Synthetic Biology: Metabolic Engineering and Synthetic Biology of Yeast - Jens Nielsen
Summary
TLDRJens Nielsen presenta una charla sobre ingeniería metabólica y biología sintética en la levadura, destacando su uso en la producción de productos tradicionales y farmacéuticos. Explica el concepto de fábricas celulares y su importancia en la biofarmacéutica, con ventas que superan los 100 mil millones de dólares. Aborda el reto de redirigir el metabolismo de la levadura para producir nuevos compuestos, como biocombustibles y productos químicos, utilizando herramientas de la ingeniería metabólica y biología sintética para mejorar la producción industrial.
Takeaways
- 🚀 La ingeniería metabólica y la biología sintética de la levadura son temas centrales del discurso de Jens Nielsen.
- 🏭 Las 'fábricas de células', como la levadura, se utilizan tradicionalmente en la producción de alimentos y farmacos, y más recientemente en biocombustibles y productos químicos.
- 💊 La producción de biofármacos supera los 300 y tiene ventas que exceden los 100 mil millones de dólares estadounidenses.
- 🌱 El concepto de biorefinería implica la conversión de biomasa en azúcares, los cuales son fermentados por microorganismos para producir combustibles y productos químicos.
- 🔬 La ingeniería metabólica es esencial para redirigir el metabolismo de las levaduras para producir diferentes tipos de combustibles y productos químicos.
- 🛠️ Se necesitan herramientas de ingeniería metabólica y biología sintética para entender y manipular el metabolismo de las levaduras y mejorar la producción de compuestos de interés.
- 🔄 El ciclo de ingeniería metabólica, también conocido como ciclo de prueba, construcción y diseño, es un proceso iterativo para desarrollar cepas industriales viables.
- 🌡️ La adaptación de las levaduras para crecer a temperaturas más altas puede reducir los costos de enfriamiento y mejorar la estabilidad del proceso en la industria del bioetanol.
- 🔬 La biología de sistemas y la biología sintética son áreas clave que contribuyen al desarrollo de nuevas herramientas para la ingeniería metabólica.
- 📈 La optimización de la producción de precursores como el acil-CoA puede aumentar significativamente la producción de compuestos de interés en las levaduras.
- 🔑 Las mutaciones identificadas en las levaduras adaptadas a temperaturas más altas, como la duplicación del cromosoma y las mutaciones puntuales en genes como ERG3, pueden ser claves para mejorar la producción y adaptabilidad de las levaduras.
Q & A
¿Qué es la ingeniería metabólica y cómo se relaciona con la biología sintética en la producción de levaduras?
-La ingeniería metabólica es el proceso de manipular el metabolismo de una célula para cambiar su función o para producir ciertos compuestos. Se relaciona estrechamente con la biología sintética, especialmente en el diseño e implementación de nuevas 'fábricas celulares' y sus propiedades, proporcionando herramientas para el diseño y construcción de levaduras que pueden producir una variedad de productos industriales.
¿Cuáles son algunos ejemplos de productos tradicionales que se producen usando 'fábricas celulares' como las levaduras?
-Algunos ejemplos de productos tradicionales que se producen con 'fábricas celulares' incluyen la cerveza, el vino, el pan y el yogur. Además, se utilizan también para la producción de farmacéuticos como antibióticos, hormonas y fármacos antineoplásicos.
¿Cómo se está utilizando recientemente a las 'fábricas celulares' para la producción de combustibles y productos químicos?
-En las 'fábricas celulares', se están utilizando bioetanol como un ejemplo clásico, así como ácido cítrico para bebidas, y 1,3 propanediol que se usa en la producción de polímeros, por ejemplo en alfombras y otros tipos de tejidos.
¿Qué es el concepto de 'biorefinería' y cómo se relaciona con la producción de productos sostenibles?
-El concepto de 'biorefinería' se refiere a la producción de productos químicos y combustibles a partir de materias primas vegetales. Se integra en una sociedad sostenible global, donde también se utilizan fuentes de energía sostenibles como la solar, el viento y otros tipos de energías.
¿Cómo se describe el proceso de ingeniería metabólica para cambiar el metabolismo de una levadura y aumentar la producción de un producto específico?
-El proceso de ingeniería metabólica implica manipular el metabolismo de la levadura para que produzca más de un producto específico y menos de otros, como el etanol. Esto puede implicar la inserción de un nuevo camino metabólico, tomando genes de otro organismo, para producir el producto deseado.
¿Cuál es el desafío principal al tratar de incrementar la producción de un producto en una levadura a través de la ingeniería metabólica?
-El desafío principal es redirigir o reconfigurar el metabolismo de tal manera que la levadura comience a producir más del biodiesel y reducir la producción de etanol. Esto requiere una comprensión profunda del metabolismo de la levadura para poder realizar dichas modificaciones.
¿Qué es el ciclo de ingeniería metabólica y cómo ayuda en el desarrollo de nuevas 'fábricas celulares'?
-El ciclo de ingeniería metabólica, a menudo llamado ciclo de prueba, construcción y diseño, es un proceso iterativo que comienza con criterios de diseño, implementación en una cepa, caracterización en un proceso de fermentación y diseño de un proceso con condiciones industriales, lo que permite utilizar herramientas de biología de sistemas para mejorar el diseño.
¿Por qué es la levadura una opción atractiva para usar como una 'fábrica celular'?
-La levadura es atractiva debido a su bien caracterización, su trazabilidad genética, su consideración general como segura, lo que significa que los productos producidos en levaduras son relativamente fáciles de introducir en el mercado, y su robustez, lo que la hace fácil de implementar en entornos industriales.
¿Cómo se aborda el problema de la complejidad y diversidad del metabolismo en la ingeniería metabólica?
-Para abordar la complejidad del metabolismo, se utilizan una serie de herramientas diferentes, incluyendo la ingeniería metabólica cíclica y las herramientas de la biología de sistemas, para entender, diseñar e implementar modificaciones en el metabolismo de la levadura.
¿Qué es la evolución adaptativa y cómo se utilizó en el estudio para mejorar el crecimiento de las levaduras a temperaturas más altas?
-La evolución adaptativa es el proceso por el cual las células se adaptan a condiciones ambientales específicas. En el estudio, se cultivaron levaduras a 40 grados Celsius durante muchas generaciones, lo que resultó en clones que podían crecer mejor a esa temperatura elevada, lo que se atribuyó a la acumulación de mutaciones puntuales en varios genes, incluido ERG3.
¿Cómo se relaciona el cambio en la composición esteroidea de las levaduras con su capacidad para crecer a temperaturas más altas?
-El cambio en la composición esteroidea, específicamente la acumulación de fucosterol en lugar de ergosterol, se ha correlacionado con la capacidad de las levaduras para crecer a temperaturas más altas. Una mutación puntual en la gena ERG3 fue identificada como la causa de este cambio en la composición esteroidea.
Outlines
🌱 Ingeniería metabólica y biología sintética de la levadura
Jens Nielsen presenta la ingeniería metabólica y la biología sintética de la levadura, destacando su importancia en la producción de productos tradicionales y farmacéuticos. Se menciona el crecimiento de la industria de biofármacos, que supera los 300 productos con ventas de más de 100 mil millones de dólares. También se discute el uso de las células como fábricas para la producción de combustibles y compuestos químicos, como el bioetanol, el ácido cítrico y el 1,3 propandiol. El concepto de biorefinería se introduce como una forma sostenible de producir químicos y combustibles a partir de biomasa, integrándose en una sociedad global sostenible que utiliza energías renovables.
🔬 Desarrollo y costos de las fábricas celulares
Se analiza el proceso de desarrollo de las fábricas celulares, enfocándose en la ingeniería metabólica para cambiar la metabolización de las células y aumentar la producción de nuevos compuestos. Se destaca la importancia de las herramientas de ingeniería metabólica y biología sintética para entender y manipular la metabolismo de las células. Se menciona el costo de desarrollo de una fábrica celular, que puede alcanzar los 50 millones de dólares, y la tendencia hacia asociaciones entre empresas para compartir riesgos e inversiones.
🛠️ Herramientas para la ingeniería metabólica
Nielsen describe el ciclo de ingeniería metabólica, que incluye diseño, construcción, caracterización y optimización de las células. Se resalta la interacción entre la biología sintética y la ingeniería metabólica, y cómo las herramientas de la biología sintética, especialmente la edición génica, han impactado significativamente en el campo. Además, se discuten las ventajas de utilizar la levadura como plataforma para la producción de una amplia gama de productos, destacando su caracterización, trazabilidad genética y robustez para el uso industrial.
🌐 Producción de compuestos a partir de acetyl-CoA
Se presentan varios ejemplos de productos derivados de acetyl-CoA, como el ácido 3-hidroxipropionico, el ácido succínico, el polihidroxibutirato y los isoprenoides, que tienen aplicaciones en diversos campos, incluyendo el plástico biodegradable y los ingredientes para perfumes. Se describe cómo, a través de la ingeniería metabólica, se logró aumentar significativamente la producción de estos compuestos, optimizando la ruta metabólica que conduce a acetyl-CoA.
🔄 Optimización de la producción de biodiesel y otros compuestos
Nielsen habla sobre el trabajo en la optimización de la producción de biodiesel a través de la ingeniería de la levadura central, lo que resultó en un aumento en la producción de esteres de ácidos grasos por más de 5 veces. También se menciona el interés en la producción de alquenos, que son compatibles con la infraestructura actual, y la conversión de ácidos grasos en alquenos dentro de la célula. Además, se discuten otros productos como el resveratrol, la insulina humana, la hemoglobina humana y la adaptación de la levadura para crecer a temperaturas más altas, lo que tiene implicaciones para la industria de bioetanol.
🔬 Evolución adaptativa y genética de la levadura
Se describe un enfoque diferente para mejorar la capacidad de la levadura para crecer a temperaturas más altas a través de la evolución adaptativa en laboratorio. Después de generaciones de cultivo a 40 grados Celsius, se aislaron clones con un mejor rendimiento a temperaturas elevadas. Se realizó secuenciación del genoma para identificar las mutaciones que contribuyeron a esta adaptabilidad, identificando una duplicación en el cromosoma 3 y un punto mutation en el gen ERG3, que resultó en una alteración en la composición esterolica de la levadura y permitió su crecimiento a temperaturas más altas.
Mindmap
Keywords
💡Metabolic Engineering
💡Sintética Biología
💡Células Factoría
💡Biorefinería
💡Bioetanol
💡1,3 Propanediol
💡Acetil-CoA
💡Adaptativa Laboratorio Evolución
💡ERG3
💡Fucosterol
Highlights
Metabolic engineering and synthetic biology are key in the development of yeast as cell factories for various products, including traditional and pharmaceutical products.
Biopharmaceuticals produced by cell factories have sales exceeding $100 billion, indicating a significant market impact.
The biorefinery concept utilizes plant feedstocks for the production of chemicals and fuels, integrating with sustainable energy sources.
Cell factories can produce liquid transportation fuels and biochemicals, playing a crucial role in a sustainable society.
Metabolic engineering involves redirecting yeast metabolism to produce different fuels and chemicals by modifying its natural ethanol production pathways.
The development of cell factories is research-intensive, with costs typically around $50 million U.S. dollars.
Partnerships between companies are becoming common to share risks and capital investments in cell factory development.
Yeast cells are attractive as cell factories due to their well-characterized nature, genetic tractability, and robustness for industrial settings.
Synthetic biology tools, particularly in genome editing, have significantly impacted metabolic engineering.
The production of 3-hydroxypropionic acid, a precursor for super absorbent polymers, was improved through metabolic engineering of yeast.
Succinic acid, a potential de-icing agent and biopolymer feedstock, saw an 80-fold increase in production through metabolic and evolutionary engineering.
Polyhydroxybutyrate, a biodegradable polymer, can be produced directly in yeast through metabolic engineering, with an 80-fold increase in production.
Isoprenoids, including sesquiterpenes for perfume ingredients, can be produced in yeast with significant improvements through pathway optimization.
N-Butanol, a chemical building block and alternative biofuel, saw a 5 to 6-fold increase in yeast production through metabolic engineering.
Biodiesel production in yeast through the expression of enzymes for fatty acid ethyl ester synthesis, compatible with current biodiesel standards.
Alkanes, fully compatible with current diesel infrastructure, can be produced in yeast through the conversion of fatty acyl-CoAs.
Resveratrol, an antioxidant with health benefits, saw significant production improvements in yeast through metabolic pathway optimization.
Human insulin production in yeast was optimized, reaching titers close to industrial scale production levels.
Human hemoglobin, a potential blood substitute, saw a 3-fold increase in yeast cell accumulation through metabolic engineering.
Adaptive laboratory evolution has enabled yeast to grow at higher temperatures, reducing cooling costs and improving process stability.
A single point mutation in the ERG3 gene in yeast resulted in a shift in sterol composition, allowing for growth at elevated temperatures.
The ability to grow yeast at higher temperatures has a significant impact on bioethanol production efficiency.
Transcripts
So, my name is Jens Nielsen and I'm
going to talk to you today about metabolic
engineering and synthetic biology of yeast.
And to start off, let me put into context
and tell a little bit about cell factories and their
use. So this is a quite old concept that we are using
cell factories in the production of beer, wine, bread,
and yogurt, as traditional products. But also
a number of pharmaceuticals are being produced using
cell factories, antibiotics, hormones, and anti-cancer drugs.
And if we look into biopharmaceuticals, to date they
produce more than 300 of these. And they have sales exceeding
$100 billion U.S. dollars. But more recently, also there's been
more focus on using cell factories for the production of fuels
and chemicals. Bioethanol is a classical example.
But also citric acid going into soft drinks, and more recently
1,3 propanediol, which is used for polymer production
that goes for example into carpets and other types of fabrics.
If we look at this biorefinery concept, which is very
much about production of chemicals and fuels, the idea
is to use plant feedstocks as a feedstock for the synthesis
and production of chemicals and fuels. And of course,
this has to go and be integrated in global sustainable
society, where we also use solar, wind, and other types of
sustainable energy sources. But particularly, the cell factories
can provide liquid transportation fuels, but also different
types of biochemicals. So in the biorefinery concept,
what you do is you have biomass that you are converting
-- can come from different sources, you convert to sugars
and then the sugars are used in a microbial fermentation,
where you then produce the fuels and the chemicals here.
And what is characteristic in the bioreactor is, you have
a cell factory here that you use for this bioconversion
process. And what we normally do is, we do metabolic
engineering of this cell factory in order for it to change its
metabolism such that it can produce these different fuels
and chemicals. If we look a little bit about the value chain
in the conversion process. So again, we have up here
the pre-treatment, the fermentation, then you come
to purification, and formulation, the traditional processes
in the chemical industry. But what is characteristic here is
that the development of the cell factory that is used
in the biotransformation is really the research intensive
part. And total cost for developing a cell factory is
typically in the range of $50 billion U.S. dollars --
sorry, $50 million U.S. dollars. And there's a trend
therefore a trend towards using partnerships where
companies are sharing risk and capital investments.
And so, some typical examples are illustrated here,
BP and Dupont partnered up to make isobutanol,
Total and Amyris partnered up on making farnesane,
that can be used as jet fuels and for heavy trucks,
but also 1,4 butanediol, a partnership between
genomatic and BSF for production of 1,4 butanediol,
which is used for the production of spandex and other
types of polymers. So, briefly, an introduction about
metabolic engineering. So, yeast cells naturally produce
ethanol and CO2 and more yeast cells, as illustrated here.
And these are of course the traits that are used in the
production of beer, bread, and wine, but also bioethanol
production. And so, what do we do when we want to use
this yeast cell for production of other chemicals is we
take use of this very efficient glycolytic pathway that yeast has
in the conversion of glucose to ethanol. But we want to tap
off the stream here and insert, typically a pathway by taking
genes from another organism, it can be from a bacteria,
or it can be from plant cells or whatever. What kind of
product we want to produce. Well, let me just illustrate it here.
Let's say we want to produce a compound that can be used
as a biodiesel, we would tap it off from the main glycolytic
pathway here, and insert this pathway here, and we will produce
some of this secreted by the cells. But as you can see,
a very small amount of this is produced when we first insert
this pathway in yeast, because yeast has evolved of course
to be efficiently converting the glucose into ethanol.
So the challenge we're facing is to redirect or rewire the
metabolism such that we begin to produce more of the biodiesel
and basically reduce the production of ethanol. And this is
basically the essence of metabolic engineering, where we need to
understand and get insight into the yeast metabolism, in order
to do this. So therefore, we also often see that it's quite easy
to make a proof of principle strain here. But what is much
more difficult is to make a final strain here that meets certain
requirements in titer yield and productivity over here.
And this final strain here, we have to obtain in order to
have an economically viable process. And so the development
from this proof of principle strain here to this final strain
here is what is costly. It takes time, 3-6 years, and several
person -- hundreds of person years. So, what is really what we're
looking for is enabling technologies, novel technologies that can
reduce this development time such that we can bring new products
and new technologies onto the market faster.
So, but if we look at the challenge on this. So if we look at a
yeast cell, it has about 1500 metabolic reactions. So these
are associated with about 900 genes. If we take a human cell,
it's more, it's about 8000 metabolic reactions associated with
3000 reactions. So metabolism is quite complex and diverse.
And so, in order to study and get insight into this, and particularly
engineer it, we need a number of different tools at hand.
So that's why we often talk about the metabolic engineering
cycle, or also often called the test, build, design cycle.
So, we often would start with some kind of design criteria
here, we would implement these in a strain by strain construction,
we would then characterize this in a fermentation process here,
we would try to design a process that has industrial-like conditions,
and we can then use a number of tools from systems biology
to characterize and phenotype the strain, and that can then be used
for further design. And so we often have this cyclic operation in
development. And we may have to go through this cycle many
times, in order to develop the strain to become applicable
for an industrial based process. This part over here we normally
call systems biology, so this is here, we have gained a lot of
experience and knowledge from the development of systems biology.
And the strain construction is often also referred to as synthetic
biology, and particularly, many tools in genome editing coming from
the synthetic biology field have impacted metabolic engineering
significantly. So, synthetic biology is a broad discipline but it
interacts closely with metabolic engineering in certain applications.
And particularly in the design and implementation of novel cell factories
and their properties. Synthetic biology has provided a number of
new tools, as we discussed recently in this commentary here
on the emergence and the interactions between synthetic biology
and metabolic engineering. So if we look at yeast as a
cell factory, why is that attractive to use? Well, first of all,
there are some advantages. It's extremely well-characterized,
we have a lot of information, it's genetically tractable,
it's what is characterized as generally regarded as safe,
that means the products produced in yeast are relatively
easy to get on the market, and it's also very robust,
so that means it is very easy to implement for industrial settings.
And so this is also why it's already used for production of
a number of different products. Bioethanol, but also
many higher value products, including also pharmaceutical
proteins. And there's also a number of ongoing development
of yeast cell factories for production of fuels and commodity
chemicals and so on. So yeast is to a large extent, one of the
preferred cell factories in industry and these are some of the
reasons for that. So, in my lab, we're therefore working on
developing yeast as a platform organism to produce a range
of different chemicals here. They range from biofuels to commodity
chemicals, and fine chemicals, and proteins. And in the
process here, we are using and developing also new synthetic
biology tools, as well as systems biology tools to phenotype
the yeast strains here. And I'm going to give a couple of
examples on production of some of these compounds listed
here to illustrate these principles here.
So, one general comment first is that often when we are going to
produce different types of products, as illustrated here, it can
be 1-butanol, it can be isoprenoids derived from plants,
for example, sterols, polyketides, polyphenols, alkanes,
alkenes, we often see that they are derived from the same
pathway intermediate, in this case here, acetyl-CoA,
which is a key central metabolite in metabolism.
And so, often the challenge in actually overproducing
and efficiently producing all of these products here boils down
to having an efficient conversion of glucose here down to acetyl-CoA.
And so, here we are challenged in yeast by the fact that acetyl-CoA
is in different compartments, it's in the nucleus, it's in the peroxisome,
it's also in the mitochondria, as illustrated here. So, what we need
to do is if we want to implement these pathways in the cytosol,
we need to make sure that the glucose is converted efficiently
to acetyl-CoA here. And this is also why we talk about platform
cell factories, because if we have an efficient cell factory for
conversion of glucose to acetyl-CoA, we can use that cell factory
for production of a range of different products here. Where we insert
the corresponding pathways. So, let me give a couple of examples
of acetyl-CoA derived products. The first one is 3-hydroxypropionic
acid, this can be derived in a simple two step pathway, acetyl CoA
goes to malonyl-CoA that then goes further to 3-hydroxypropionic
acid. This chemical is interesting because it can be converted into
acrylates, which are used as super absorbent polymers, for example
diapers, but find a number of different applications as a polymer.
The market is quite large, as illustrated here, and there are several
companies that are interested in producing this. So we worked
on engineering expression, first of all, the enzyme for malonyl-CoA
to 3-hydroxypropionic acid, but then we demonstrated that
by optimizing the production of acetyl-CoA, the precursor
up here, we could increase significantly the production of
3-HP, as illustrated here. So this was about a 10-fold improvement
that was obtained by providing more efficiently the precursor
upstream of this pathway here. We also worked on another
that is also used as a feedstock for production of polymers.
This is succinic acid, there's also a lot of interest in this
in the field today. Succinic acid can be used for de-icing of
airplanes, but can also be used as a feedstock for production
of biopolymers like spandex. And so what we did was we
did metabolic engineering of yeast as well as combining
this with adaptive laboratory evolution and with this process
we see an improved production of succinic acid by 80-fold.
Another product -- the opportunity to produce the polymers directly
in yeast, so what we did here was we took a pathway from
bacteria and expressed it in yeast to produce this
polyhydroxybutyrate, which is a biodegradable polymer.
There's much interest in using that, Coca Cola is introducing
this for their Coke bottles. And what we did was, that again
illustrated here, by insertion of the pathway in yeast,
we produce relatively low levels, as seen over there on the reference.
But when we then engineered again this acetyl-CoA supply
we can boost the production 80-fold inside the cell.
We begin to see accumulation here, as the white dot illustrated here,
of this polymer inside the cell. Another class of compounds
that there's much interest in is isoprenoids, and particularly,
one subclass of these, sesquiterpenes. Some of these
can find a number of applications. Here is listed
four compounds that are of interest to be used for perfumes
or in perfume ingredients, and this is work we did in collaboration
with a Swiss company, Firmenich. And so what we did was
we inserted the plant genes for production of these
pathways -- of these metabolites in yeast. And at
the same time, we worked on up-engineering of the upstream
metabolism, such that we could efficiently produce these
different perfume ingredients. And here I'm just going to illustrate
briefly, for santalene. So by expression of the santalene
synthase directly in yeast, as you can see over there,
that we have a very low level of production. But by engineering
the upstream pathway by targeting a number of different
steps, but also optimization of the fermentation process,
we could more than 10-fold improve this and actually reach
a titer that was sufficient to push this forward for beginning to
have commercial production. Finally, we -- to mention also,
we also worked on another pathway derived from acetyl-CoA,
n-Butanol production. So here, you can see we took a pathway
from bacteria again. And yeast naturally does not produce
1-butanol, so we expressed these enzymes in yeast.
It gives initially very low level production, but again through
engineering of the acetyl-CoA metabolism, we could increase
the production about 5 to 6-fold. And butanol is interesting to
be used both as a chemical building block, but it can also be used
as an alternative biofuel. Biodiesel, there's also much interest in
producing biodiesel. We've taken different approaches to this
One is to produce what is very similar to what is used in
biodiesels today, these are esters. So what you do today is
that you extract plant oils and you do a re-esterification,
as illustrated up here, with methanol typically, so you produce
fatty acid methyl esters. We expressed enzymes in yeast
so we can take fatty acids, fatty acyl-CoAs, esterize them
with ethanol here, that yeast naturally produce, and then produce
the fatty acid ethyl ester that is completely compatible
with the current biodiesel used. And again, we illustrated here
by engineering, yeast central metabolism here, we could
increase the production of these fatty acyl ethyl esters
by more than 5-fold. What there's really interest in is
to produce alkanes, because these are completely compatible
with the current infrastructures. So what we have also been
working on is to convert the fatty acyl-CoAs directly to alkanes
and these can then be used as diesel substitute and be directly
blended into both heavy diesel and also into the jet fuels.
A couple of other examples of products we worked on,
we also worked on production of resveratrol, this is an antioxidant
ingredient in plants, for example in grape. It's clinically proven to be
beneficial for a number of different human diseases, cancer and
type 2 diabetes treatment, for example. But it's also used
in cosmetics. And this is a pathway that is derived from aromatics,
and we express then the plant genes in yeast and we could then
initially produce some proof of principle levels here. But by
pathway optimization, particularly upstream of phenylalanine
biosynthesis, we could significantly improve the production
as illustrated here. And so this is an example also of how we
can insert complex plant pathways in yeast and use that
-- the yeast thereby to more efficiently produce these compounds.
We also worked on human insulin. Yeast is already used
for commercial production of human insulin by Novo Nordisk,
which is the largest insulin producer in the world. It's also
used for production of many other biopharmaceutical proteins.
But we were interested to see if we could make an efficient
expression system for expression of insulin, but also other
secreted proteins in yeast. And so we evaluated the number
of different expression systems, as illustrated here. And then
we could identify a very efficient expression system where we
could 20-fold improve the secretion of the insulin out of the
yeast cells, and actually reach titers close to 100 milligrams
per liter, which is quite significant and is close to what you
would require for industrial scale production. We also work on
production of human hemoglobin, so this is a protein that
has a heme group inserted into it, and if we can therefore
not have it secreted, so it's accumulating inside the cell.
But there's much interest in producing human hemoglobin
as a blood substitute to be used, for example, in transfusion.
And hereby, you could prevent disease transfer and hemoglobin
is a key ingredient in blood transfusion because of its oxygen
carrying capabilities. What we did was we combined expression
of different forms of the protein of the human hemoglobin,
but we also upregulated the heme biosynthetic pathway in yeast.
And hereby, we could increase the accumulation more than 3-fold
of hemoglobin inside the yeast cell. So let me end off talking
a little bit about somewhat different approach. So we were interested
in having yeast cells that could grow at higher temperatures
and there is much interest in that in the bioethanol industry,
because then you could reduce the cost of cooling, you can also
have a more stable process, for example, combining with
enzymes that like to operate at higher temperatures. So
the problem however, is that it's difficult for yeast to grow
above 35C, it has very, very slow growth. And so one could
maybe begin to look at how the heat shock response functions
in yeast, and engineer this. But the problem is the heat shock
response is something that the cell has evolved to cope with
sudden temperature stresses. And basically, cope with this stress
in order to survive when the temperature later on drops again.
So we therefore took another approach, where we did so-called
directed adaptive laboratory evolution. So we grew yeast
over a series of shake flasks and many generations at 40 degrees,
39.5 to be more specific. And after about 600 generations
we then isolated clones that were able to grow better at
this higher temperature. As illustrated here, we did this in 3
shake flask series. We isolated three clones from each of these
flasks, and as shown here, that all of these 9 clones here,
they grow about twice the rate of the wild type strains at this
elevated temperature. This we also characterized these strains
in bioreactors, and as shown here, they grow faster at this temperature
here. And they also have a higher glucose uptake rate.
So, we then -- sorry, we're then interested to see what happened
actually in these strains. Which genetic mutations accumulated
in order to accommodate for this improved growth at higher
temperatures. So we did genome sequencing, we found
that in some of the clones that we isolated, there was a duplication
in part of chromosome 3, which is one of the smaller chromosomes
in yeast. And we can see here that there are certain segments
that were duplicated here. One could of course speculate whether this
had anything to do with the adaptability and ability to grow at
higher temperatures. However, we also noticed that there was
accumulation of point mutations in several genes, as indicated
over here. And particularly, in one gene was identified to have
a point mutation in the clones that we isolated. And that was ERG3,
so we of course were zooming in to look into ERG3 further.
We did see also accumulation of point mutations in ATP3,
so we also looked further into that. And when we inserted the
point mutation into ATP3, you can see here in this M22 strain
here, we can see that that is not really what brings the
phenotype is not conferring increased growth at high
temperatures. But when we introduce point mutations in
the ERG3 that we identified by mutation, we can see in the M7
strain here, that we basically have improved growth. Almost
as good as those strains that we evolved, and much better
definitely than the wild type. So what is the mutation in ERG3
bring to the cell? Well, what we found was that there is a complete
shift in its sterol content. You can see here, the wild type mainly
is accumulating ergosterol, which is the typical sterol
of yeast. Whereas, the evolved mutant is accumulating fucosterol,
which is illustrated here. It's a bended sterol compared with
ergosterol, which is a more flat sterol. And also when we introduce
the point mutation in ERG3, we can also see that we get this
fucosterol here. So, we are confident that basically changing
the sterol composition is what brings this phenotype and the
ability to grow at an elevated temperature. And it's kind of
remarkable that just a single point mutation actually is sufficient
to bring this adaptability of yeast to grow at higher temperatures.
This performance, or this ability to grow at higher temperatures has
significant translational impact that's illustrated over here.
Because if you grow at higher temperatures, you can see that
the wild type is producing less ethanol than the evolved mutants,
as illustrated here. So with that, let me just acknowledge
everyone who has been working on this. Here's a list of the people
and current members and alumni that have been working on this,
and also collaborators. And of course, also the funders.
And thank you for your attention.
Ver Más Videos Relacionados
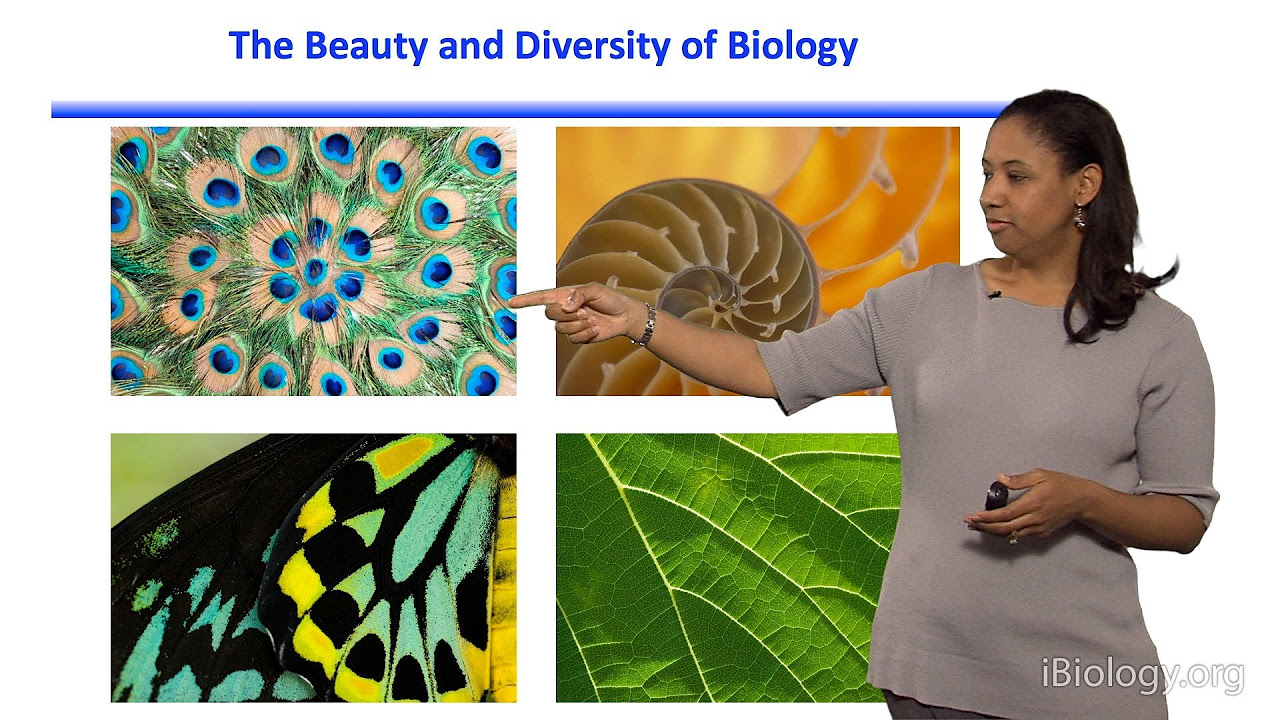
Kristala L. J. Prather (MIT) Part 1: Introduction to Synthetic Biology and Metabolic Engineering

QUÉ ES INGENIERÍA INDUSTRIAL Y CUÁLES SON LAS FUNCIONES QUE REALIZA
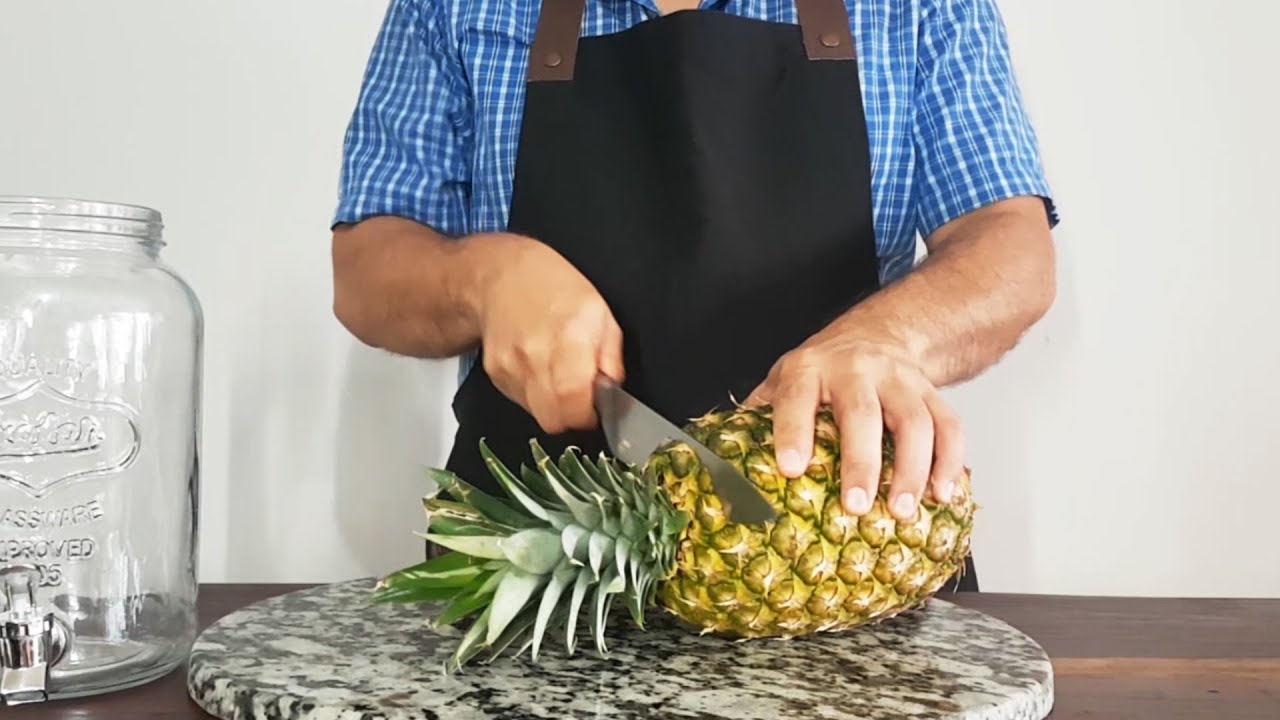
Levadura de Piña, Como Extraerla
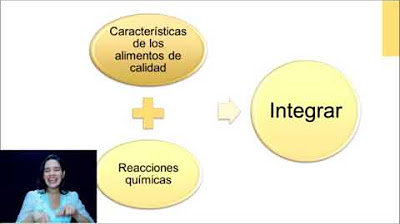
Introducción . Química de los Alimentos

Compuestos Nitrogenados
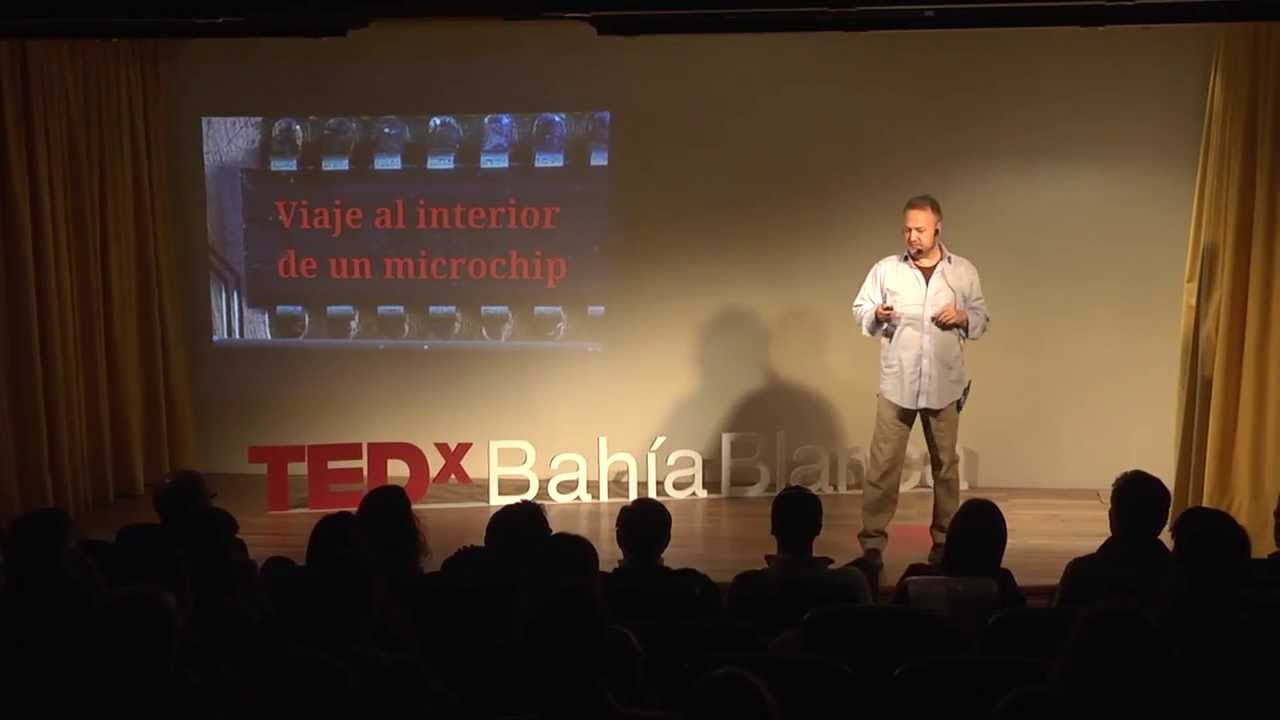
Viaje al interior de un microchip: Pedro Julián at TEDxBahiaBlanca
5.0 / 5 (0 votes)