All Fundamental Forces and Particles Explained Simply | Elementary particles
Summary
TLDRThis script delves into the intricate composition of the human body, from organs and cells to the fundamental particles that make up our universe. It explores the standard model of particle physics, detailing the roles of quarks, leptons, and bosons, and their interactions through forces like the strong nuclear, electromagnetic, weak, and the elusive gravitational force. The narrative highlights the mass-energy equivalence, the color charge of quarks, and the significance of the Higgs boson in imparting mass to particles. It underscores the ongoing quest to unravel the mysteries of the universe at the quantum level.
Takeaways
- 🧬 Humans are made up of organs, bones, blood, nerves, and are composed of around 40 trillion cells, each containing approximately 100 trillion atoms.
- 🔬 If we zoom into a cell, we find molecules, and further into those molecules, we find atoms, which are made up of a nucleus and orbiting electrons.
- 🌌 The nucleus itself is composed of protons and neutrons, which are made of even smaller particles called quarks.
- 🚀 Elementary particles, such as quarks, are the smallest known building blocks of the universe and cannot be broken down into smaller components.
- 🌐 The standard model of particle physics classifies particles into 12 matter particles and 4 force carriers, grouped into families: quarks, leptons, and bosons.
- 🔋 Elementary particles possess three basic properties: mass, spin, and charge, which are crucial for understanding their behavior.
- 👫 Quarks come in six 'flavors' and three 'colors', and they bind together to form baryons like protons and neutrons through the strong nuclear force.
- 💥 The strong nuclear force, mediated by gluons, is the strongest force in the universe but has a very short range.
- ⚡ The electromagnetic force is responsible for the interactions between charged particles, such as electrons and protons, and is carried by virtual photons.
- 🌟 Electrons, which are leptons, orbit the nucleus and can jump between energy levels, emitting or absorbing photons in the process.
- 💫 The weak force, mediated by W and Z bosons, is responsible for processes like beta decay and is much weaker in strength and range than the strong force.
Q & A
What is the human body composed of at its most basic level?
-The human body is composed of organs, bones, blood, nerves, and more, which are made up of cells, molecules, and ultimately atoms.
How many cells and atoms are estimated to be in a single human cell?
-A single human cell contains approximately 100 trillion atoms, and the human body consists of around 40 trillion cells.
What are the subatomic particles found within the nucleus of an atom?
-The nucleus of an atom contains protons and neutrons, which are subatomic particles.
What are elementary particles or fundamental particles?
-Elementary particles, also known as fundamental particles, are the smallest known building blocks of the universe that cannot be broken down into smaller components.
What are the three families of particles in the standard model of particle physics?
-The three families of particles in the standard model are quarks, leptons, and bosons.
What are the basic properties of all elementary particles?
-All elementary particles have three basic properties: mass, spin, and charge.
How many quarks are there in total, and what are their charges?
-There are six flavors of quarks, with up quarks carrying a charge of +⅔ and down quarks carrying a charge of -⅓.
What is the role of gluons in the context of quarks?
-Gluons act as messenger particles, carrying a color charge from one quark to another and facilitating interactions between quarks, thus holding them together.
Why are protons and neutrons considered baryon particles?
-Protons and neutrons are considered baryon particles because they are composed of three quarks each.
What is the strong nuclear force, and how does it relate to the structure of an atom?
-The strong nuclear force is the force that binds quarks together to form protons and neutrons, and it is also responsible for holding the nucleus of an atom together.
What is the charge of an electron, and how does it interact with the nucleus of an atom?
-An electron has a charge of negative 1 and interacts with the positively charged protons in the nucleus through the electromagnetic force, which causes attraction between opposite charges.
What is the significance of the weak force in particle physics?
-The weak force is responsible for processes such as beta decay, which allows an unstable atom to achieve a stable state by converting a neutron into a proton or vice versa.
What is the role of the Higgs boson in the standard model?
-The Higgs boson, associated with the Higgs field, is believed to be responsible for giving mass to all elementary particles and the W and Z bosons.
Why are gravitons hypothetical particles of interest in the standard model?
-Gravitons are hypothetical particles that scientists are trying to add to the standard model as they are believed to be responsible for the gravitational force.
Outlines
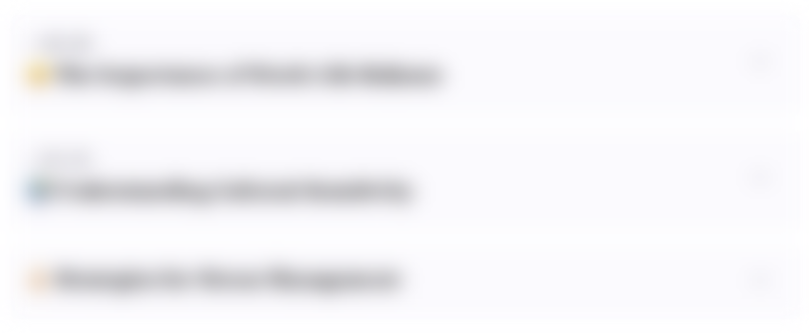
This section is available to paid users only. Please upgrade to access this part.
Upgrade NowMindmap
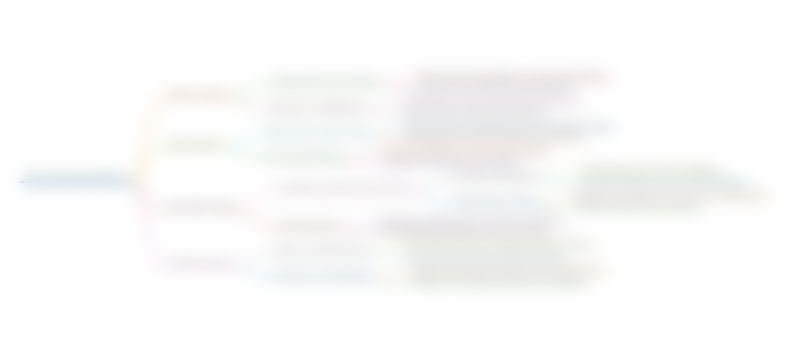
This section is available to paid users only. Please upgrade to access this part.
Upgrade NowKeywords
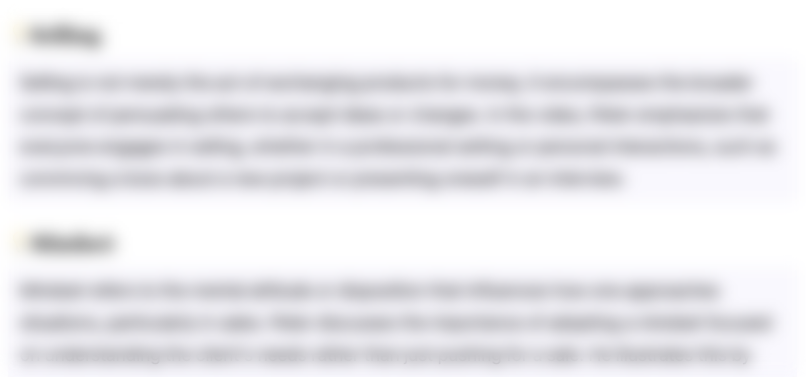
This section is available to paid users only. Please upgrade to access this part.
Upgrade NowHighlights
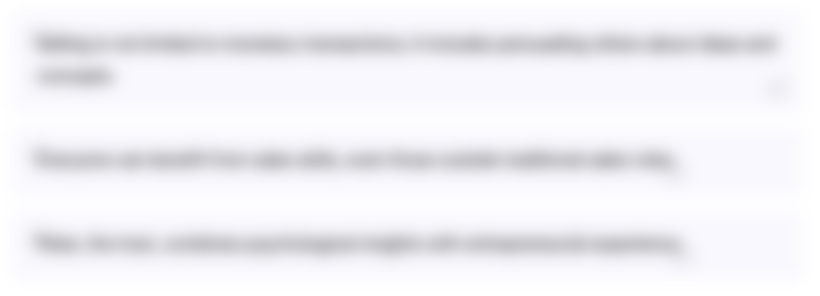
This section is available to paid users only. Please upgrade to access this part.
Upgrade NowTranscripts
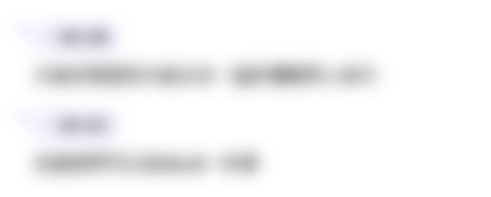
This section is available to paid users only. Please upgrade to access this part.
Upgrade NowBrowse More Related Video
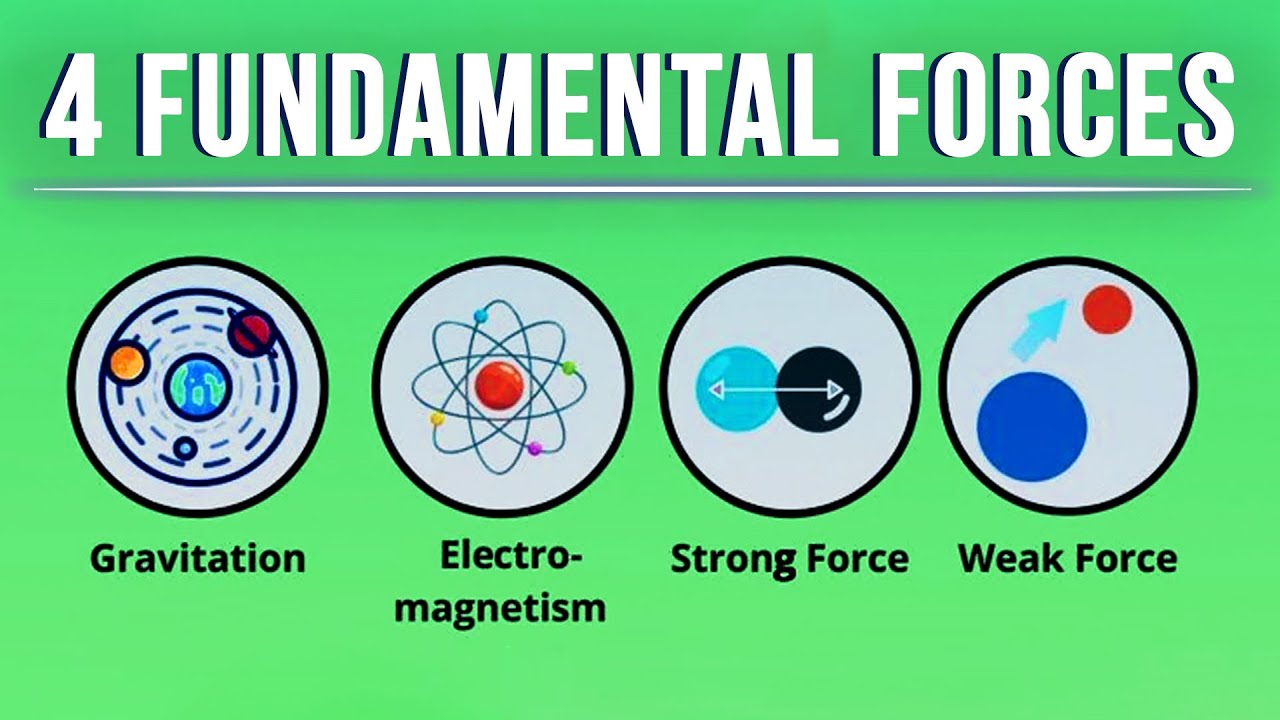
The 4 Fundamental Forces (Interactions) Of Physics Explained
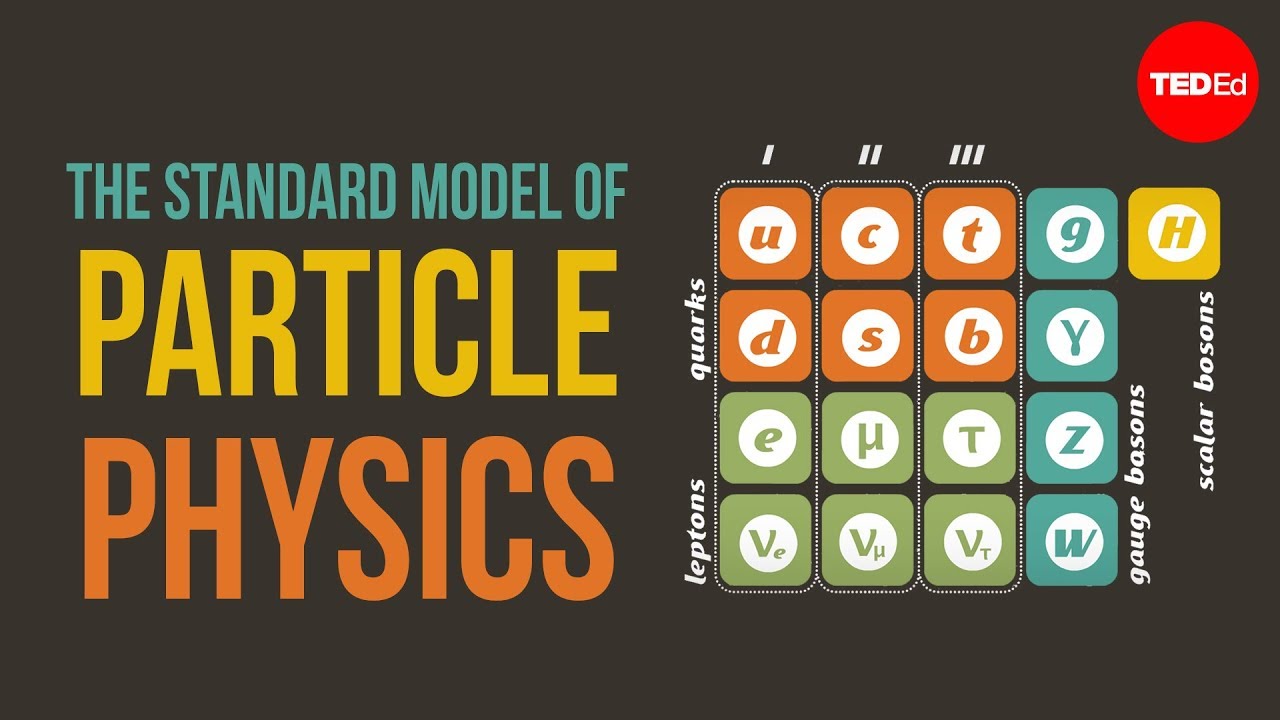
What’s the smallest thing in the universe? - Jonathan Butterworth

All of PARTICLES & QUANTUM in 15 mins - AS & A-level Physics
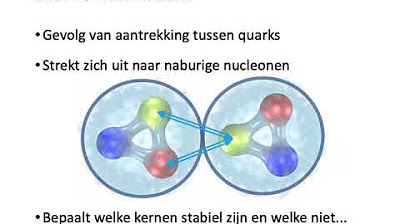
We1 sterke&zwakke ww
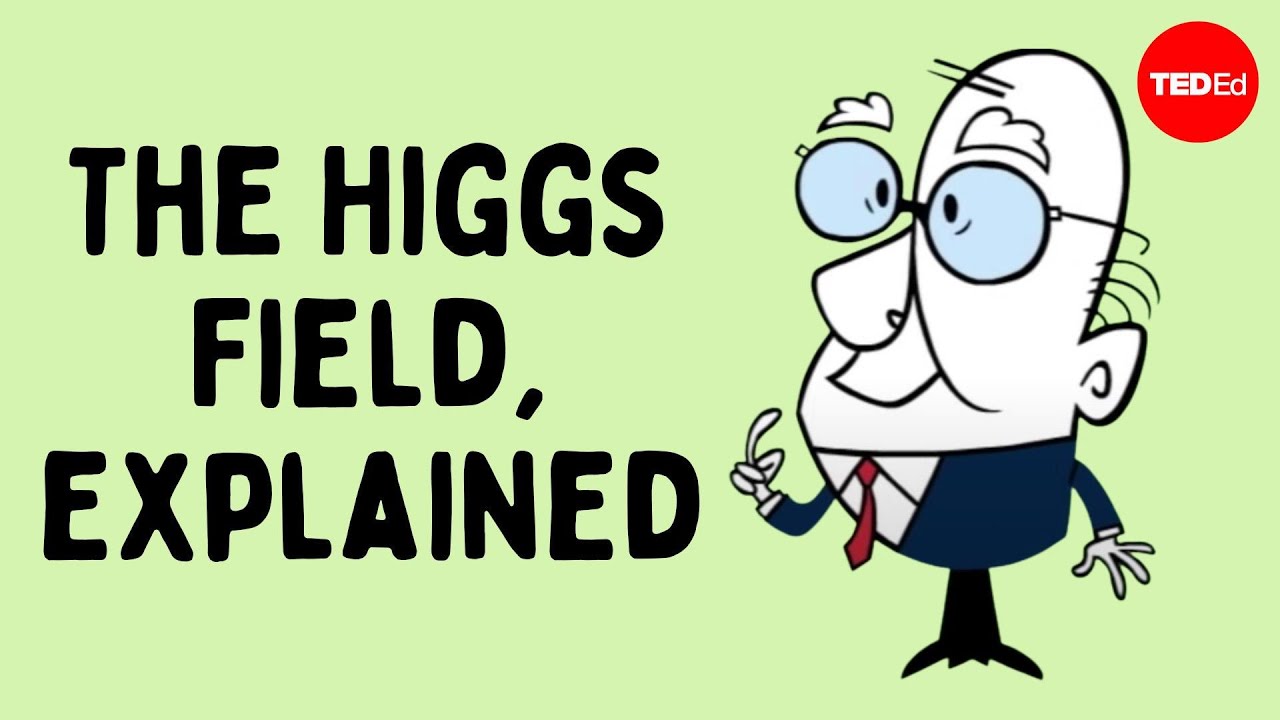
The Higgs Field, explained - Don Lincoln
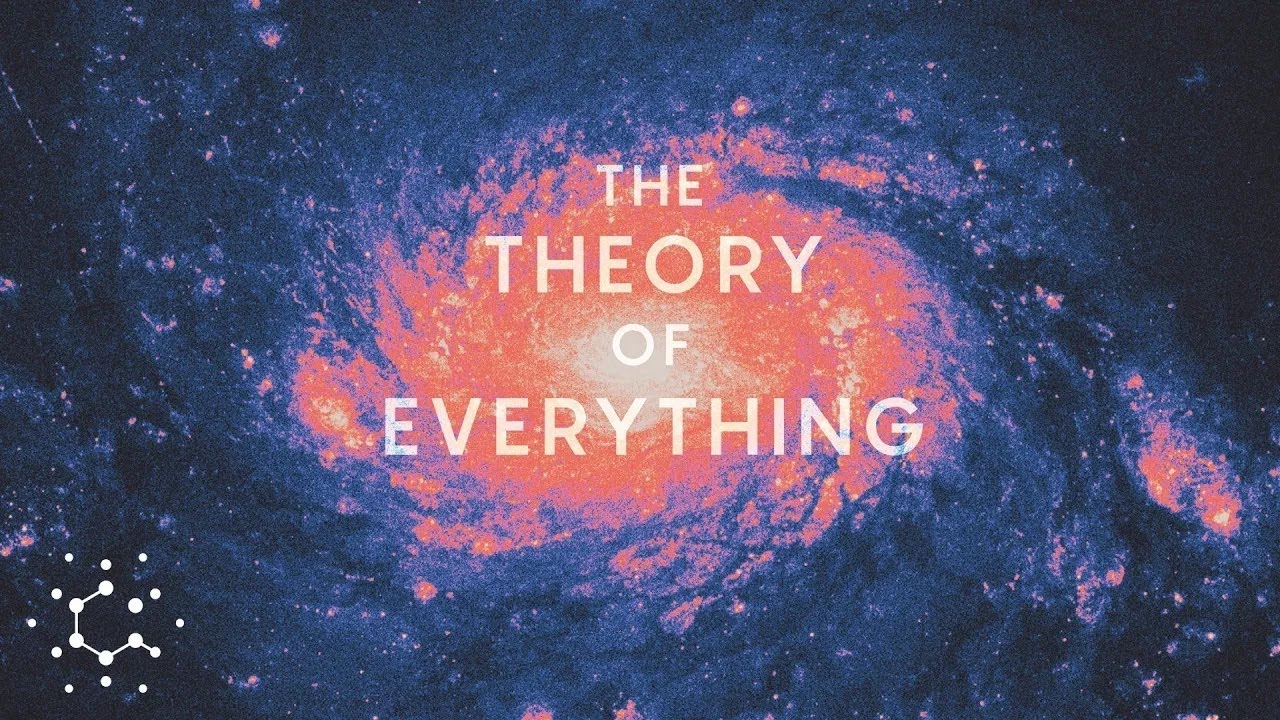
The Standard Model of Particle Physics: A Triumph of Science
5.0 / 5 (0 votes)