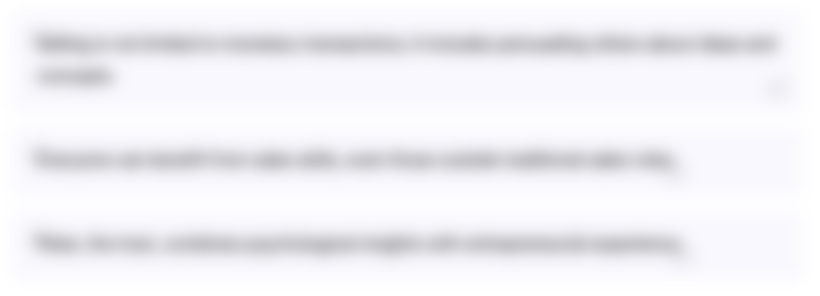
このセクションは有料ユーザー限定です。 アクセスするには、アップグレードをお願いします。
今すぐアップグレードTranscripts
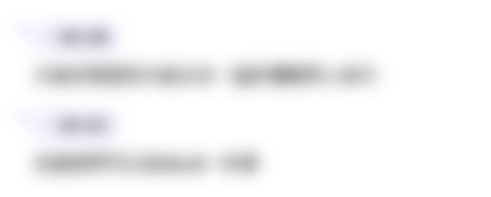
このセクションは有料ユーザー限定です。 アクセスするには、アップグレードをお願いします。
今すぐアップグレード関連動画をさらに表示
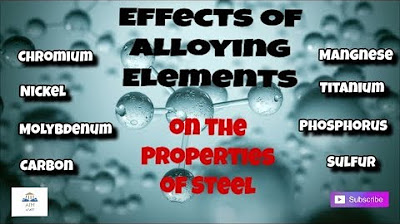
Effects of alloying Elements on the Properties of steel. ||Engineer's Academy||

Material Science, The Iron Carbon Phase Diagram, Part 1
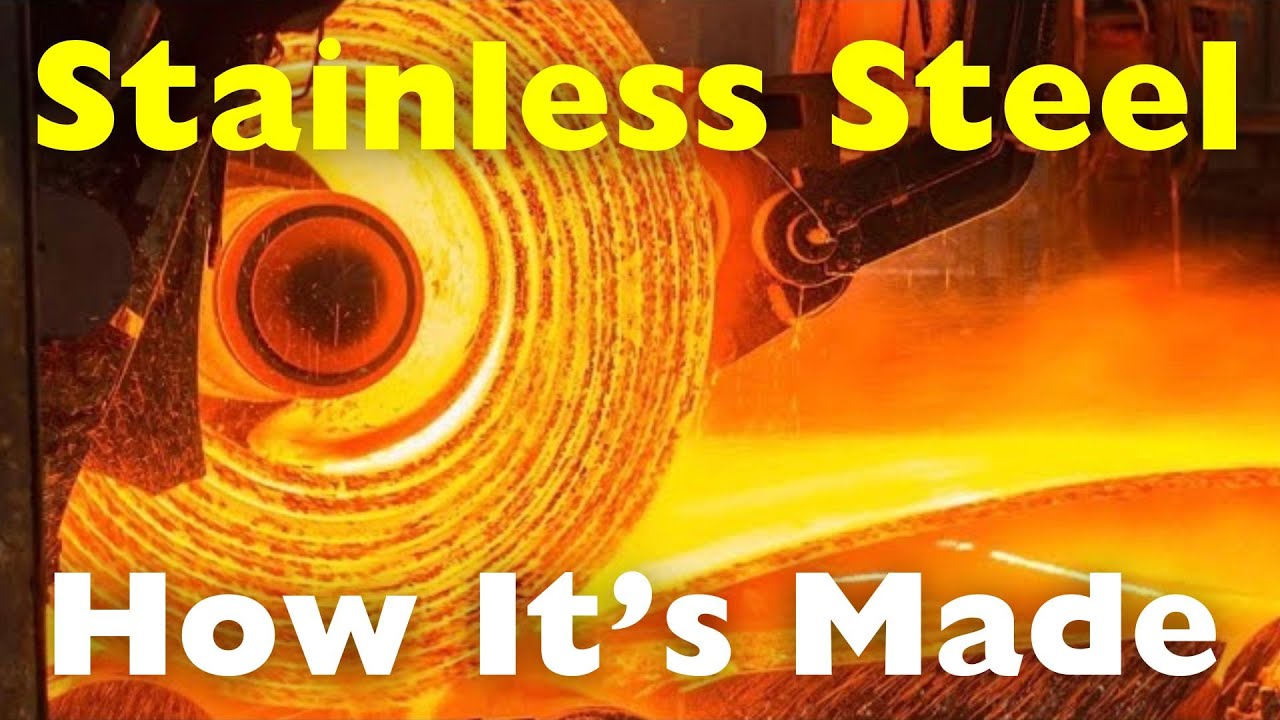
How Is Stainless Steel Made?
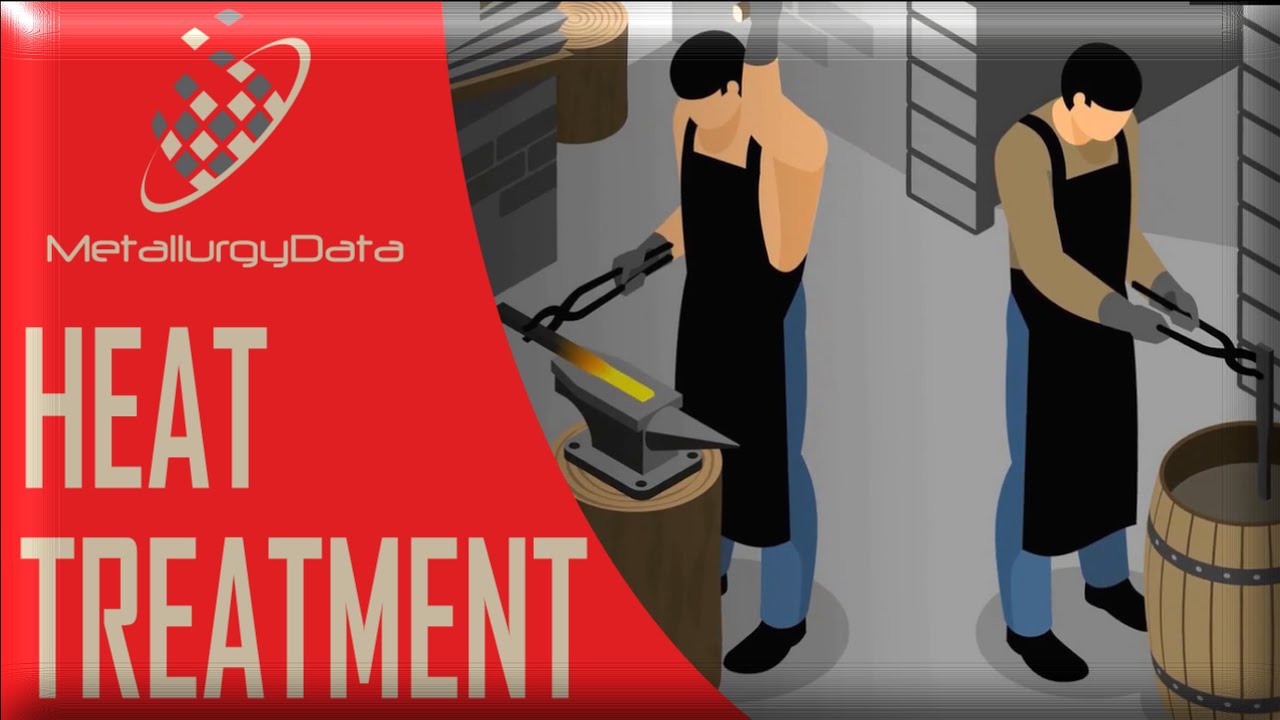
Heat Treatment - Types (Including Annealing), Process and Structures (Principles of Metallurgy)
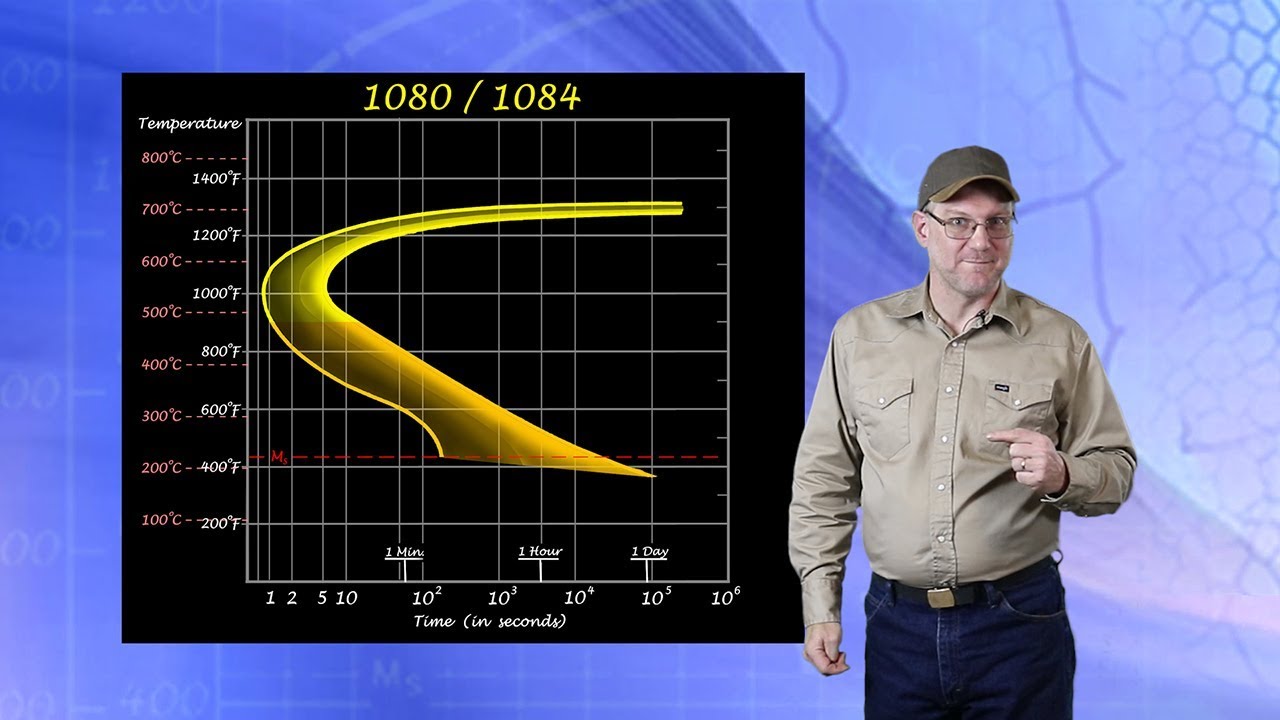
The TTT curve (1080/1084)
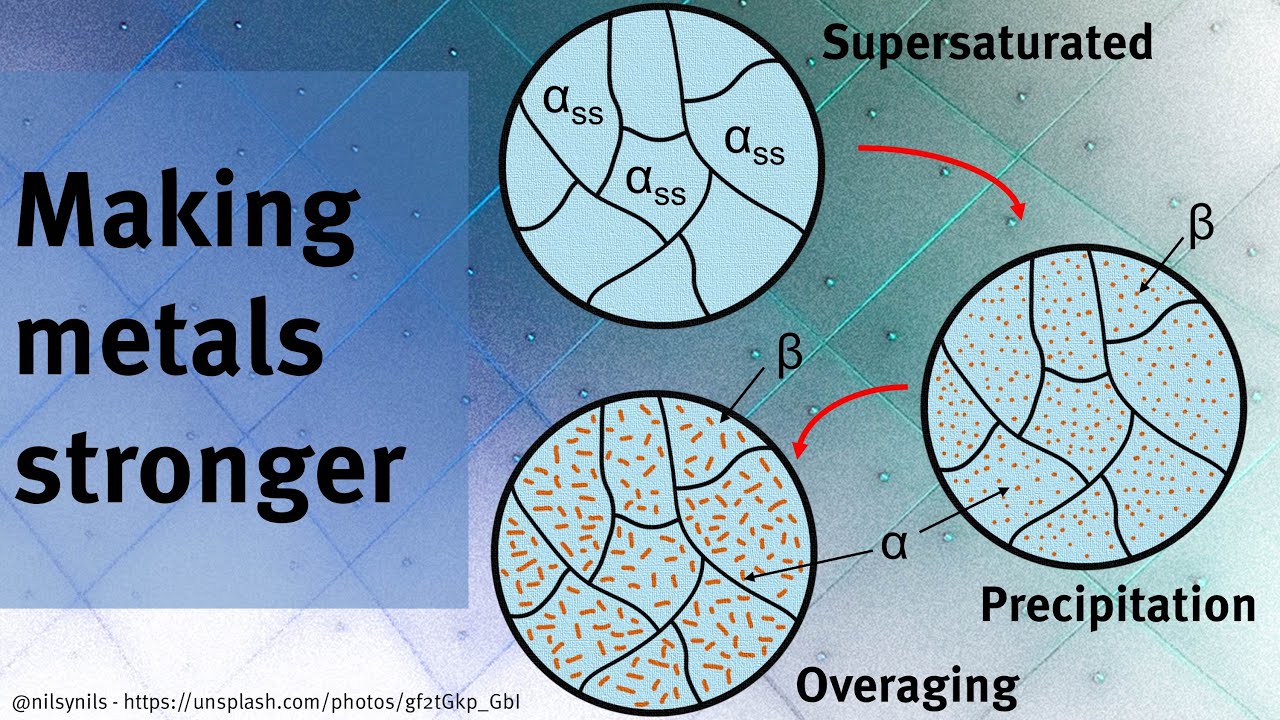
How to make metal stronger by heat treating, alloying and strain hardening
5.0 / 5 (0 votes)