Neuron action potential mechanism | Nervous system physiology | NCLEX-RN | Khan Academy
Summary
TLDRThis video explores the generation and conduction of action potentials in neurons. It explains how action potentials are initiated at the axon's trigger zone, where voltage-gated sodium channels open in response to reaching the threshold potential. The influx of sodium ions causes a rapid depolarization, leading to the rising phase of the action potential. The falling phase is triggered by the outflow of potassium ions through leak and voltage-gated potassium channels. The video also discusses the refractory period, which prevents immediate reactivation of the action potential, ensuring unidirectional signal transmission along the axon.
Takeaways
- 🧠 The video discusses the generation and conduction of action potentials, focusing on the trigger zone and the axon.
- 🌟 The axon is depicted with a high concentration of voltage-gated ion channels, which are crucial for the initiation of action potentials.
- 🔋 The resting membrane potential is typically around -60 millivolts, and the threshold potential is around -50 millivolts.
- ⚡ Action potentials are initiated when the membrane potential at the trigger zone reaches the threshold potential, causing voltage-gated sodium channels to open.
- 💧 Sodium ions rush into the neuron through the open sodium channels, leading to depolarization and the rising phase of the action potential.
- 🔼 The action potential peaks at around +40 millivolts, not reaching the sodium equilibrium potential due to the inactivation of sodium channels.
- 🔄 The falling phase of the action potential is caused by the efflux of potassium ions through leak channels and voltage-gated potassium channels.
- 🔽 The action potential returns to the resting potential as voltage-gated potassium channels close, and the membrane permeability to potassium normalizes.
- 🚫 The refractory period follows the action potential, during which it is difficult or impossible to trigger another action potential in the same membrane area.
- 🛑 The refractory period consists of an absolute refractory period, where sodium channels are inactivated, and a relative refractory period, where additional stimulation is required to initiate a new action potential.
Q & A
What is the primary focus of the video?
-The video primarily focuses on explaining how action potentials are generated, the role of the trigger zone, and how these action potentials are conducted down the axon.
What is the resting membrane potential of a neuron?
-The resting membrane potential of a neuron is typically around negative 60 millivolts.
What is the threshold potential and how does it relate to the generation of an action potential?
-The threshold potential is around negative 50 millivolts, and it is the value that, when crossed, causes voltage-gated ion channels to open, leading to the generation of an action potential.
What are voltage-gated ion channels and how do they contribute to the action potential?
-Voltage-gated ion channels are a type of ion channel that opens in response to changes in membrane potential. They play a crucial role in the action potential by allowing sodium ions to flow into the neuron, causing depolarization, which initiates the action potential.
What is the trigger zone and why is it significant?
-The trigger zone is the initial segment of the axon where action potentials usually start. It is significant because it has the greatest density of voltage-gated sodium channels, which allows the action potential to initiate.
Describe the rising phase of an action potential.
-The rising phase of an action potential is when the membrane potential rapidly increases due to the influx of sodium ions through the voltage-gated sodium channels, making the inside of the neuron more positive than the outside.
Why does the action potential not reach the sodium equilibrium potential?
-The action potential does not reach the sodium equilibrium potential because the voltage-gated sodium channels automatically start to close at higher potential values, preventing further sodium influx.
What causes the falling phase of the action potential?
-The falling phase of the action potential is caused by the efflux of potassium ions through leak channels and voltage-gated potassium channels, which helps to repolarize the neuron back towards its resting potential.
What is the refractory period and why is it important?
-The refractory period is a time after an action potential during which it is difficult or impossible to trigger another action potential in the same part of the membrane. It is important because it prevents the action potential from traveling back along the axon and ensures unidirectional conduction.
How is the refractory period divided and what are its parts?
-The refractory period is divided into two parts: the absolute refractory period, when voltage-gated sodium channels are inactivated and cannot open, and the relative refractory period, when these channels are functional again but the membrane potential is hyperpolarized, requiring more excitatory input to trigger another action potential.
How does the movement of ions across the membrane cause the waveform of the action potential?
-The movement of sodium and potassium ions across the membrane, through voltage-gated and leak channels, causes the characteristic waveform of the action potential, including its rising, peak, falling phases, and after-hyperpolarization.
Outlines
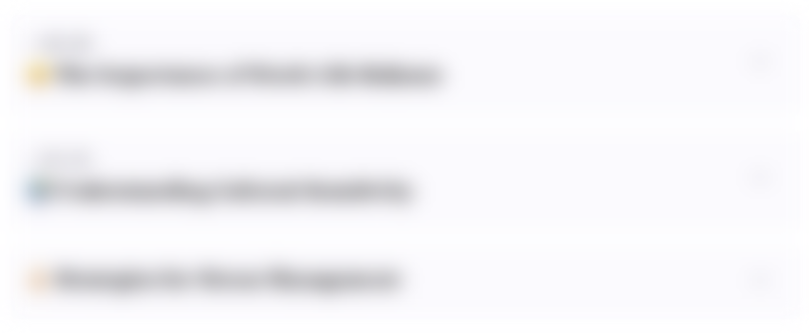
此内容仅限付费用户访问。 请升级后访问。
立即升级Mindmap
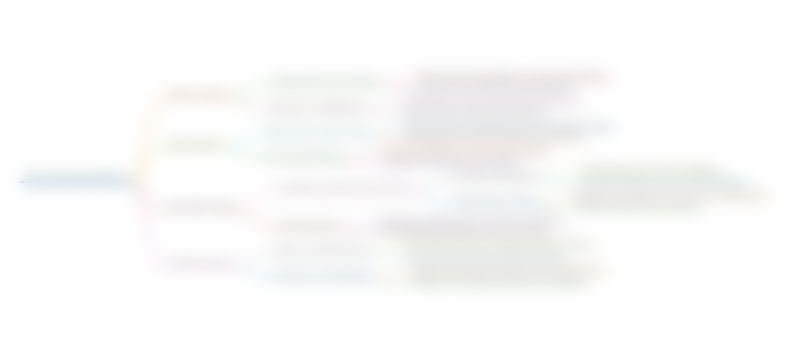
此内容仅限付费用户访问。 请升级后访问。
立即升级Keywords
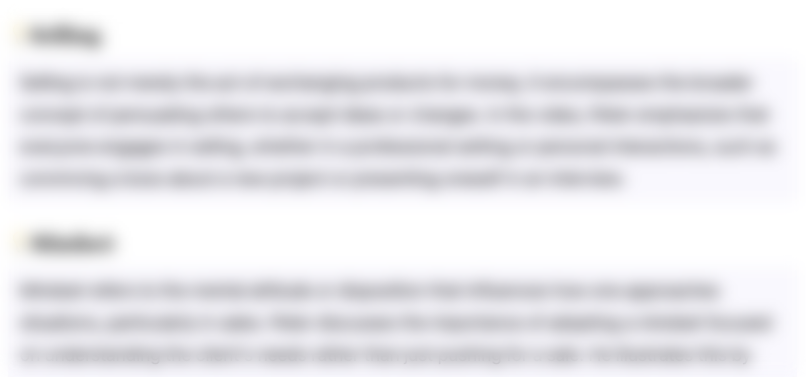
此内容仅限付费用户访问。 请升级后访问。
立即升级Highlights
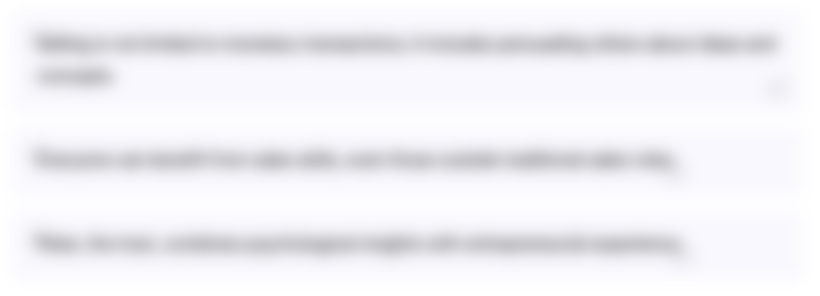
此内容仅限付费用户访问。 请升级后访问。
立即升级Transcripts
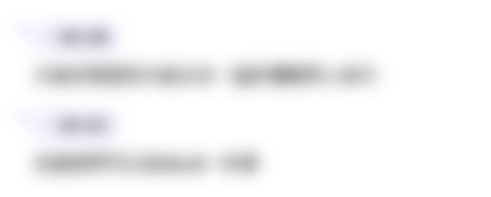
此内容仅限付费用户访问。 请升级后访问。
立即升级5.0 / 5 (0 votes)