MRI physics overview | MRI Physics Course | Radiology Physics Course #1
Summary
TLDRThis introductory MRI physics module aims to build a comprehensive understanding of MRI imaging principles. It compares learning to assembling a puzzle, emphasizing the importance of gradually mastering individual concepts before integrating them for a complete picture. The script covers the basics, including the role of hydrogen atoms, the Cartesian plane for image localization, and the significance of magnetic moments and resonance frequencies. It also introduces key MRI parameters like TE and TR, which are crucial for generating image contrast, and hints at more complex topics like pulse sequences and k-space that will be explored in subsequent lectures.
Takeaways
- π§© The MRI physics module is designed to build a comprehensive understanding of MRI principles through multiple detailed talks.
- π§ The learning process is likened to solving a puzzle, where concepts are broken down and mastered before being combined for a complete picture.
- π MRI imaging uses the Cartesian plane to localize signals within the patient, with the Z-axis representing the longitudinal axis and the X-Y plane for transverse sections.
- π Nuclear magnetic resonance (NMR) is central to MRI, utilizing a large magnetic field to resonate hydrogen atoms, which are abundant and have a non-zero spin in the human body.
- π§² Hydrogen atoms, acting as tiny bar magnets, align and precess around their axis when subjected to an external magnetic field, creating a magnetic moment.
- π The net magnetization vector, representing the combined magnetic moments of hydrogen atoms, is crucial for MRI imaging and is influenced by the external magnetic field.
- π The radio frequency (RF) pulse is applied perpendicular to the main magnetic field to move the net magnetization vector into the transverse plane, enabling signal measurement.
- π The loss of transverse magnetization, or T2 decay, and the regaining of longitudinal magnetization, or T1 recovery, are key processes that occur independently and are exploited to generate image contrast.
- π The flip angle of the net magnetization vector, induced by the RF pulse, is a critical parameter in MRI imaging, affecting the signal generated and the resulting image contrast.
- πΊ The time of echo (TE) and time of repetition (TR) are adjustable parameters that control the contrast in MRI images by manipulating the signal's sensitivity to T1 and T2 relaxation times.
- π Advanced imaging techniques, pulse sequences, and the use of k-space for data storage and image creation will be explored in further detail throughout the module.
Q & A
What is the main difference between MRI imaging and other imaging modalities like X-ray, CT, and ultrasound?
-The main difference is that in MRI imaging, the signal used to generate the image comes from within the patient, specifically from the hydrogen atoms in the body, whereas in X-ray, CT, and ultrasound, the signal comes from external sources or mechanical vibrations.
What is the Cartesian plane used for in MRI imaging?
-The Cartesian plane is used to localize the signal within the patient. It separates the image into three axes: the longitudinal (Z or z-axis), and the transverse (X-Y plane), which are essential for understanding the orientation of the imaging slices.
Why is the hydrogen atom used in MRI imaging?
-The hydrogen atom is used because it is abundant in the human body, and it has a non-zero spin, which means it acts as a tiny bar magnet with a north and south pole, contributing to the magnetic moment used in image generation.
What is nuclear magnetic resonance and how is it relevant to MRI imaging?
-Nuclear magnetic resonance is a phenomenon where certain atomic nuclei, like hydrogen, resonate in a large magnetic field. In MRI imaging, this principle is used to induce resonance in hydrogen atoms within the patient, which is essential for image formation.
How does the magnetic field influence the hydrogen atoms in the MRI scanner?
-The magnetic field causes the hydrogen atoms to align with it and precess around their own axis at a frequency determined by the type of atom and the strength of the magnetic field.
What is the significance of the net magnetization vector in MRI imaging?
-The net magnetization vector represents the combined magnetic moments of all the free hydrogen atoms in the body. It is crucial for MRI imaging as it is the vector that is manipulated and measured to generate the image.
What is the purpose of the radio frequency (RF) pulse in MRI imaging?
-The RF pulse is applied to move the net magnetization vector perpendicular to the main magnetic field, allowing for the measurement of the signal. It also causes the protons to precess in phase, which is necessary for generating a detectable signal.
What is the flip angle in the context of MRI imaging?
-The flip angle refers to the angle at which the net magnetization vector is tilted from its alignment with the main magnetic field to a position perpendicular to it by the RF pulse.
Why is it important to consider the time of echo (TE) and time of repetition (TR) in MRI imaging?
-The TE and TR are parameters that control the timing of the MRI pulse sequence, affecting the contrast in the images by exploiting differences in the relaxation times (T1 and T2) of various tissues.
What is the role of k-space in MRI imaging?
-K-space is a mathematical space used to encode the data for different slices in an MRI image. It is filled with data that is then used to reconstruct the image by stacking the k-spaces on top of one another.
What are the different types of pulse sequences mentioned in the script, and what is their significance?
-The script mentions spin echo, inversion recovery, and gradient echo sequences. These pulse sequences are used to generate images with different contrasts and are essential for understanding various tissue properties and diagnosing conditions.
Outlines
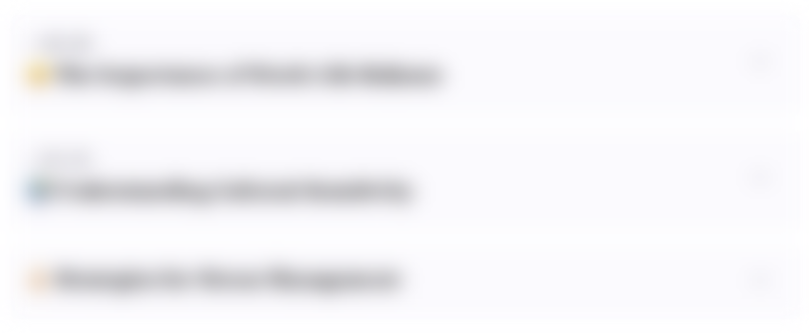
This section is available to paid users only. Please upgrade to access this part.
Upgrade NowMindmap
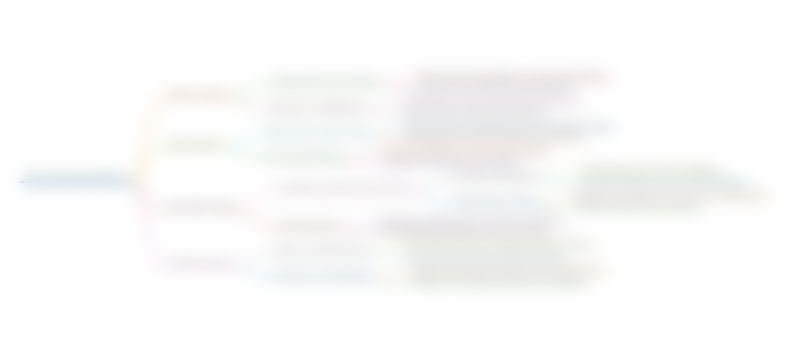
This section is available to paid users only. Please upgrade to access this part.
Upgrade NowKeywords
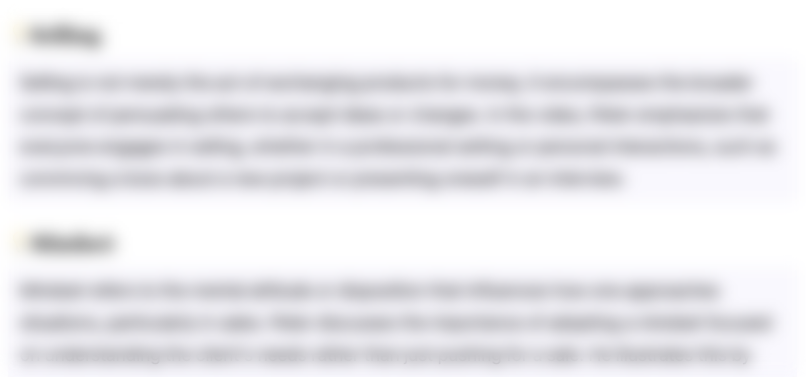
This section is available to paid users only. Please upgrade to access this part.
Upgrade NowHighlights
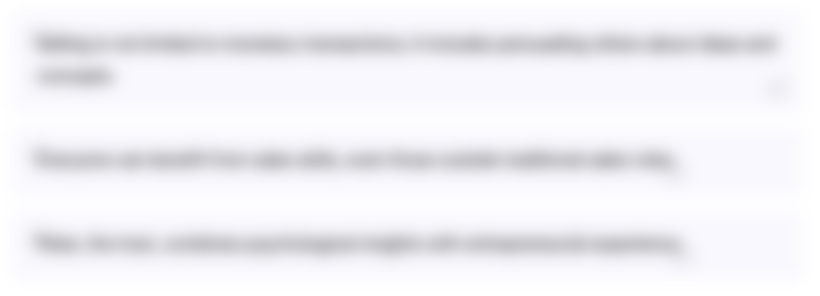
This section is available to paid users only. Please upgrade to access this part.
Upgrade NowTranscripts
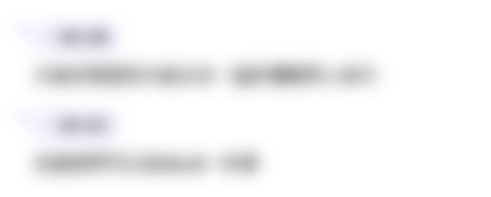
This section is available to paid users only. Please upgrade to access this part.
Upgrade NowBrowse More Related Video
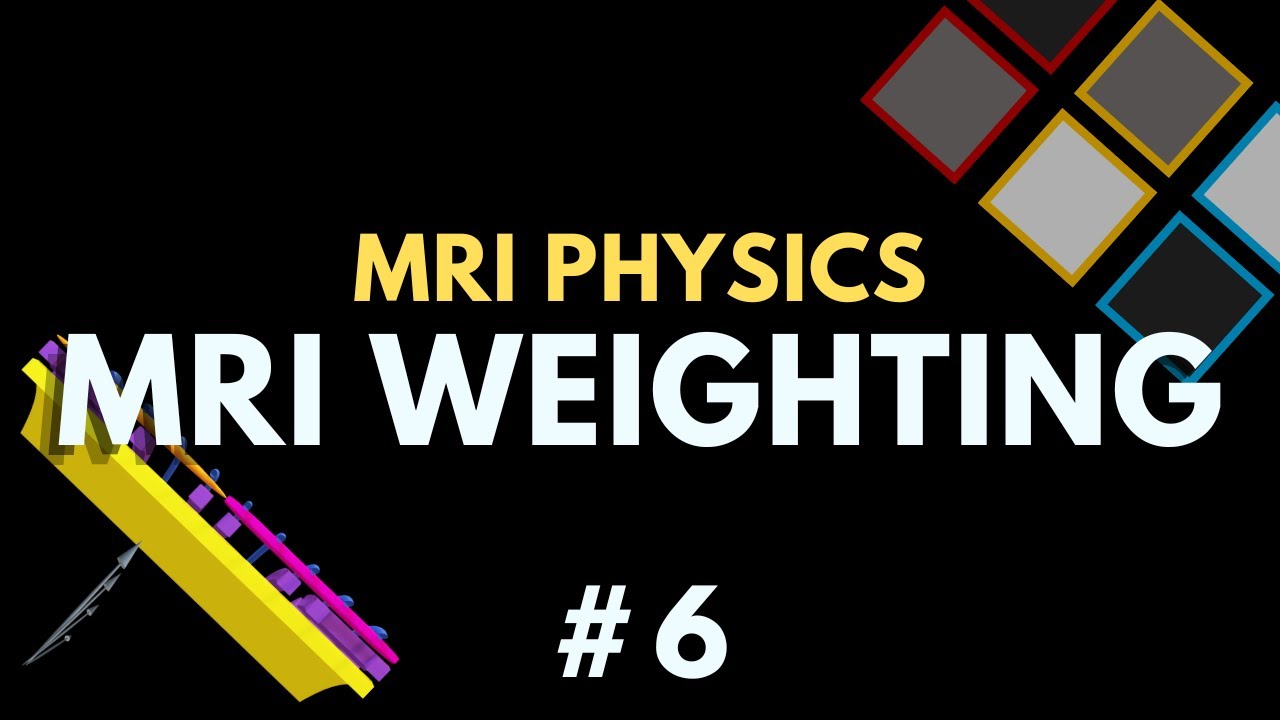
T1, T2 and Proton Density Weighting | MRI Weighting and Contrast | MRI Physics Course #6
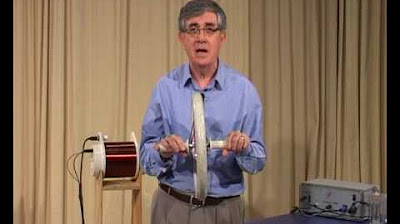
Introductory NMR & MRI: Video 01: Precession and Resonance
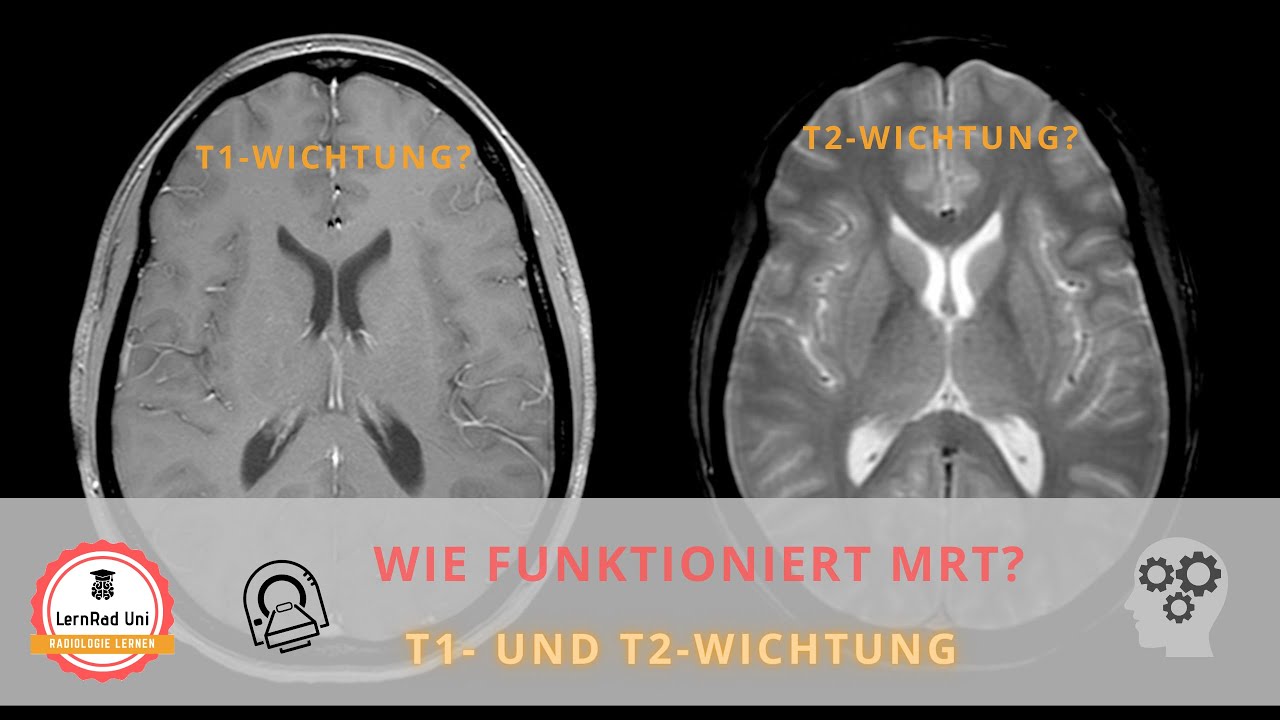
Wie funktioniert MRT? T1 und T2 Wichtung

How does an MRI machine work?
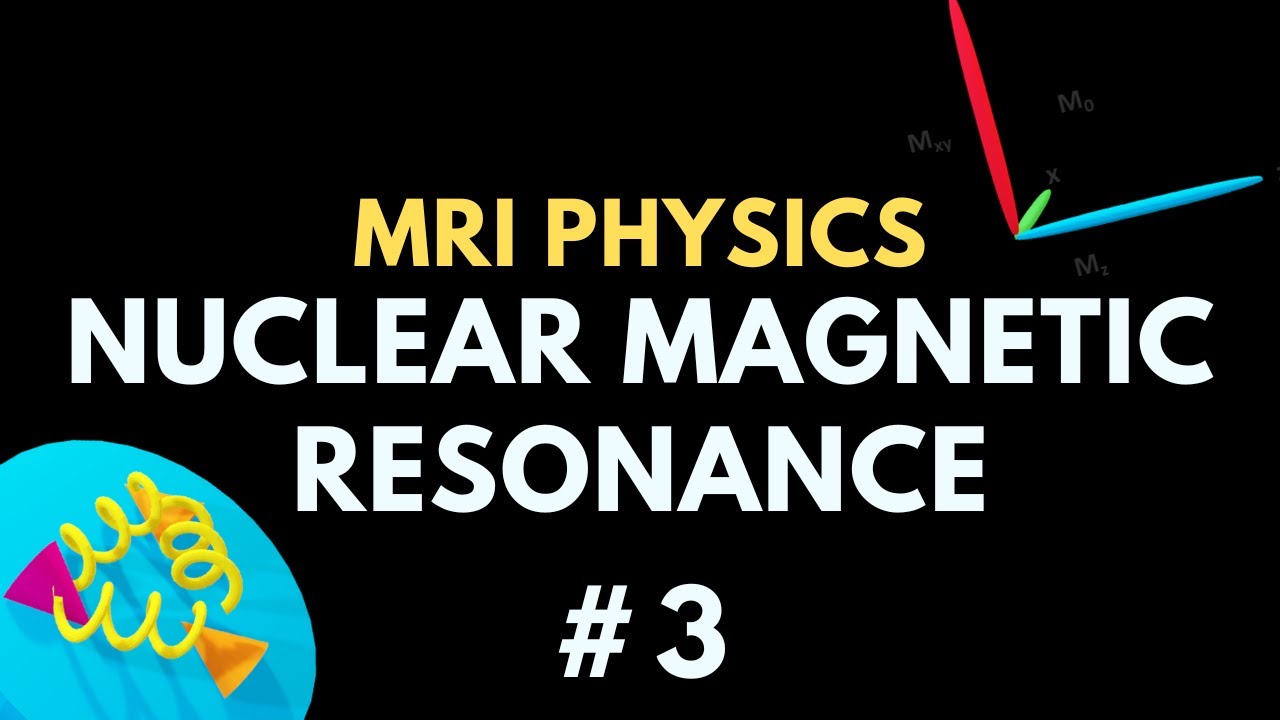
Spin, Precession, Resonance and Flip Angle | MRI Physics Course | Radiology Physics Course #3

Biomedical Instrumentation- MRI scan
5.0 / 5 (0 votes)