UV/Vis spectroscopy | Spectroscopy | Organic chemistry | Khan Academy
Summary
TLDRThis script explores the principles of UV/Vis spectrophotometry, demonstrating how molecules like 1,3-Butadiene and ethanal absorb different wavelengths of light. It explains the concept of molecular orbitals, including bonding and antibonding orbitals, and how the energy difference between the highest occupied molecular orbital (HOMO) and the lowest unoccupied molecular orbital (LUMO) determines the absorbed wavelength. The script also discusses electron transitions, such as pi to pi* and n to pi*, and how they relate to a molecule's color and light absorption characteristics.
Takeaways
- 🌈 Molecules can absorb different wavelengths of light, and this can be measured using a UV/Vis spectrophotometer.
- 🔍 The spectrophotometer shines light across a wide range of wavelengths (200-800 nm) through a compound to produce an absorption spectrum.
- 🧪 For 1,3-Butadiene, the most strongly absorbed wavelength is just under 220 nm, specifically at 217 nm, which is referred to as lambda max.
- 🏞️ Butadiene is colorless because it absorbs in the UV region, which is not visible to the human eye.
- 🔬 The molecule's structure, specifically the sp2 hybridization of carbons leading to p orbitals, is crucial for understanding its molecular orbitals.
- ⚛️ Butadiene has four pi electrons that occupy the bonding molecular orbitals in its ground state.
- 🌟 When light is shone on Butadiene, a pi electron can absorb energy and be promoted from the HOMO (highest occupied molecular orbital) to the LUMO (lowest unoccupied molecular orbital).
- ⚡ The energy absorbed by the molecule corresponds to a specific wavelength of light, which can be calculated using Planck's constant and the speed of light.
- 🌟 The energy difference between molecular orbitals is inversely proportional to the wavelength of light absorbed, leading to different colors for different molecules.
- 🔬 Ethanal, another molecule discussed, has two pi electrons and can undergo both pi to pi* and n to pi* transitions, with the latter corresponding to a longer wavelength of light due to a smaller energy difference.
Q & A
What is the purpose of a UV/Vis spectrophotometer?
-A UV/Vis spectrophotometer is used to determine the wavelengths of light absorbed by a compound in the ultraviolet or visible region of the electromagnetic spectrum by shining light with a range of wavelengths through a sample of the compound and analyzing the resulting absorption spectrum.
What is the wavelength range of light that a UV/Vis spectrophotometer typically covers?
-The wavelength range of light covered by a UV/Vis spectrophotometer typically extends from approximately 200 nanometers to 800 nanometers.
What is the term for the wavelength of light absorbed most strongly by a molecule?
-The wavelength of light absorbed most strongly by a molecule is referred to as lambda max (λmax).
Why is 1,3-Butadiene colorless?
-1,3-Butadiene is colorless because it absorbs light in the UV region, specifically at a wavelength of 217 nanometers, which is not visible to the human eye.
What type of hybridization do the carbons in 1,3-Butadiene have, and what does this imply for their orbitals?
-The carbons in 1,3-Butadiene are sp2 hybridized, which means each carbon has a p orbital. This results in four atomic orbitals that combine to form four molecular orbitals, including two bonding and two antibonding orbitals.
How many pi electrons are present in 1,3-Butadiene, and where are they located in the molecular orbitals?
-1,3-Butadiene has four pi electrons, which in the ground state are located in the two bonding molecular orbitals, with two electrons in each.
What are the highest occupied molecular orbital (HOMO) and the lowest unoccupied molecular orbital (LUMO), and why are they significant in the context of light absorption?
-The HOMO is the highest energy orbital that is occupied by electrons, while the LUMO is the lowest energy orbital that is unoccupied. They are significant in light absorption because the energy difference between these two orbitals determines the specific wavelength of light that a molecule can absorb, leading to an electron transition from HOMO to LUMO.
How does the energy of a photon of light relate to its wavelength?
-The energy of a photon of light is inversely proportional to its wavelength. This relationship is described by the equation E = h * c / λ, where E is the energy, h is Planck's constant, c is the speed of light, and λ is the wavelength.
What is the significance of the energy difference between the HOMO and LUMO in terms of the molecule's absorption spectrum?
-The energy difference between the HOMO and LUMO corresponds to a specific wavelength of light that the molecule can absorb. This is observed as a peak in the absorption spectrum, which can be used to identify the molecule.
What type of electron transition occurs in ethanal, and what is its approximate wavelength?
-In ethanal, a pi to pi star transition occurs, which has an approximate wavelength of 180 nanometers. Additionally, an n to pi star transition is possible, corresponding to a longer wavelength of approximately 290 nanometers.
How does the energy difference between molecular orbitals affect the wavelength of light absorbed by a molecule?
-A smaller energy difference between molecular orbitals corresponds to a longer wavelength of light absorbed. As the energy difference decreases, the wavelength of absorbed light increases, which is related to the color that the molecule can exhibit.
Outlines
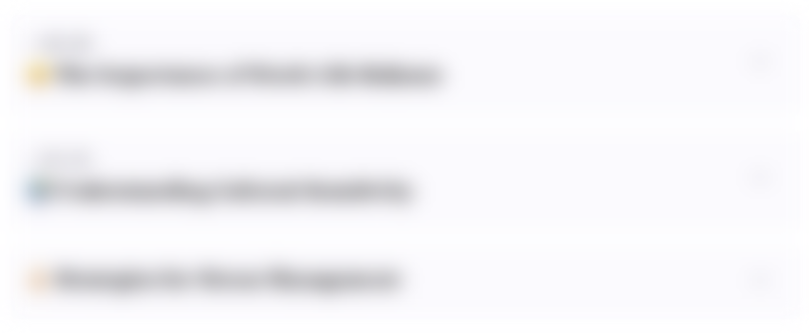
This section is available to paid users only. Please upgrade to access this part.
Upgrade NowMindmap
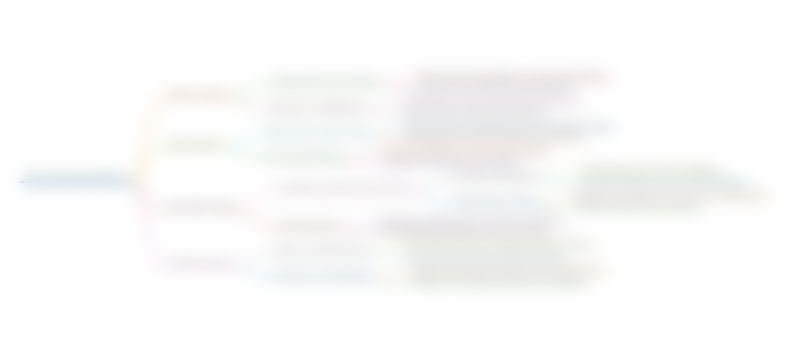
This section is available to paid users only. Please upgrade to access this part.
Upgrade NowKeywords
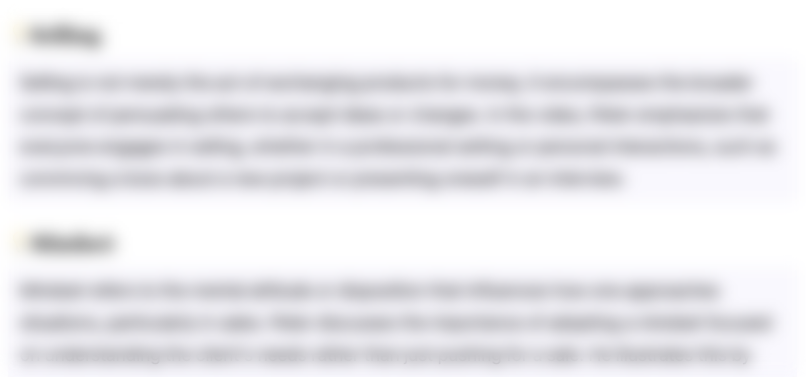
This section is available to paid users only. Please upgrade to access this part.
Upgrade NowHighlights
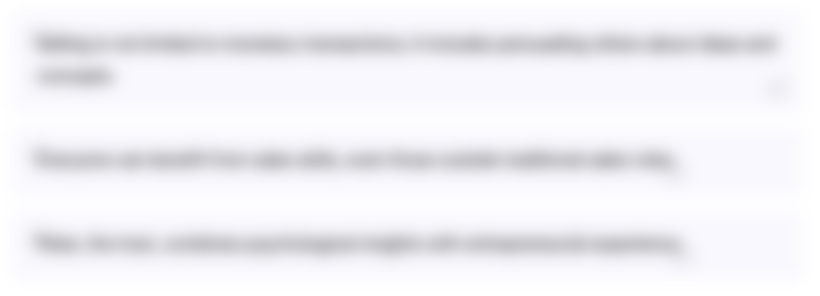
This section is available to paid users only. Please upgrade to access this part.
Upgrade NowTranscripts
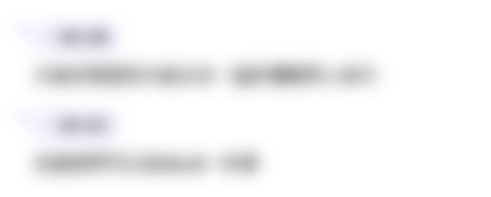
This section is available to paid users only. Please upgrade to access this part.
Upgrade NowBrowse More Related Video
5.0 / 5 (0 votes)