Tensile Stress & Strain, Compressive Stress & Shear Stress - Basic Introduction
Summary
TLDRThe video script explores fundamental concepts of mechanical stress and strain on objects. It explains tensile stress, where a downward force causes objects to stretch, and compressive stress, where an upward force leads to compression. The script delves into the formulas for calculating tensile and compressive strain, emphasizing the importance of understanding an object's ultimate strength, such as concrete's differing tensile and compressive strengths. It also introduces shear stress, its calculation, and the concept of equilibrium in both translational and rotational contexts. The elastic modulus (Young's modulus) and shear modulus are highlighted as key ratios in stress-strain relationships, crucial for predicting an object's deformation under various forces.
Takeaways
- 🔍 Tensile stress occurs when an object is hung from a ceiling and a downward force is applied, causing the object to stretch.
- 📏 Tensile strain is the positive change in length (delta l) of an object when it is stretched, calculated as delta l divided by the original length (l0).
- 🧱 Compressive stress happens when a downward force is applied to a column resting on a surface, causing it to decrease in length.
- 📉 Compressive strain is negative, as the object's length decreases, calculated as negative delta l divided by the original length (l0).
- 🏗️ Concrete's ultimate strength indicates the maximum stress it can withstand without breaking, with tensile strength being lower than compressive strength.
- 🔢 The maximum tensile and compressive strengths of concrete are given as 2 x 10^6 N/m^2 and 20 x 10^6 N/m^2, respectively.
- 📚 Tensile and compressive strengths represent the maximum stress an object can endure, calculated as maximum force divided by area.
- 📉 The elastic modulus (Young's modulus) is used to calculate the change in length (delta l) of an object under tensile or compressive stress.
- ⚖️ Shear stress is experienced when an object is deformed by forces applied in opposite horizontal directions, causing a change in shape.
- 📏 Shear strain is calculated as the horizontal change in length (delta l) divided by the original vertical length (l0).
- 🔄 Shear modulus (G) is the ratio of shear stress to shear strain, used in calculating the deformation under shear stress.
Q & A
What is tensile stress?
-Tensile stress is a type of mechanical stress that results from forces applied to an object that cause it to stretch or elongate. It is calculated as the force applied divided by the area over which the force is distributed.
How is tensile strain calculated?
-Tensile strain is calculated as the change in length (delta l) of an object divided by its original length (l0). It is a positive value when the object's length increases due to the applied force.
What is compressive stress?
-Compressive stress is the mechanical stress that results from forces applied to an object that cause it to decrease in volume. It is also calculated as the force divided by the area, but it results in a decrease in the object's length.
How is compressive strain different from tensile strain?
-Compressive strain is the change in length (delta l) of an object due to compression, which is negative because the object's length decreases. It is calculated as negative delta l divided by the original length (l0).
What is the ultimate strength of concrete?
-The ultimate strength of concrete refers to the maximum stress that the material can withstand before breaking. The maximum tensile strength of concrete is 2 x 10^6 newtons per square meter, and the maximum compressive strength is 20 x 10^6 newtons per square meter.
Why is concrete's compressive strength greater than its tensile strength?
-Concrete's compressive strength is greater than its tensile strength because the material is inherently stronger when resisting compression (being pressed together) than when resisting tension (being pulled apart). This makes concrete well-suited for structures that bear weight but not as ideal for those that experience a lot of tension.
What is the formula for calculating the change in length (delta l) under stress?
-The formula for calculating the change in length (delta l) under stress is delta l = (1 / E) * (F / A) * l0, where E is the elastic modulus or Young's modulus, F is the applied force, A is the cross-sectional area, and l0 is the original length of the object.
What is shear stress?
-Shear stress is the stress that results from forces that cause parallel layers within a material to slide against each other. It is calculated as the force applied divided by the cross-sectional area of the material.
How is shear strain calculated?
-Shear strain is calculated as the change in length (delta l) in the direction of the applied force divided by the original length (l0) in the perpendicular direction. It is a measure of the deformation caused by shear stress.
What is the shear modulus?
-The shear modulus, also known as the modulus of rigidity, is the ratio between shear stress and shear strain. It is denoted by the letter G and is used to describe a material's resistance to shear deformation.
What is the significance of torque in the context of shear stress?
-Torque is significant in the context of shear stress because it represents the rotational force that can cause an object to rotate. When an object is under shear stress, the torques acting on the object must be balanced for the object to remain in equilibrium and not rotate.
How can you ensure an object under shear stress remains in equilibrium?
-An object under shear stress can remain in equilibrium by ensuring that the net force and net torque acting on the system are both zero. This typically involves the application of additional forces that counteract the effects of the applied shear forces and torques.
Outlines
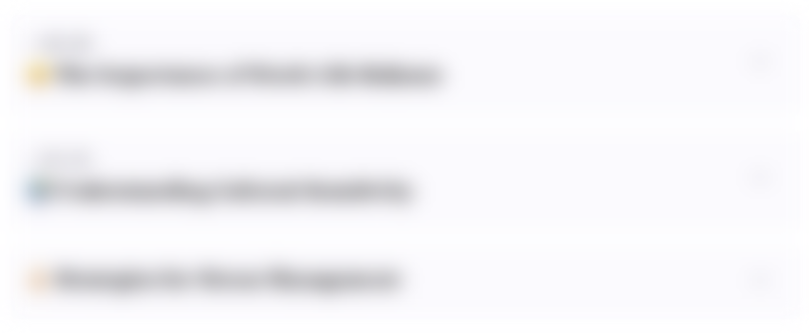
This section is available to paid users only. Please upgrade to access this part.
Upgrade NowMindmap
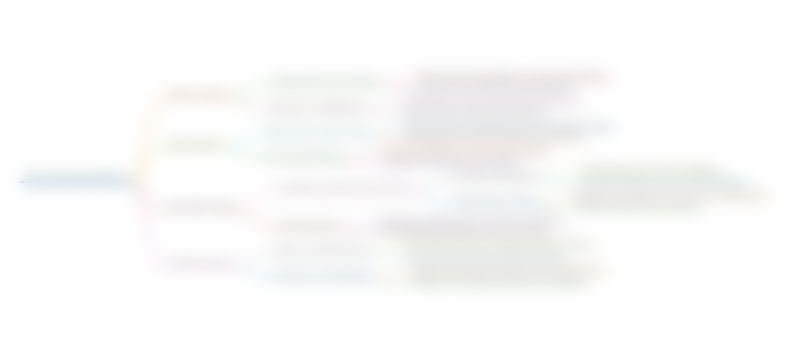
This section is available to paid users only. Please upgrade to access this part.
Upgrade NowKeywords
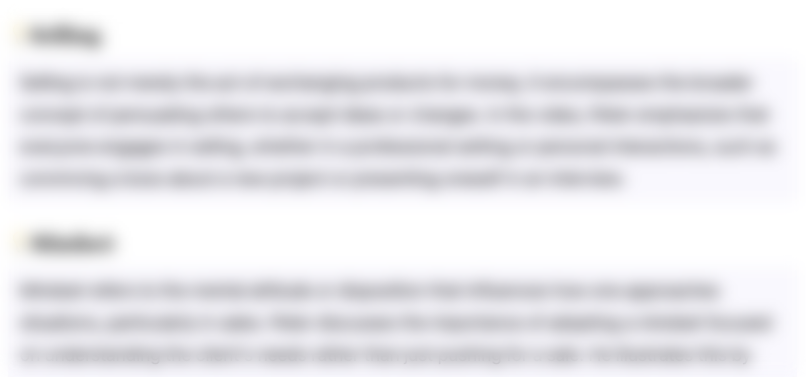
This section is available to paid users only. Please upgrade to access this part.
Upgrade NowHighlights
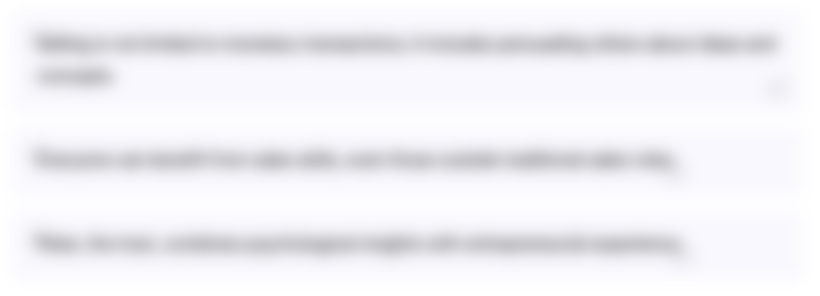
This section is available to paid users only. Please upgrade to access this part.
Upgrade NowTranscripts
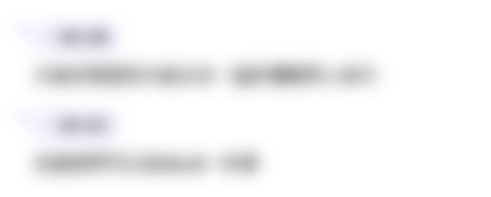
This section is available to paid users only. Please upgrade to access this part.
Upgrade NowBrowse More Related Video

Stress, Strain & Young's Modulus | A-level Physics | OCR, AQA, Edexcel

Understanding Young's Modulus
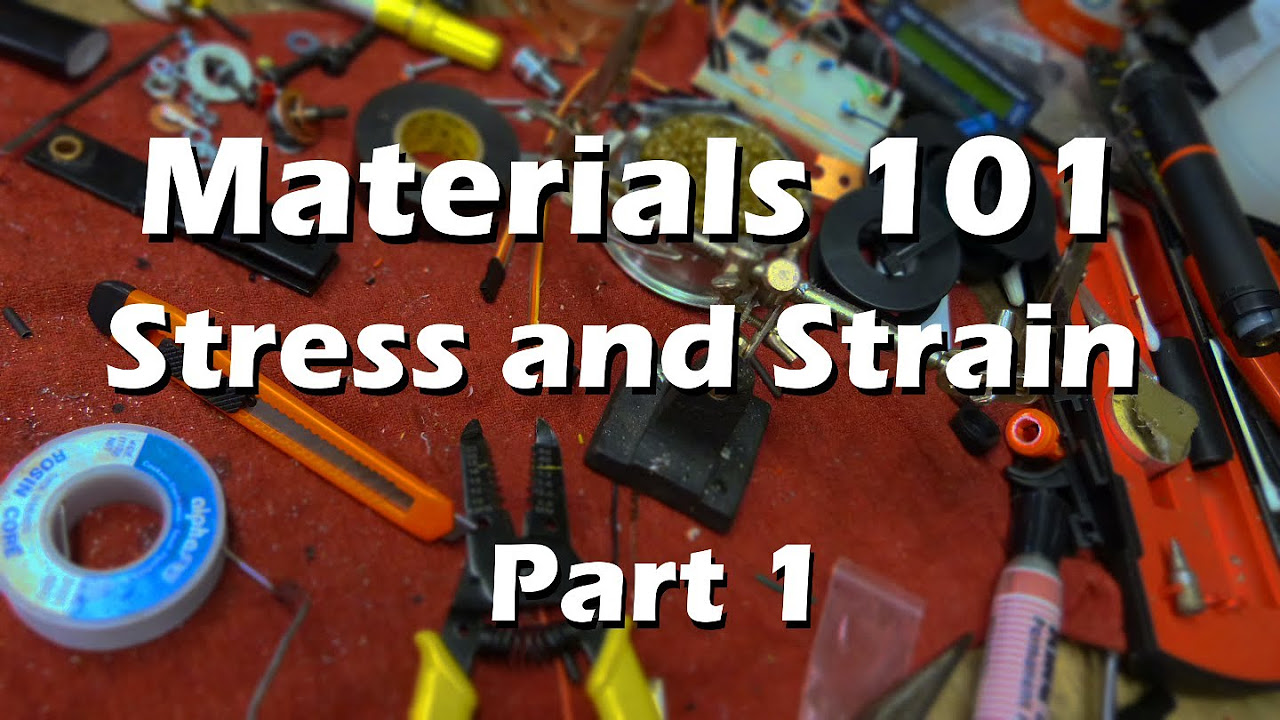
Materials Science Mechanical Engineering - Part 1 Stress and Strain Explained
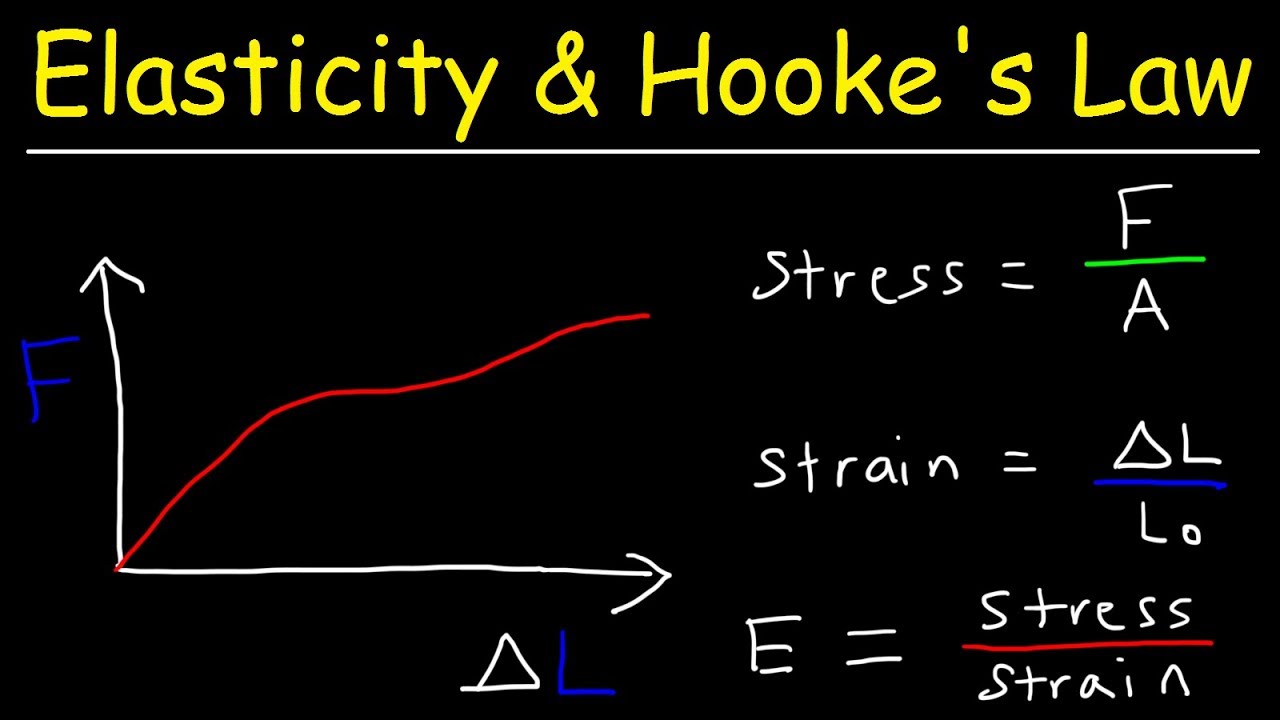
Elasticity & Hooke's Law - Intro to Young's Modulus, Stress & Strain, Elastic & Proportional Limit

Airframes & Aircraft Systems #1 - Aircraft Structures - Loads Applied to the Airframe
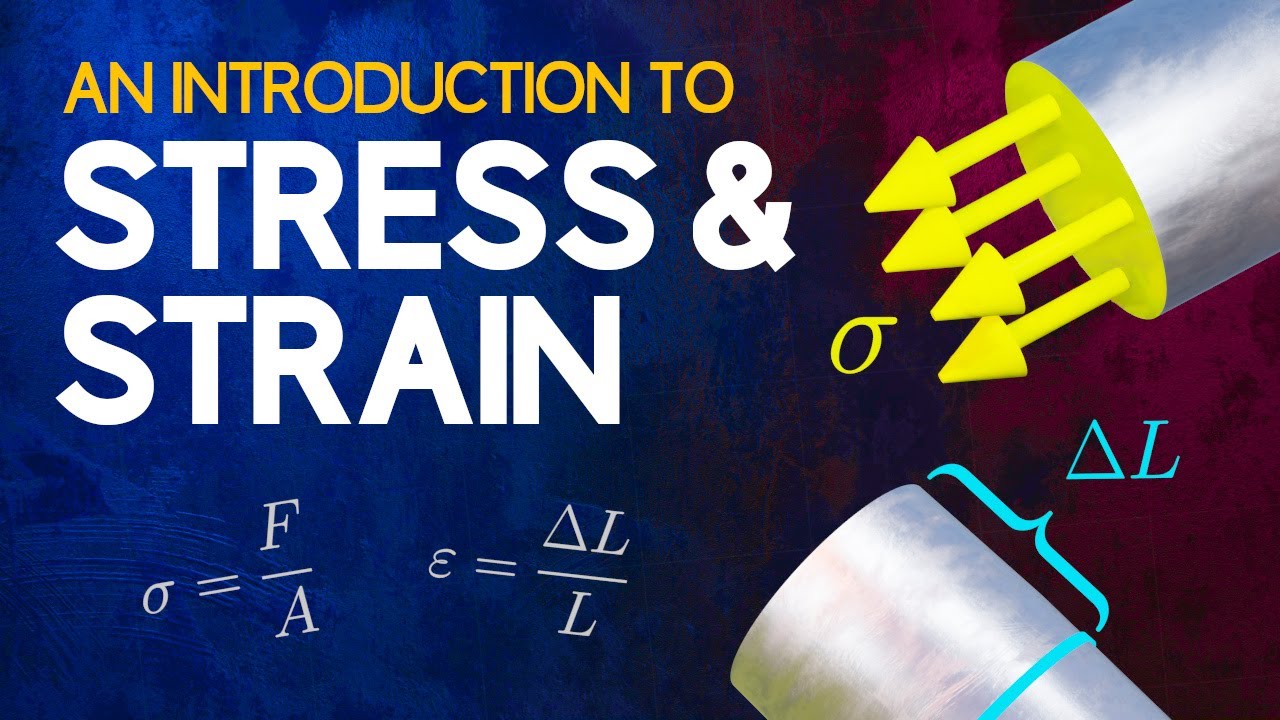
An Introduction to Stress and Strain
5.0 / 5 (0 votes)