Spin qubits | QuTech Academy
Summary
TLDRThis script chronicles the evolution of semiconductor technology, from the first transistor in 1947 to the development of integrated circuits and quantum bits. It delves into the intricacies of isolating and controlling individual electrons using quantum dots and charge sensing, highlighting the potential for large-scale quantum circuits. Collaborations with industry leaders like Intel are paving the way for standardized production of quantum dot arrays, aiming for thousands of qubits in compact, efficient designs.
Takeaways
- 🔬 The first transistor was demonstrated in 1947, marking a significant milestone in semiconductor technology.
- 📻 The first transistor radio was developed shortly after the invention of the transistor, utilizing multiple transistors along with capacitors and resistors on a circuit.
- 🛠 The initial transistor technology had limitations due to the manual assembly of components, which led to the development of the integrated circuit in 1958.
- 💡 Integrated circuits allowed for the integration of multiple components in a single piece of germanium or silicon, paving the way for complex processors and memory chips.
- 🚀 In the last decade, the same core technology of transistors and integrated circuits has been used to create high-quality quantum bits.
- 🛑 A transistor functions as a switch that controls electron flow using a gate voltage, and by modifying this design, quantum dots can be created to isolate electrons.
- 🔋 Quantum dots are small spaces where electrons are isolated, and at the extreme limit, a single electron can be isolated to represent a quantum bit.
- ⚡ The concept of charging energy, resulting from the Coulomb repulsion between electrons, is crucial for controlling the number of electrons in quantum dots.
- 🔍 Charge sensing is a method used to detect the presence of individual electrons by observing changes in current through neighboring quantum dots.
- 🔄 The development of large-scale quantum circuits requires uniformity and precision, which has led to collaborations with industry-standard clean rooms like Intel.
- 🔗 Cross-bar technology, similar to that used in displays and memory chips, is being explored to wire and control two-dimensional arrays of quantum dots.
- 🌐 The vision for quantum computing includes the integration of local arrays with quantum links to transfer entanglement and classical electronics for efficient signal distribution.
Q & A
What was the significance of the first transistor demonstrated in 1947?
-The first transistor in 1947 marked a pivotal moment in the development of semiconductor technology, paving the way for the miniaturization and integration of electronic components, which eventually led to the creation of more complex devices such as transistor radios.
How did the invention of the transistor radio represent a limitation in the early stages of semiconductor technology?
-The transistor radio, despite being a remarkable innovation, highlighted the limitations of manually soldering components onto a circuit board. It raised the question of how many components could realistically be assembled in this manner, indicating the need for a more efficient method of integration.
What was the second major advancement in semiconductor technology mentioned in the script?
-The second major advancement was the demonstration of the integrated circuit in 1958, which integrated all relevant components monolithically in a single piece of germanium or silicon, leading to the development of powerful processors and memory chips used today.
How are quantum bits related to the technology of transistors and integrated circuits?
-Quantum bits, or qubits, are built using the same core technology of transistors and integrated circuits. The development of high-quality qubits in the last decade has been facilitated by the ability to isolate and control individual electrons using the principles that govern transistor operation.
What is a quantum dot and how is it used in the context of qubits?
-A quantum dot is a small space where electrons are isolated below a gate, separated from other puddles of electrons. By taking the concept of a transistor and using multiple gates to isolate individual electrons, the spin of these electrons can be used as a quantum bit for quantum computing.
What is the concept of charging energy in the context of quantum dots?
-Charging energy is the energy resulting from the Coulomb repulsion between electrons. In the context of quantum dots, the charging energy is the energy required to add one single electron charge to the quantum dot, which can be larger than the thermal energy at low temperatures, enabling the control of individual electron charges.
How is the number of charges on a quantum dot controlled at low temperatures?
-At temperatures as low as 4K, the charging energy required to add a single electron charge can be larger than the thermal energy, allowing for precise control of the number of charges on the quantum dot, effectively enabling the isolation of individual electrons.
What is Coulomb blockade and how does it relate to the flow of electrons in a quantum dot?
-Coulomb blockade is a phenomenon where the flow of electrons is blocked due to the energy required to add an electron being larger than the energy available from the Fermi energy of the reservoirs. This blockade can be lifted by adjusting the gate voltage to align the electrochemical potential within the biased window between source and drain, allowing individual electrons to move through the quantum dot.
What is charge sensing and how is it used to detect individual electrons in quantum dots?
-Charge sensing is a method that involves using a neighboring quantum dot to detect the presence or absence of individual electrons in a primary quantum dot. A single charge in the neighboring dot can shift the energy levels in the primary dot through capacitive coupling, changing the current flow in a measurable way.
How does the script suggest scaling up from simple quantum dot arrays to large-scale quantum circuits?
-The script suggests that to scale up, quantum dots need to be made as identical and uniform as possible. It also mentions the need for industry-standard clean rooms for producing high-quality quantum dot arrays and the use of cross-bar technology for wiring in two-dimensional arrays. The integration of classical electronics with qubits and the development of quantum links for transferring quantum information are also discussed.
What is the envisioned size of a single array containing 1024 qubits according to the script?
-The script envisions that an array containing approximately 1024 qubits could be integrated into an area no larger than 30 x 30 micrometres, using cross-bar technology for efficient wiring.
Outlines
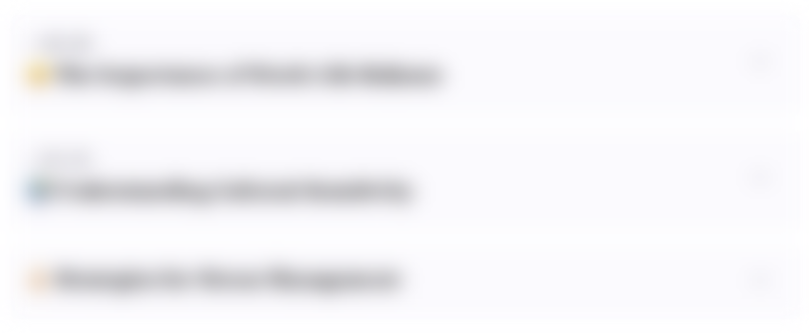
This section is available to paid users only. Please upgrade to access this part.
Upgrade NowMindmap
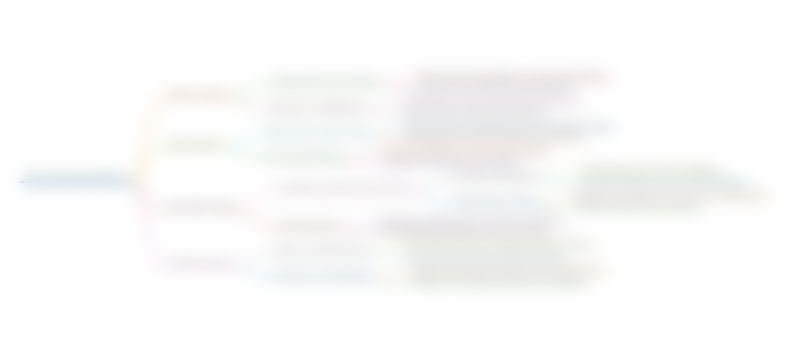
This section is available to paid users only. Please upgrade to access this part.
Upgrade NowKeywords
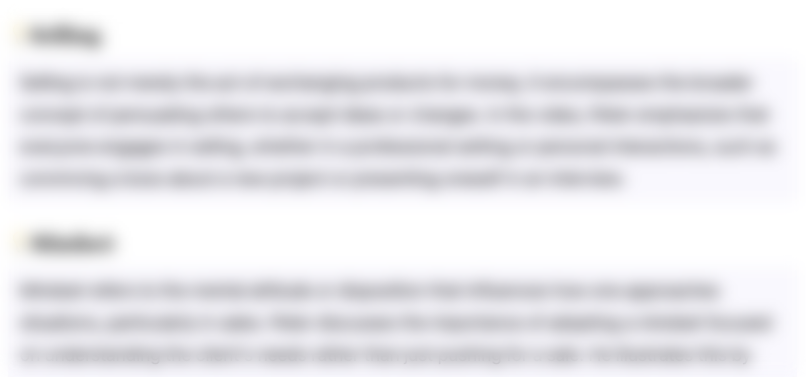
This section is available to paid users only. Please upgrade to access this part.
Upgrade NowHighlights
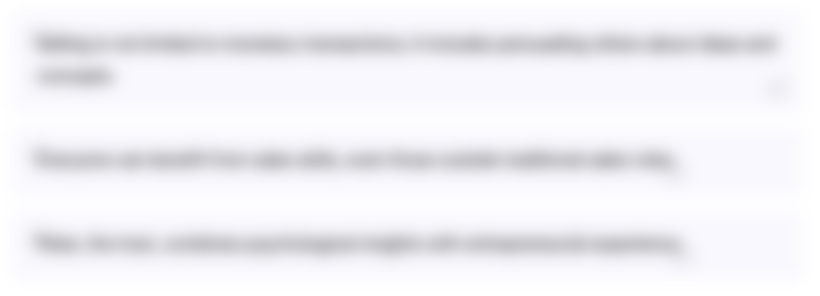
This section is available to paid users only. Please upgrade to access this part.
Upgrade NowTranscripts
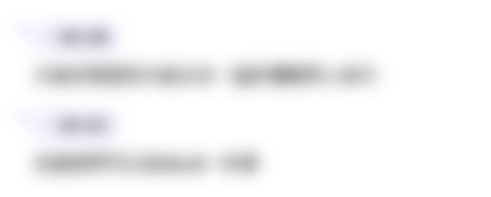
This section is available to paid users only. Please upgrade to access this part.
Upgrade NowBrowse More Related Video
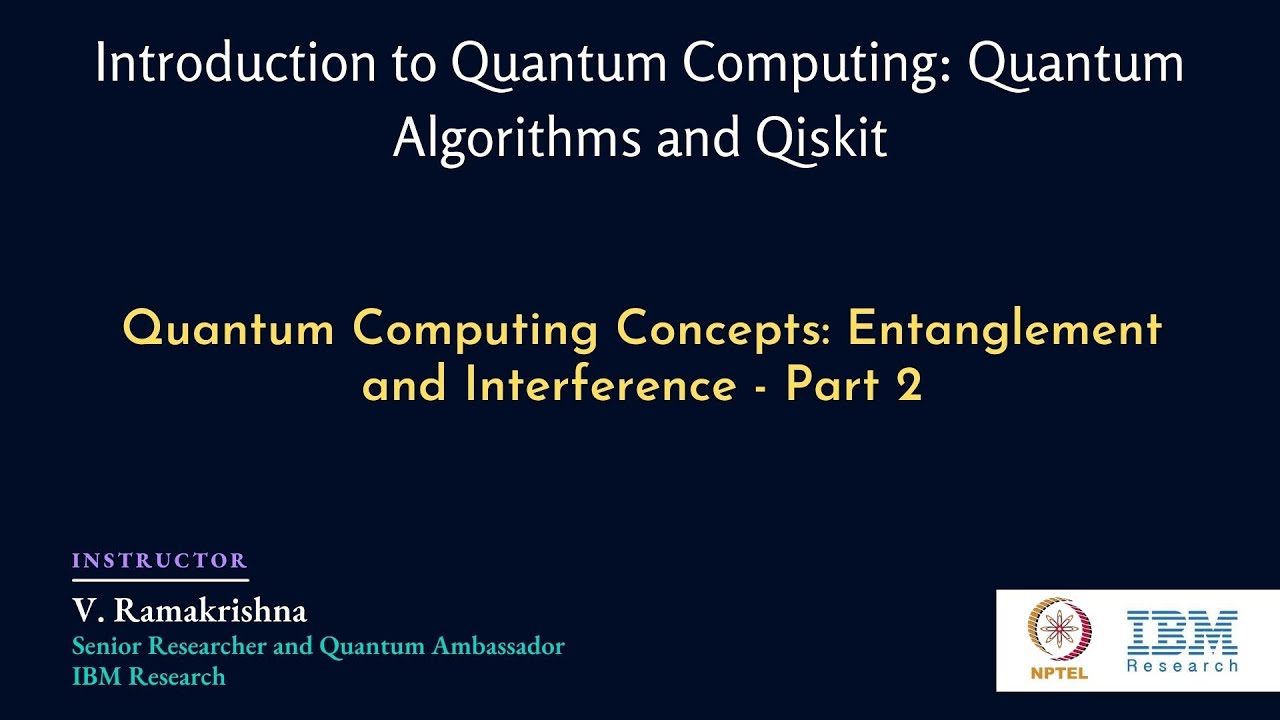
mod02lec12 - Quantum Computing Concepts: Entanglement and Interference - Part 2
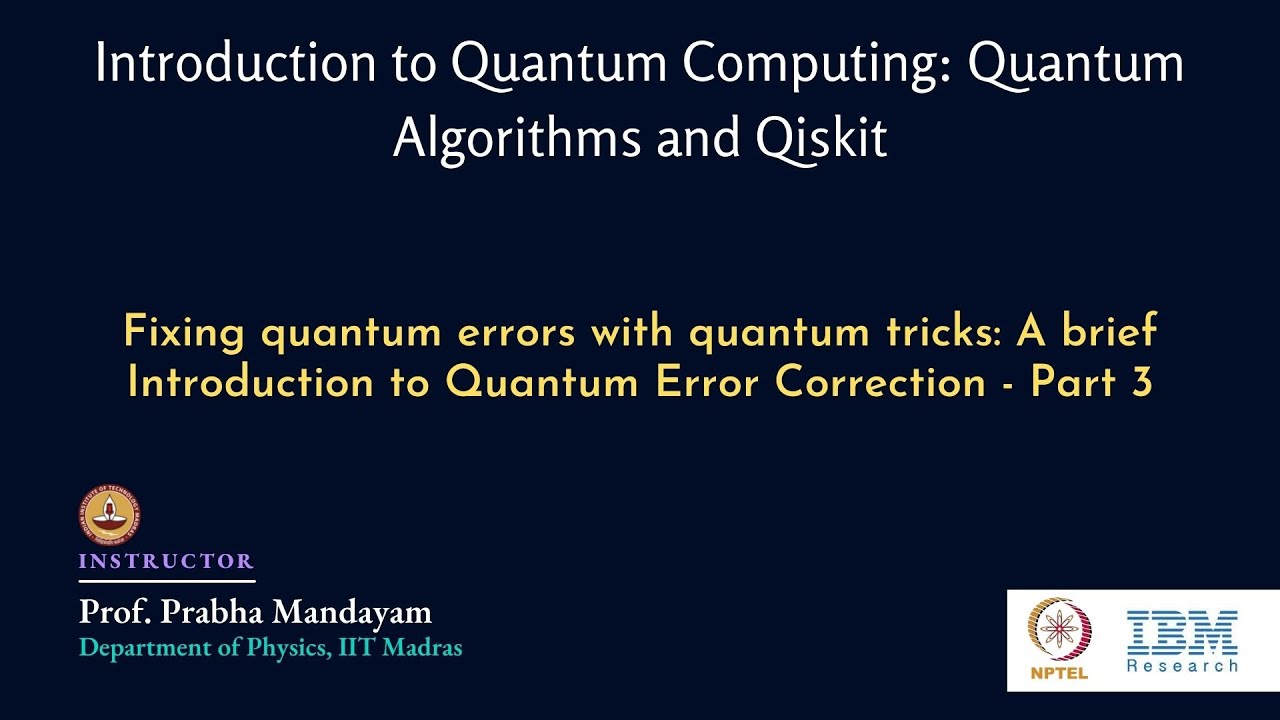
mod04lec25 - Fixing quantum errors with quantum tricks: A brief introduction to QEC - Part 3
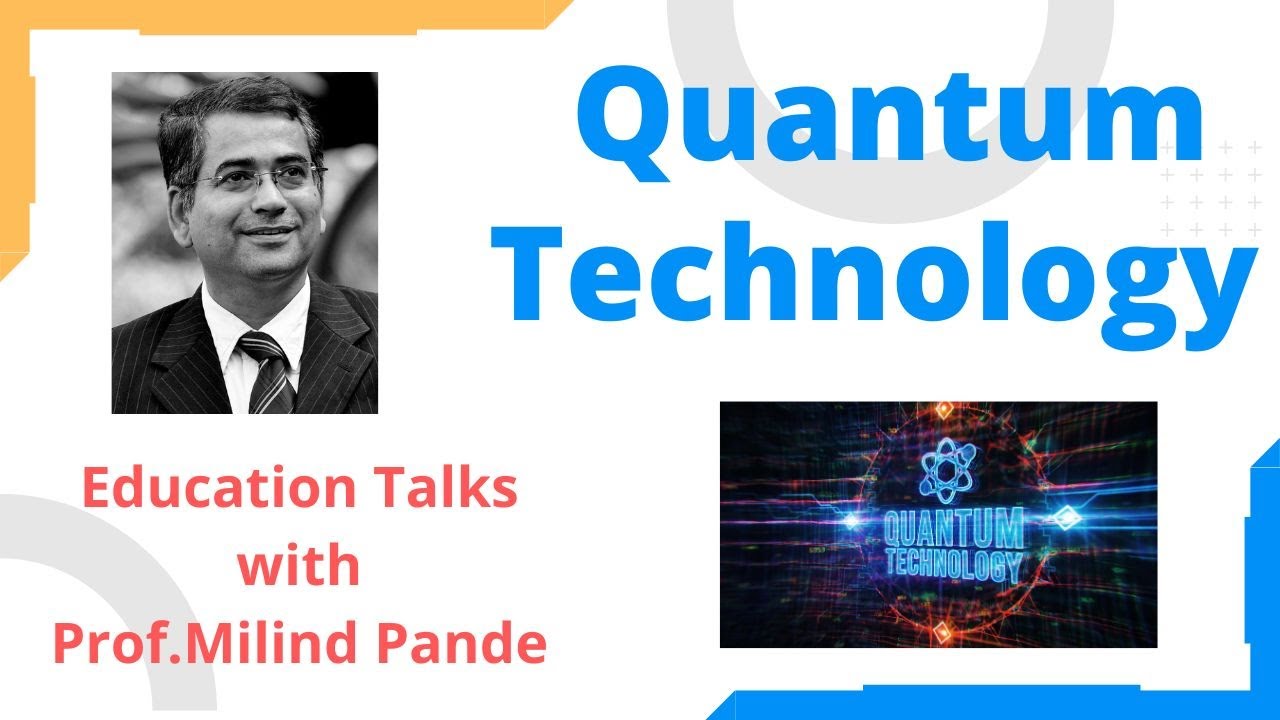
Quantum Computing in Marathi | Edu. Talk with Prof. Milind Pande
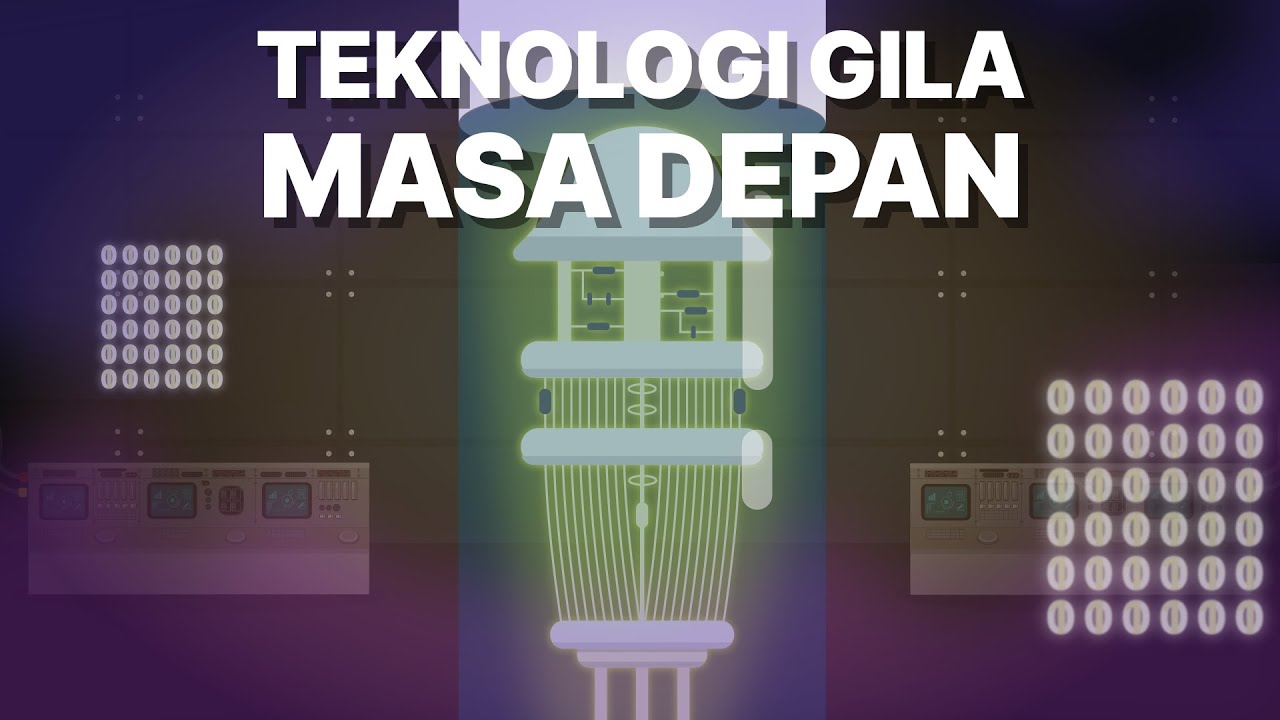
Apa itu Komputer Kuantum Sebenarnya?
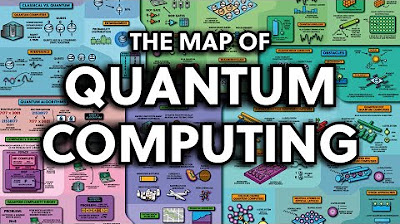
The Map of Quantum Computing - Quantum Computing Explained

Quantum Computers, Explained With Quantum Physics
5.0 / 5 (0 votes)