FISIKA Kelas 12 - Induksi Elektromagnetik: Induktansi Diri | GIA Academy
Summary
TLDRThis educational YouTube video by Gia Akademi explores the concept of self-induction in electrical circuits. It explains how the gradual brightening and dimming of lights when switched on and off is due to the changing magnetic flux, inducing an electromotive force (EMF) that opposes the current change. The video delves into the principles of self-induction, including the formula for induced EMF, \( \varepsilon = -L \frac{\Delta I}{\Delta t} \), where \( L \) is the inductance, \( \Delta I \) is the change in current, and \( \Delta t \) is the change in time. It also covers the calculation of inductance in solenoids and toroids, the energy stored in inductors, and mutual inductance between two coils. The video concludes with practical examples and problems to solidify the understanding of self-induction.
Takeaways
- 🔌 The concept of self-induction is applied in everyday life, such as the gradual dimming of a light when switched off due to changes in magnetic flux.
- 💡 When turning on a light, the switch closes, and electricity flows through the circuit, creating a change in magnetic flux which causes the light to gradually brighten rather than instantly.
- 🌀 The gradual fading of a light when switched off is due to the change in magnetic flux as the electric current in the circuit is interrupted.
- ⚖️ The self-induced electromotive force (emf) is calculated using the formula ε = -L * (ΔI/Δt), where L is the self-inductance, and ΔI/Δt is the rate of change of current over time.
- 👨🔬 Joseph Henry, an American scientist, formulated the law stating that the magnitude of self-induced emf is proportional to the rate of change of current with respect to time.
- 🧲 The self-inductance of a coil can be determined by the formula L = n * ΔΦ/ΔI, where n is the number of turns, and ΔΦ is the change in magnetic flux.
- 📏 The self-inductance at the center of a solenoid can be calculated using the formula L = μ₀ * n² * a / l, with μ₀ being the permeability of free space.
- 🔗 The energy stored in a coil or inductor is given by the formula W = 1/2 * L * I², where W is the energy in joules, L is the inductance in henries, and I is the current in amperes.
- 🔄 Mutual or reciprocal inductance occurs between two coils when a changing current in one coil induces a changing magnetic flux in the other, calculated by M = N₁ * Φ₂ / I₂ = N₂ * Φ₁ / I₁.
- 📡 The inductance of a toroid can be determined using the formula L = μ₀ * N² * a / 2π, where a is the mean radius, N is the number of turns, and μ₀ is the permeability of free space.
Q & A
What is the concept of self-induction explained in the video?
-Self-induction is a phenomenon where a changing electric current in a circuit induces an electromotive force (EMF) in the same circuit due to the change in magnetic flux. This concept is demonstrated through the example of a light bulb, which doesn't turn on or off instantly when the switch is flipped.
How is the EMF of self-induction calculated?
-The EMF of self-induction is calculated using the formula ε = -L * (ΔI/Δt), where ε is the induced EMF, L is the self-inductance, and (ΔI/Δt) is the rate of change of current with respect to time.
Who is Joseph Henry and what is his contribution to the understanding of self-induction?
-Joseph Henry was an American scientist who formulated the law of self-induction, stating that the magnitude of the induced EMF is proportional to the rate of change of the magnetic flux, which can be expressed as ε = -L * (dI/dt).
What is the unit of self-inductance and what is its symbol?
-The unit of self-inductance is the Henry, symbolized as 'H'.
What is the relationship between the change in magnetic flux and the induced EMF according to Faraday's law?
-According to Faraday's law, a change in magnetic flux through a coil induces an EMF in the coil, which is proportional to the rate of change of the magnetic flux, expressed as ε = -n * (ΔΦ/Δt), where n is the number of turns in the coil and ΔΦ is the change in magnetic flux.
How is the self-inductance of a solenoid calculated?
-The self-inductance of a solenoid is calculated using the formula L = μ₀ * n² * A / l, where μ₀ is the permeability of free space, n is the number of turns per unit length, A is the cross-sectional area of the solenoid, and l is the length of the solenoid.
What is the energy stored in an inductor and how is it calculated?
-The energy stored in an inductor is given by the formula W = 1/2 * L * I², where W is the energy in joules, L is the inductance in henries, and I is the current in amperes.
What is mutual induction and how does it differ from self-induction?
-Mutual induction occurs when a changing current in one coil (primary) induces a current in a nearby coil (secondary) due to the changing magnetic field. It differs from self-induction, which occurs within the same coil. Mutual induction is calculated using the formula M = N₁ * Φ₂ / I₂ = N₂ * Φ₁ / I₁, where M is the mutual inductance, N₁ and N₂ are the number of turns in the primary and secondary coils, and Φ₁ and Φ₂ are the magnetic fluxes in the primary and secondary coils, respectively.
How is the self-inductance of a toroid calculated?
-The self-inductance of a toroid is calculated using the formula L = μ₀ * n² * A / (2π), where μ₀ is the permeability of free space, n is the number of turns, A is the cross-sectional area, and the division by 2π accounts for the toroid's geometry.
What is the significance of the problem-solving examples provided in the video?
-The problem-solving examples in the video demonstrate the practical application of the concepts of self-induction, mutual induction, and energy storage in inductors. They help in understanding how to calculate the induced EMF, self-inductance, and stored energy in different types of inductors.
Outlines
💡 Introduction to Self-Inductance
The script begins with an introduction to the concept of self-inductance using the everyday example of a light switch. It explains that when a light is turned on or off, the light doesn't immediately change state due to the principle of electromagnetic induction. The video aims to explore self-inductance in detail, including how it occurs and its applications in daily life. The script delves into the scientific explanation of self-inductance, mentioning the role of changing magnetic flux and how it induces an electromotive force (EMF) that opposes the change in current, as described by Faraday's law of induction and further elaborated by Joseph Henry.
🧲 Calculation of Self-Inductance in Solenoids and Toroids
This paragraph focuses on the calculation of self-inductance in solenoids and toroids. It discusses the formula for self-inductance at the center of a solenoid, which depends on the number of turns per unit length, the cross-sectional area, and the permeability of free space. The script also covers the self-inductance of a toroid, explaining how it is determined by similar factors. The mathematical relationships are simplified to provide formulas for calculating self-inductance in these coil configurations.
🔋 Energy Storage in Inductors and Mutual Inductance
The script moves on to discuss how inductors can store energy in a magnetic field, with the energy stored in an inductor being proportional to the square of the current flowing through it, as per the formula W = 1/2 * L * I^2. It then introduces the concept of mutual inductance, which occurs when two coils are in proximity and a changing current in one coil induces a voltage in the other. The mutual inductance is calculated using the number of turns in each coil and the magnetic flux linkage. The script provides examples of how mutual inductance is calculated for both solenoids and toroids.
📐 Practical Problems on Self-Inductance and Mutual Inductance
The final paragraph presents a series of practical problems to apply the concepts of self-inductance and mutual inductance. It includes problems such as calculating the induced EMF for a given coil with a time-varying current, determining the self-inductance of a coil based on the change in current and the resulting magnetic flux, and calculating the energy stored in a toroid. The script also covers the calculation of self-inductance for a telephone cable and mutual inductance between two solenoids. Each problem is solved step by step, demonstrating the application of the formulas introduced earlier in the script.
Mindmap
Keywords
💡Induction
💡Self-Induction
💡Electromotive Force (EMF)
💡Inductance
💡Magnetic Flux
💡Joseph Henry
💡Faraday's Law
💡Mutual Induction
💡Energy Storage
💡Solenoid
💡Toroid
Highlights
Induction is a concept applied in everyday life, such as the gradual dimming of lights when switched off.
Self-induction occurs when a change in electric current in a circuit induces a voltage in the same circuit.
The induced electromotive force (EMF) is proportional to the rate of change of the magnetic flux, as described by Faraday's law of induction.
Joseph Henry's law states that the magnitude of self-induced EMF is proportional to the rate of change of electric current over time.
The formula for self-induced EMF is given by ε = -L * (ΔI/Δt), where L is the self-inductance, and ΔI and Δt represent changes in current and time, respectively.
Self-inductance is measured in henries (H) and is calculated based on the change in magnetic flux and current in a coil.
The self-inductance of a solenoid can be determined using the formula L = μ₀ * n² * A / l, where μ₀ is the permeability of free space, n is the number of turns, A is the cross-sectional area, and l is the length of the solenoid.
The energy stored in an inductor can be calculated using the formula W = 1/2 * L * I², where W is the energy in joules, L is the inductance, and I is the current.
Mutual induction or transformer action occurs when a changing current in one coil induces a current in a nearby coil.
The mutual inductance between two coils is given by the formula M = N₁ * Φ₂ / I₂ = N₂ * Φ₁ / I₁, where M is the mutual inductance, N₁ and N₂ are the number of turns in each coil, and Φ₁ and Φ₂ are the magnetic fluxes.
The self-inductance of a toroid can be calculated using the formula L = μ₀ * n² * A / (2π), where A is the cross-sectional area and n is the number of turns.
An example problem demonstrates how to calculate the self-induced EMF given a time-varying current and inductance.
Another example shows how to determine the self-inductance of a coil given a change in current and the resulting change in magnetic flux.
The self-inductance of a telephone cable can be calculated by considering its spiral shape and the number of turns.
The energy stored in a toroid can be determined by calculating its self-inductance and the square of the current flowing through it.
Mutual inductance between two solenoids is calculated using the formula M = μ₀ * N₁ * N₂ * A / l, taking into account the geometry and number of turns of each solenoid.
The video concludes with a summary of the concepts of self-induction and mutual induction, encouraging viewers to continue learning about these topics.
Transcripts
[Musik]
Halo teman-teman selamat datang kembali
di channel YouTube Gia Akademi Semoga
teman-teman selalu sehat dan terus
semangat teman-teman biasa nya ketika
akan tidur kita mematikan lampu kamar
Pernahkah teman-teman memperhatikan
bahwa setelah kita menekan saklar lampu
tidak langsung padam melainkan redup
dahulu baru kemudian padam begitu juga
saat kita menghidupkan lampu setelah
saklar kita tekan lampu tidak langsung
menyala terang peristiwa ini merupakan
salah satu penerapan konsep induktansi
diri dalam kehidupan sehari-hari tahukah
teman-teman Apa itu induktansi diri
Bagaimanakah proses terjadi nya
induktansi diri kita akan membahas
materi ini secara lengkap di video kali
ini jadi di video ini kita akan belajar
tentang induktansi diri simak terus
videonya
ya teman-teman saat menghidupkan lampu
saklar ditutup lampu akan menyala dan
pada kumparan akan mengalir arus listrik
yang mengakibatkan timbulnya perubahan
fluks magnetik dari nol menuju nilai
tertentu Itulah sebabnya lampu tidak
langsung menyala terang saat kita
hidupkan sedangkan saat mematikan lampu
saklar dibuka lampu masih menyala sesaat
karena arus listrik di dalam rangkaian
akan terputus akibatnya pada kumparan
akan terjadi perubahan fluks magnetik
dari nilai tertentu kembali menjadi nol
ini juga yang menyebabkan lampu tidak
langsung padam ketika kita matikan
arus listrik yang berubah-ubah pada
rangkaian listrik menyebabkan fluks
magnetik pada kumparan juga mengalami
perubahan perubahan fluks magnetik Ini
menimbulkan GGL induksi diri yang
dinyatakan dengan persamaan εeps = - l *
Delta I / dengan Delta t dengan eps S
GGL induksi diri satuannya volt l
induktansi diri satuannya Henry Delta I
perubahan arus listrik satuannya amper
dan Delta t perubahan waktu satuannya
sek persamaan tersebut dirumuskan oleh
seorang ilmuwan Amerika bernama Joseph
Henry ia menyatakan bahwa besarnya GGL
induksi diri yang timbul akibat
perubahan fluks magnetik sebanding
dengan laju perubahan arus terhadap
waktu
Jika perubahan arus listrik terjadi
dalam waktu singkat atau Delta t
mendekati 0 GGL induksi diri ditentukan
dengan persamaan eps = - l *
di/dt dengan eps S GGL induksi diri
satuannya volt l induktansi diri
satuannya Henry di/dt turunan pertama
fungsi arus listrik terhadap waktu
satuannya ampere
perss pada materi sebelumnya kita sudah
mengetahui bahwa menurut farade adanya
perubahan garis gaya magnetik pada
kumparan akan menimbulkan GGL induksi
yang ditentukan dengan persamaan eps in
= -n * Delta Pi Dib Delta t menurut
Joseph Henry besarnya laju perubahan
arus listrik pada kumparan juga
bergantung pada perubahan garis gaya
magnetik sehingga dapat dinyatakan bahwa
ep s = εep in - l * Delta I / Delta t =
-n * Delta pi / dengan Delta t kita
Sederhanakan sehingga kita peroleh
persamaan induktansi diri l = n * Delta
V / Delta I
dengan l induktansi diri satuannya Henry
n jumlah lilitan Delta Pi perubahan
fluks magnetik satuannya Weber Delta I
perubahan arus listrik satuannya amper
dari persamaan tersebut dapat
disimpulkan bahwa induktansi diri suatu
kumparan sama dengan jumlah fluks
magnetik yang melingkupi kumparan tiap
satuan kuat arus listrik yang mengalir
pada kumparan
kita juga dapat menentukan besar
induktansi diri pada pusat solenoida
yaitu l = n * Pi / I karena Pi = b * a
maka l = n * b * a / i induksi magnetik
pada pusat solenoida adalah b = mi0 * n
* i / l maka Mak l = n * a / i * μ0 * n
* i / dengan l kita Sederhanakan
sehingga besar induktansi diri pada
pusat solenoida dapat ditentukan dengan
persamaan l = μ0 * n² * a /
l dengan l induktansi diri solenoida
satuannya Henry mu 0 permeabilitas ruang
hampa nilainya 4pi *
10^-7
weber/ampm n jumlah lilitan a luas
bidang kumparan satuannya met² dan l
panjang solenoida satuannya
meter dengan cara yang sama kita juga
dapat menentukan besar induktansi diri
pada toroida karena induksi magnetik
pada toroida adalah b = μ0 * n * i / 2P
maka l = n * a / i * μ0 * n * i / 2P
kita Sederhanakan sehingga besar
induktansi diri pada teroroida dapat
ditentukan dengan persamaan l = μ0 * n²
* a / 2π dengan l induktansi diri
toroida satuannya Henry miol
permeabilitas ruang hampa n jumlah
lilitan a luas bidang kumparan satuannya
meter² dan a jari-jari toroida satuannya
meter pemasangan kumparan pada rangkaian
listrik dapat menimbulkan GGL induksi
Oleh karena itu kumparan dikenal juga
dengan induktor energi yang tersimpan
dalam kumparan atau induktor dapat
ditentukan dengan persamaan w = 1/2 * l
* i² dengan W energi yang tersimpan
dalam induktor satuannya Joule l
induktansi diri satuannya Henry I kuat
arus listrik satuannya
amper selanjutnya kita akan membahas
mengenai induktansi silang atau dikenal
juga dengan induktansi timbal balik pada
gambar terlihat dua kumparan yaitu
kumparan primer P dan kumparan sekunder
S yang berdekatan satu sama lain apabila
hambatan reostat R diubah-ubah maka akan
mengalir arus yang berubah-ubah pada
kumparan primer sehingga akan timbul GGL
induksi pada kumparan sekunder GGL
induksi pada kumparan sekunder ini
menimbulkan arus induksi yang
berubah-ubah sehingga kembali
menimbulkan GGL induksi pada kumparan
primer GGL yang timbul pada kumparan
primer maupun kumparan sekunder ini
disebut induktansi silang atau
induktansi timbal balik yang dapat
ditentukan dengan persamaan m = N1 * pi2
/ I2 = N2 * pi1 /
I1 dengan m induktansi timbal balik
satuannya Henri N1 jumlah lilitan pada
kumparan 1 N2 jumlah lilitan pada
kumparan 2 pi1 fluks magnetik pada
kumparan 1 pi2 fluks magnetik pada
kumparan 2 keduanya dalam satuan Weber
I1 arus listrik pada kumparan 1 I2 arus
listrik pada kumparan dua keduanya dalam
satuan
amper dengan mensubstitusikan persamaan
fluks magnetik Pi = b * a dan induksi
magnetik solenoida b = μ0 * n * i / l
maka diperoleh rumus induktansi silang
pada solenoida sebagai berikut m = 0 *
N1 * N2 * a / l sedangkan besar
induktansi silang pada toroida
ditentukan dengan persamaan m = μ0 * N1
* N2 * a Dib dengan 2
pi dengan m induktansi timbal balik
satuannya Henri N1 jumlah lilitan pada
kumparan 1 N2 jumlah lilitan pada
kumparan 2 mi0 permeabilitas ruang hampa
a luas bidang kumparan satuannya meter²
F panjang solenoida dan a jari-jari
toroida keduanya dalam satuan meter
sampai di sini teman-teman paham
ya agar teman-teman semakin paham Mari
kita selesaikan contoh soal
berikut soal pertama Diketahui sebuah
kumparan dengan induktansi l = 0,8 Henry
dialiri arus listrik yang berubah-ubah
terhadap waktu dengan persamaan I = 2t^
- 4T + 3 I AMP dan t se saat GGL induksi
diri ep S terjadi sebesar
-1,6 volt kita diminta menentukan waktu
T untuk menjawab soal ini kita gunakan
persamaan
s = - l *
di/dt
-1,6 = -0 0,8 *
d/dt dari 2t² - 4T + 3 -1,6
/
-0,8 = 4T - 4 2 = 4T - 4 4T = 6 t = 6 /
4 = 1,5 se jadi jawabannya
C soal kedua Diketahui sebuah kumparan
terdiri atas n = 200 lilitan dalam waktu
Delta t = 0,1 se mengalami perubahan
kuat arus dari I1 = 6 AMP menjadi I2 = 8
AMP dan menimbulkan fluks magnetik
sebesar Delta Pi =
10^-3 Weber kita diminta menentukan
besar induk ansi diri l yang diberikan
kumparan untuk menjawab soal ini kita
gunakan persamaan l = n * deltaap /
dengan Delta I = 200 *
10^-3 di/ dengan 2 =
10^-1 sama juga dengan 0,1 Henry
Jawabannya
d soal selanjutnya diketahui kabel
telepon berbentuk spiral berdiameter d =
1 cm jumlah lilitan kabel n = 100
lilitan apabila panjang kabel saat tidak
direnggangkan l = 50 cm dan
permeabilitas ruang hampa 0 4P *
10^--7 weber/ AMPM kita diminta
menentukan induktansi diri l pada kabel
telepon tersebut untuk menjawab soal ini
terlebih dahulu kita tentukan luas kabel
telepon a =
1/4πd^ = 1/4 * pi * 1² =
0,25π cm² =
2,5p *
10^-5 m² selanjutnya kita Tentukan
induktansi diri kabel telepon berbentuk
spiral dengan persamaan l = 0 * n² * A /
dengan l kita masukkan nilainya kita
lakukan perhitungan kita peroleh nilai
induktansi diri dari kabel telepon
sebesar 2p^ *
10^-7 hry jadi jawabannya
B soal keempat diketahui sebuah toroida
terdiri dari n = 1000 lilitan dengan
jari-jari a = 20 cm toroida kemudian
dialiri arus listrik I = 10 AMP kita
diminta menentukan besar energi yang
tersimpan dalam toroida tersebut yaitu W
untuk menjawab soal ini terlebih dahulu
kita tentukan luas penampang toroida a =
pi a² = Pi * 2 *
10^-1 di = 4P *
10^-2 m² selanjutnya kita tentukan besar
induktansi diri toroida dengan persamaan
l = μ0 * n² * a / 2π kita masukkan
nilainya kita lakukan perhitungan kita
peroleh nilai l = 8p * 10^ -2 Henri
terakhir kita tentukan nilai energi yang
tersimpan dalam toroida dengan persamaan
w = 1/2 * l * i² = 1/2 * 8p * 10^ -2 *
10² = 4π Jou jawabannya
C soal terakhir diketahui solenoida
sepanjang l = 2
cm terdiri atas N1 = 500 lilitan di luar
solenoida dililitkan kawat sebanyak N2 =
200 lilitan Apabila luas penampang
solenoida a = 20
cm^ kita diminta menentukan induktansi
timbal balik kedua solenoida yaitu m
untuk menjawab soal ini kita gunakan
persamaan m = 0 * n 1 * N2 * a / l kita
masukkan nilainya kita lakukan
perhitungan kita peroleh nilai m = 40p *
10^-5 Henry ama juga dengan 4pi *
10^-4 Henry Jawabannya
a Oke teman-teman demikianlah pembahasan
kita tentang induktansi diri jangan lupa
tonton terus video-video terbaru di
channel kita ya sampai jumpa di video
[Musik]
[Tepuk tangan]
berikutnya
تصفح المزيد من مقاطع الفيديو ذات الصلة
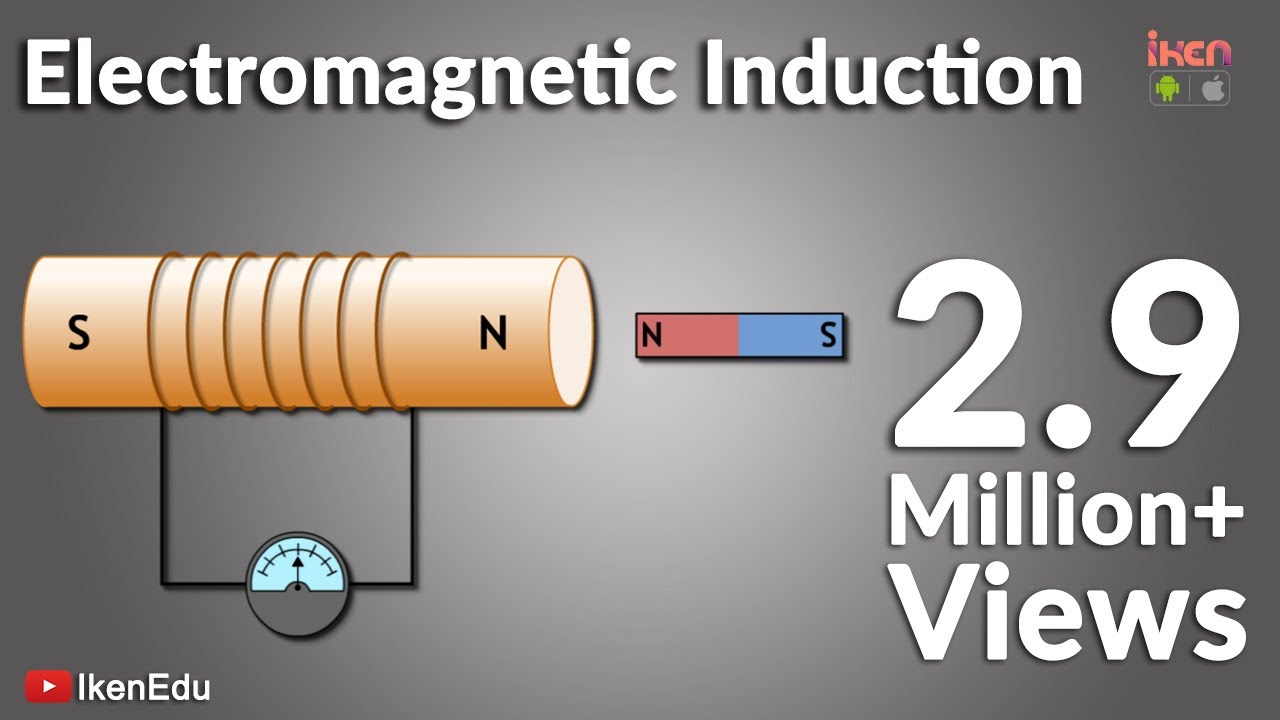
What is Electromagnetic Induction? | Faraday's Laws and Lenz Law | iKen | iKen Edu | iKen App

The Ultimate Guide to Understanding Inductance and Inductors
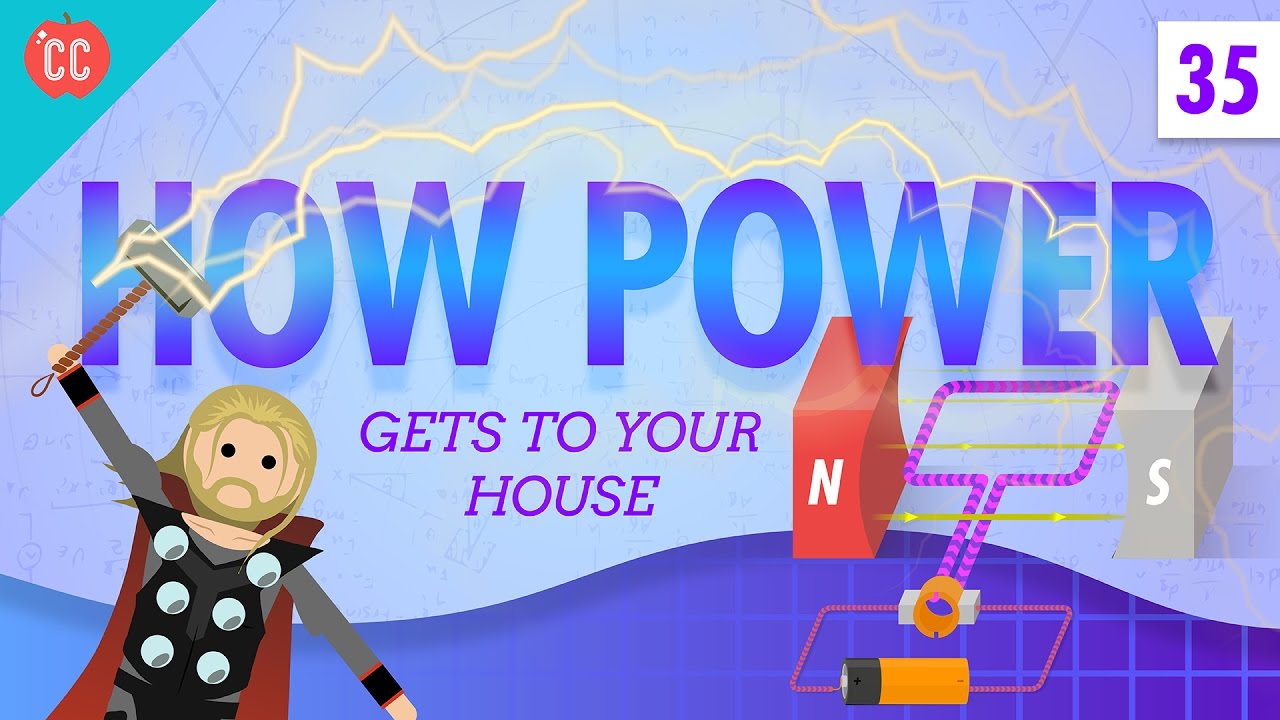
How Power Gets to Your Home: Crash Course Physics #35
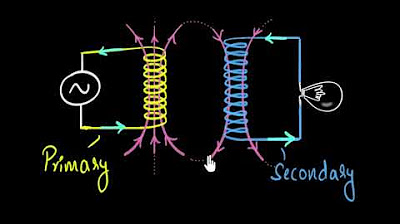
Transformers - working & applications (step up and step down) | A.C. | Physics | Khan Academy
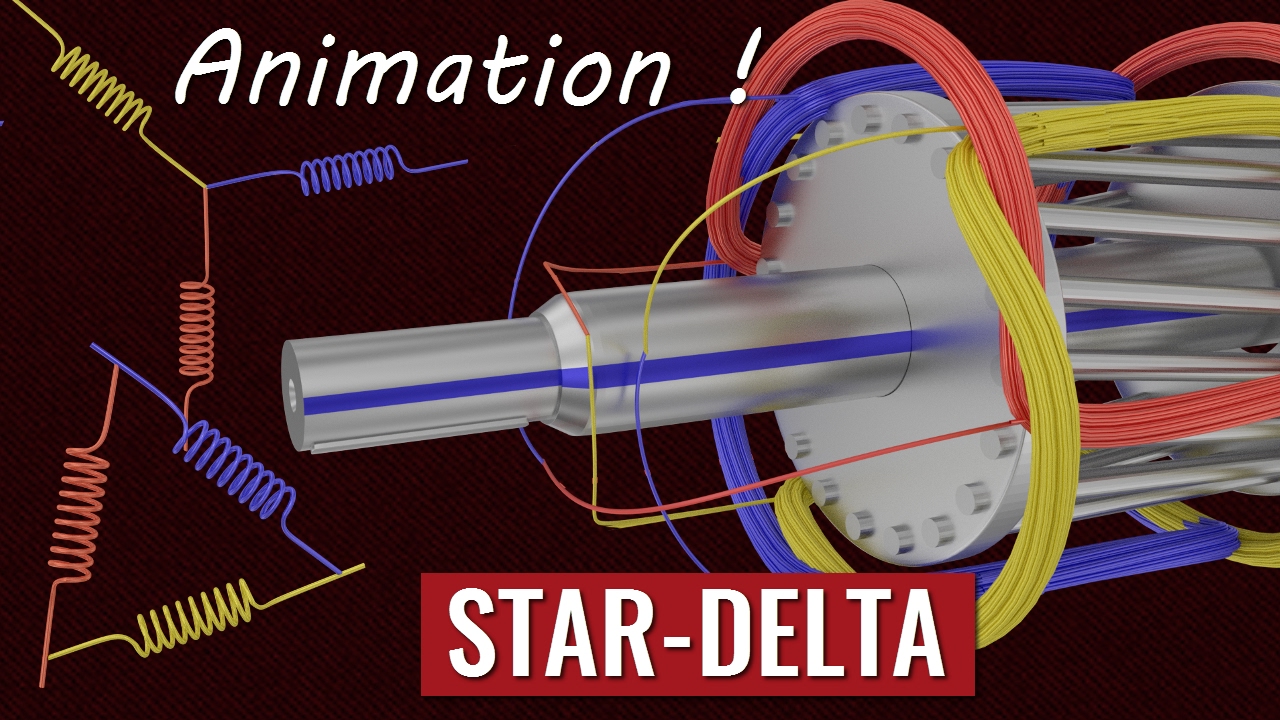
Understanding STAR-DELTA Starter !
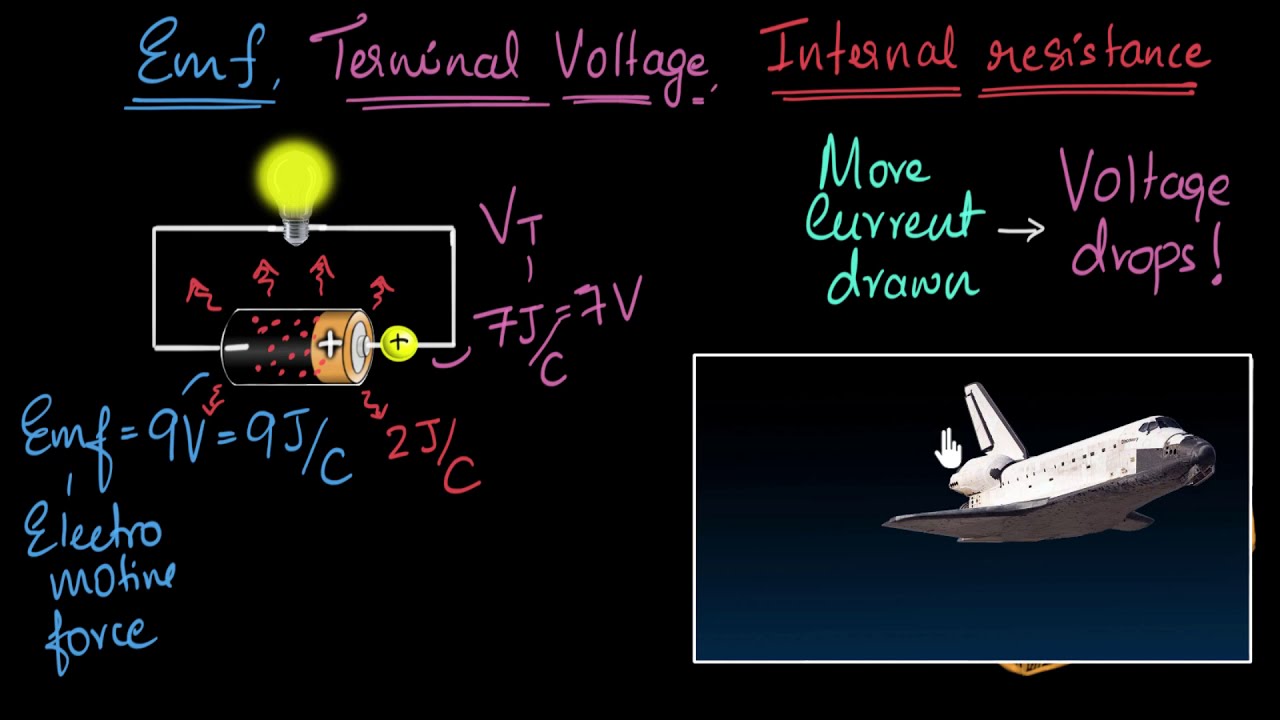
Cells, EMF, terminal voltage & internal resistance | Electric current | Physics | Khan Academy
5.0 / 5 (0 votes)