Kirchhoff's Loop Rule Is For The Birds
Summary
TLDREn este captivante script de video, el profesor Walter Lewin imparte un curso de física sobre electricidad y magnetismo, destacando la importancia de entender correctamente la ley de Faraday. Critica que muchos libros de física高等院校 tienen explicaciones erróneas sobre este tema, ya que los profesores no comprenden adecuadamente la ley. Lewin utiliza un circuito simple con resistores y un electromagnete para ilustrar cómo la ley de Faraday entra en juego cuando hay un cambio en el flujo magnético, lo que lleva a una EMF no nula y a una corriente en el circuito. Destaca la contradicción con la regla de Kirchhoff del circuito cerrado y cómo esto es un tema no intuitivo que puede confundir. Al final, motiva a los estudiantes a que estudien seriamente para tener éxito en su comprensión de la materia.
Takeaways
- 🧲 La lección es impartida por Walter Lewin sobre física, específicamente sobre electricidad y magnetismo, a petición de Jason Hafner, el profesor de física del estudiante.
- 🔌 Se menciona que la mayoría de los libros de física de universidad tienen un error común en la interpretación de la ley de Faraday, según Lewin, debido a que los profesores no la entienden correctamente.
- 📚 Lewin recomienda ver su conferencia número 16 y leer sus notas de la misma para comprender mejor el tema tratado.
- 🌀 Se describe un experimento con un circuito simple que incluye dos resistores y un electromagnetismo perpendicular al pizarrón, para ilustrar cómo cambia la flujo magnético y su efecto en un circuito.
- 📐 La integral de la fuerza eléctrica (E) dot producto con el elemento de longitud (dl) alrededor de un circuito cerrado no es cero cuando hay un cambio en el flujo magnético, lo que entra en conflicto con la regla de Kirchhoff para circuitos cerrados.
- ⚡️ Se introduce el concepto de Fuerza Electromotriz (EMF), que es el resultado de un cambio en el flujo magnético y que induce un corriente en el circuito.
- 🔆 La diferencia de potencial entre dos puntos en un circuito puede variar dependiendo de la dirección de la corriente y la posición de los resistores, lo que muestra la no intuición de estos fenómenos.
- 🔵 Se discute cómo el valor y la polaridad de la diferencia de potencial pueden ser diferentes en dos mediciones realizadas en dos puntos del mismo circuito, debido a la dirección de la corriente y la configuración de los resistores.
- 🔍 Se hace hincapié en la importancia de entender la ley de Faraday y cómo la mayoría de los libros de texto la presentan de manera incorrecta o engañosa.
- 🔌 Se presenta un ejemplo de un circuito con un solenoide (autoinductor) ideal, un capacitor, una resistencia y una batería, para demostrar cómo la ley de Faraday se aplica en circuitos con componentes reactivos.
- 📚 Se sugiere que los estudiantes lean las notas de la conferencia número 20 para comprender mejor los conceptos discutidos en la lección.
Q & A
¿Quién es Walter Lewin y qué propósito tiene su clase en este script?
-Walter Lewin es un profesor de física que imparte una clase con el objetivo de enseñar sobre la electricidad y el magnetismo, específicamente sobre un aspecto que considera que la mayoría de los libros de física universitaria entienden incorrectamente, a saber, la ley de Faraday.
¿Por qué dice Lewin que la mayoría de los libros de física tienen algo incorrecto sobre la ley de Faraday?
-Lewin argumenta que la mayoría de los libros de física tienen algo incorrecto sobre la ley de Faraday porque los profesores de física no la entienden adecuadamente, lo que resulta en una interpretación errónea de cómo se aplica esta ley en ciertos escenarios.
¿Qué es lo que Lewin sugiere que los estudiantes hagan para comprender mejor la ley de Faraday?
-Lewin sugiere que los estudiantes deberían ver su conferencia número 16 y leer sus notas de la misma, ya que allí cubre temas relacionados con la ley de Faraday de una manera que considera más clara y precisa.
¿Cuál es el ejemplo práctico que Lewin utiliza para demostrar un concepto de electricidad y magnetismo?
-Lewin utiliza un circuito simple con dos resistores y un electromagnetismo perpendicular al pizarrón para ilustrar cómo la ley de Faraday se aplica cuando hay un cambio en el flujo magnético y cómo esto afecta la corriente y el potencial diferencial en el circuito.
¿Qué es el 'flujo magnético' y cómo se define en el script?
-El 'flujo magnético' es una cantidad definida como el integral del producto punto entre el campo magnético B y un elemento de superficie ds sobre una superficie abierta. En el script, Lewin lo asocia con el cambio en el tiempo que afecta el circuito eléctrico.
¿Por qué Lewin insiste en que los puntos A1 y A2, así como D1 y D2, son eléctricamente idénticos?
-Lewin insiste en que los puntos A1 y A2, y D1 y D2, son eléctricamente idénticos porque están conectados a un alambre que no tiene resistencia, lo que implica que no hay diferencia de potencial entre ellos y, por lo tanto, pueden ser considerados como un solo punto desde el punto de vista eléctrico.
¿Qué implica Lewin cuando dice que 'Kirchhoff's loop rule is for the birds' y por qué?
-Lewin utiliza la expresión 'Kirchhoff's loop rule is for the birds' para criticar la aplicación indiscriminada de la regla de Kirchhoff en situaciones donde el flujo magnético cambia, ya que esta regla predice que la suma de las fuerzas electromotrices en un circuito cerrado debe ser cero, lo que no es cierto cuando hay un cambio de flujo magnético.
¿Cómo se relaciona el 'EMF' o 'Fuerza Electromotriz' con el cambio en el flujo magnético según Lewin?
-Según Lewin, cuando hay un cambio en el flujo magnético, el integral del producto punto entre la electricidad E y el elemento de longitud dl alrededor del circuito no es cero, lo que indica la presencia de una 'Fuerza Electromotriz' (EMF) que induce una corriente en el circuito.
¿Qué muestra el experimento de Lewin con el voltímetro V1 y V2 y qué conclusión se puede sacar de ello?
-El experimento de Lewin muestra que el voltímetro V1 y V2, aunque miden la diferencia de potencial entre los mismos puntos A y D, muestran valores y polaridades diferentes. Esto demuestra lo no intuitivo y contradictorio que puede ser la aplicación de principios de electricidad y magnetismo en certas situaciones.
¿Qué es un 'autoinductor' o 'solenoid' en el contexto de la clase de Lewin y cómo afecta la corriente cuando se activan?
-Un 'autoinductor' o 'solenoid' es un dispositivo que consiste en un alambre enrollado en forma de espiral y que tiene la capacidad de almacenar energía magnética. En el contexto de la clase de Lewin, el autoinductor es idealizado como un conductor supercondutor, lo que significa que no tiene resistencia interna y, por lo tanto, no puede haber un campo eléctrico dentro de él cuando la corriente comienza a fluir.
¿Cómo Lewin describe el comportamiento de la corriente y el potencial diferencial en un circuito con autoinductor, resistencia, capacitor y fuente de voltaje?
-Lewin describe que cuando se cierra un switch en un circuito de este tipo, la corriente comienza a fluir y, debido a la presencia del autoinductor, la corriente no es instantánea. El potencial diferencial a través del autoinductor no es cero y está relacionado con la tasa de cambio de la corriente con el tiempo, lo que se describe mediante la ecuación de Faraday y la ley de la autoinducción.
¿Por qué Lewin critica a los libros de física universitarios por 'hacer trampa' al escribir las ecuaciones de circuito?
-Lewin critica a los libros de física porque, a pesar de obtener resultados correctos, utilizan una forma errónea de escribir las ecuaciones de circuito que no respeta la ley de Faraday. Según él, esta forma incorrecta de presentar la física puede llevar a malentendidos conceptuales y a una comprensión incorrecta de los estudiantes.
¿Qué consejo le da Lewin a los estudiantes de la clase de Jason y a aquellos que toman el curso edX de Electricidad y Magnetismo?
-Lewin alienta a los estudiantes a tomar el curso seriamente, esforzándose en las tareas y los exámenes, y les asegura que, si lo hacen, tienen muy buenas posibilidades de pasar el curso. Les desea suerte en sus estudios.
Outlines
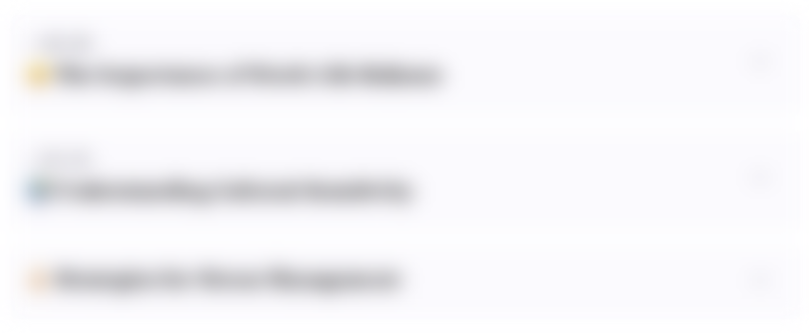
Cette section est réservée aux utilisateurs payants. Améliorez votre compte pour accéder à cette section.
Améliorer maintenantMindmap
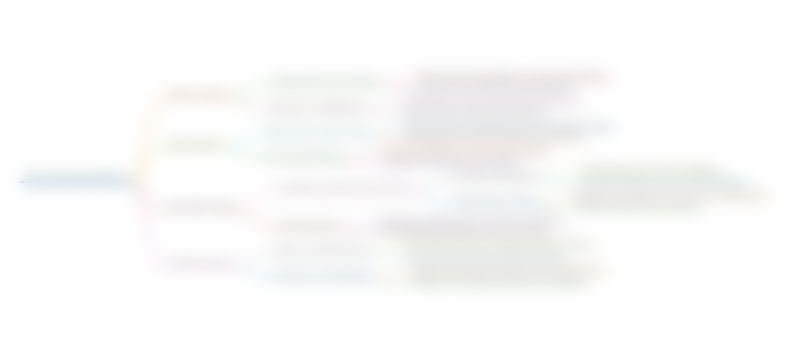
Cette section est réservée aux utilisateurs payants. Améliorez votre compte pour accéder à cette section.
Améliorer maintenantKeywords
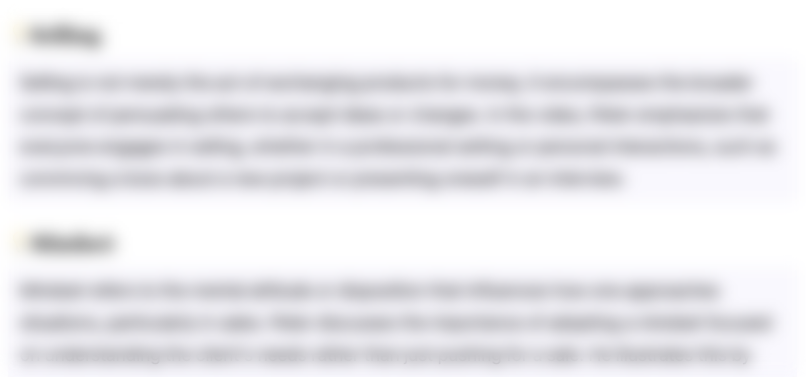
Cette section est réservée aux utilisateurs payants. Améliorez votre compte pour accéder à cette section.
Améliorer maintenantHighlights
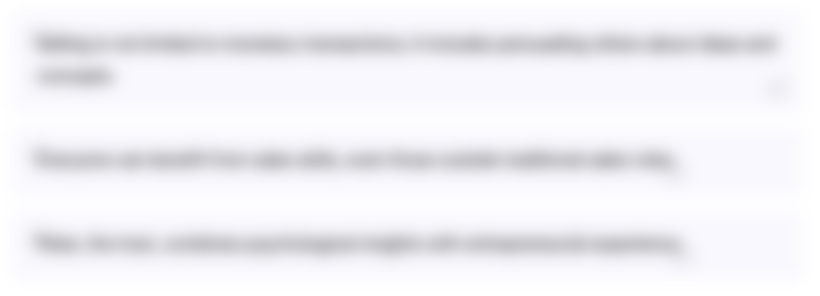
Cette section est réservée aux utilisateurs payants. Améliorez votre compte pour accéder à cette section.
Améliorer maintenantTranscripts
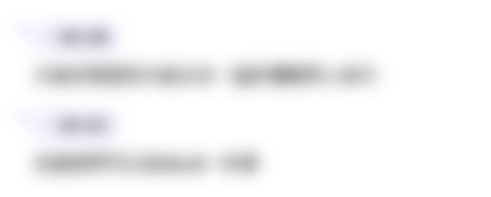
Cette section est réservée aux utilisateurs payants. Améliorez votre compte pour accéder à cette section.
Améliorer maintenantVoir Plus de Vidéos Connexes
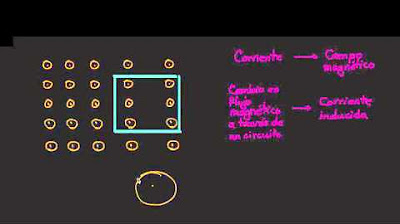
Introducción a la ley de Faraday | Física | Khan Academy en Español

¿Cómo crear electricidad con magnetismo? INDUCCIÓN Electromagnética ⚡ Ley de Faraday y Lenz
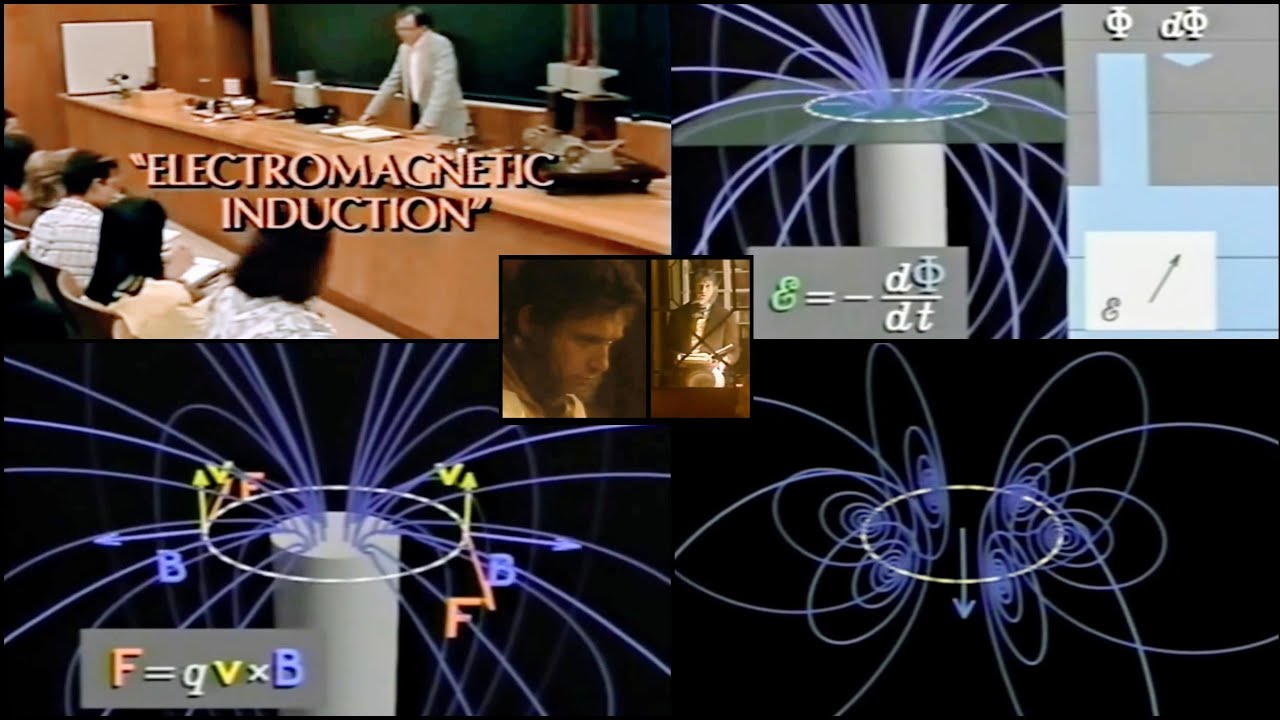
Inducción Electromagnética (Universo Mecánico 37)
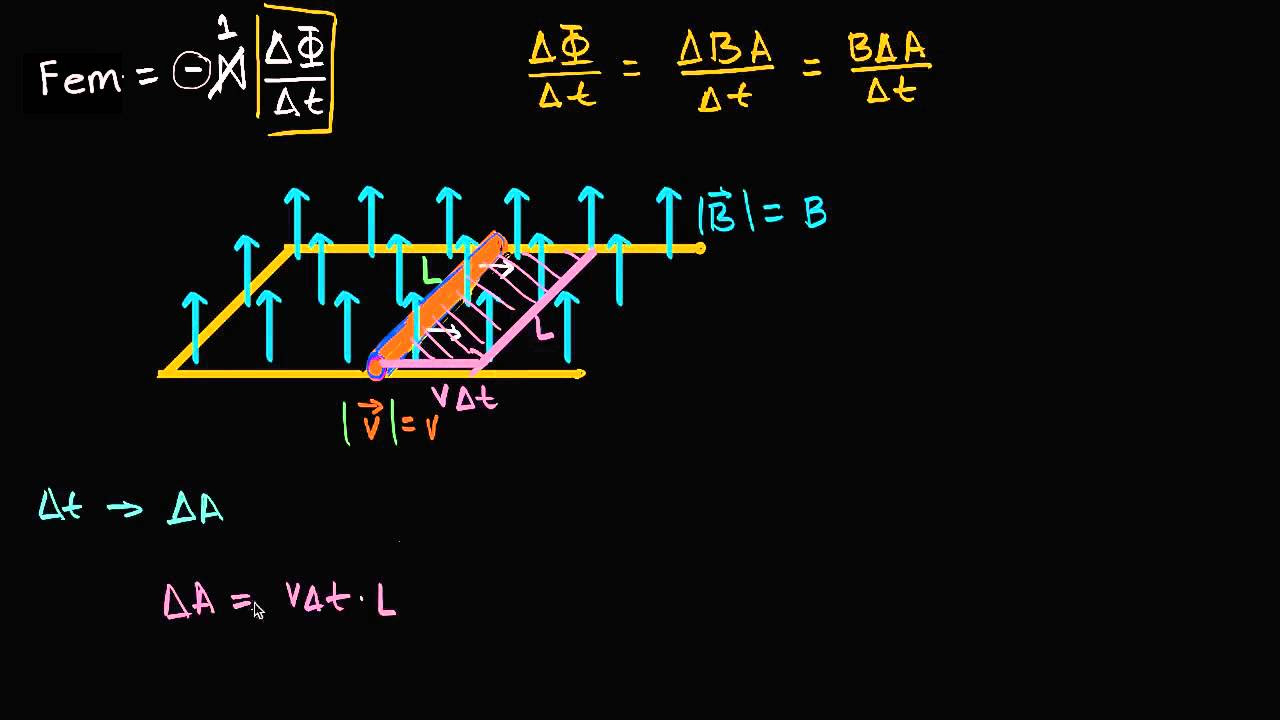
FEM inducida en una barra que se mueve a través de un campo magnético | Khan Academy en Español

Principio del Generador Eléctrico
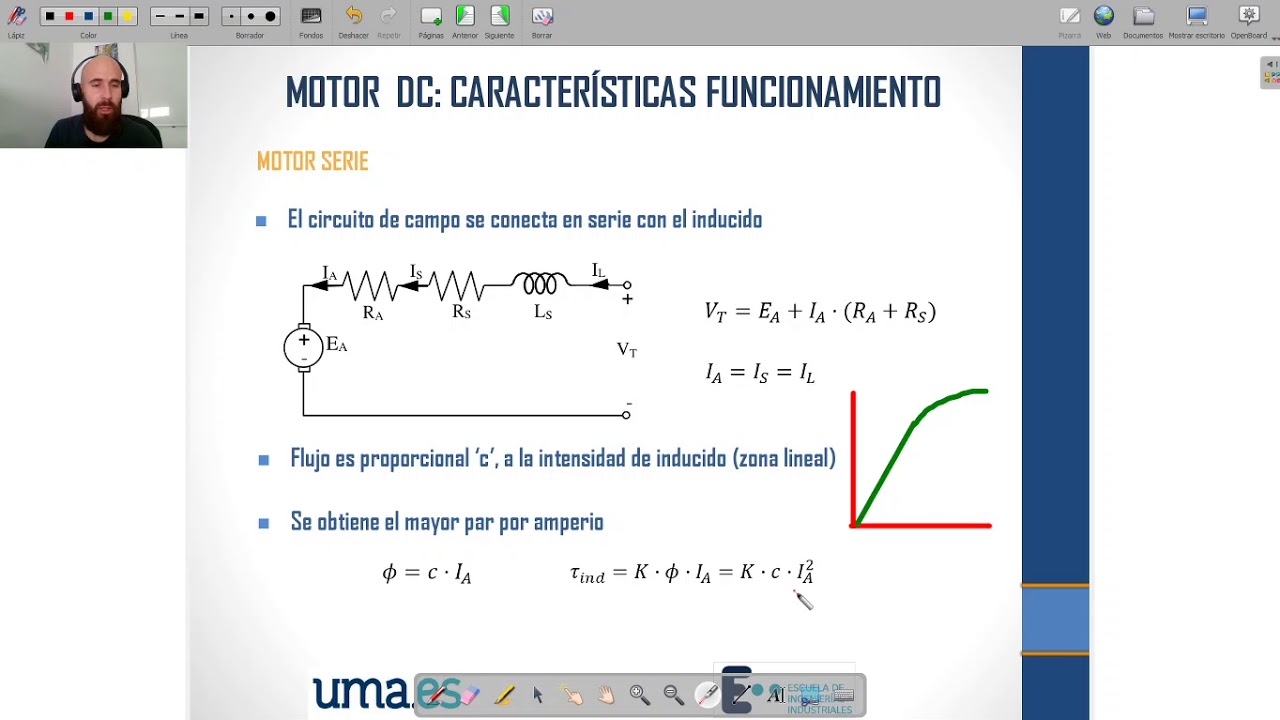
MOTOR DC SERIE
5.0 / 5 (0 votes)