A primer to wireless power transfer
Summary
TLDRLa présentation intitulée 'A primer to wireless power transfer' explore l'idée de la transmission de puissance sans fil, une notion ancienne popularisée par Nikola Tesla en 1898. Le texte décrit le concept de base impliquant des bobines primaires et secondaires, la création de flux magnétique et le coefficient de couplage qui détermine l'efficacité de la transfert. L'auteur aborde les défis, notamment la distance, les pertes par résistances parasitaires et la question de la puissance apparente. Il insiste sur l'importance des circuits résonants pour améliorer le couplage et explique le rôle du facteur de qualité (Q) dans ces circuits. La présentation couvre également divers modèles de circuits, y compris les équivalents de résistances pour des charges non linéaires telles que des batteries. Enfin, elle examine les méthodes pour améliorer le découplage et augmenter le coefficient de couplage, comme l'utilisation d'éléments de ferrite pour canaliser les lignes de flux. L'objectif est d'offrir une vue d'ensemble intuitive du transfert de puissance sans fil, soulignant sa complexité et la nécessité d'une adaptation aux différents types de charges pour obtenir des résultats optimaux.
Takeaways
- 🕰️ L'idée de transfert d'énergie sans fil n'est pas nouvelle, remontant à Nikola Tesla en 1898.
- 🔌 Le transfert d'énergie sans fil a été exploré dans les années 1920, avec des suggestions de constructions de transformateurs sans fil dans des journaux bricoleurs.
- 🌐 L'idée de Tesla visait non seulement à une technologie, mais aussi à une foi et une idéologie pour améliorer les relations pacifiques universelles en éliminant la distance.
- 🪢 Le coefficient de couplage est un facteur clé dans le transfert d'énergie sans fil, déterminant la quantité de flux magnétique atteignant le secondaire.
- ⚙️ Le modèle de système peut être basé sur la mutualité ou sur la relation d'un transformateur, avec des notions de permeabilité et de pertes.
- 🔋 L'efficacité du transfert d'énergie sans fil est cruciale, nécessitant une gestion des pertes et une réduction de la puissance apparente.
- 🔗 L'utilisation de circuits résonnants est une approche pour améliorer le couplage entre les bobines et réduire l'impédance totale du circuit.
- 📡 Le facteur de qualité (Q) est important dans les circuits résonnants, influençant la forme de l'onde sinusoïdale et la relation entre l'énergie réactive et réelle.
- 🔧 L'efficacité du transfert peut être optimisée en réduisant les pertes dues aux résistances parasitaires et en utilisant des composants à faible résistance équivalente série (ESR).
- 🔄 La simulation de circuits peut aider à comprendre le comportement du système sous différentes conditions et à trouver les points de fonctionnement optimaux.
- 🛠️ Il existe différentes configurations pour le transfert d'énergie sans fil, y compris des résonances séries et parallèles à l'entrée et à la sortie, chacune ayant ses avantages et inconvénients.
Q & A
Quelle est la première mention de la transmission sans fil de l'énergie ?
-La première mention de la transmission sans fil de l'énergie a été publiée par Nikola Tesla en 1898.
Quel était le but initial de la transmission sans fil de l'énergie selon Tesla ?
-Selon Tesla, le but initial de la transmission sans fil de l'énergie était de contribuer à des relations pacifiques universelles par l'élimination de la distance.
Comment la capacité électronique moderne influence-t-elle la transmission sans fil de l'énergie ?
-La capacité électronique moderne rend la transmission sans fil de l'énergie beaucoup plus pratique grâce à l'amélioration de la technologie.
Quel est le facteur clé dans la transmission sans fil de l'énergie ?
-Le facteur clé dans la transmission sans fil de l'énergie est le coefficient de couplage, qui détermine la quantité de flux magnétique généré dans le bobinage primaire et qui parvient au bobinage secondaire.
Comment les circuits résonnants améliorent-ils la transmission sans fil de l'énergie ?
-Les circuits résonnants améliorent la transmission sans fil de l'énergie en augmentant le coefficient de couplage entre les bobines et en réduisant l'impédance totale du circuit.
Quelle est la définition du facteur de qualité (Q) dans un circuit résonnant ?
-Le facteur de qualité (Q) dans un circuit résonnant est défini comme le rapport entre l'énergie réactive et l'énergie réelle, ce qui indique l'efficacité énergétique du circuit.
Quels sont les défis dans la transmission sans fil de l'énergie ?
-Les défis dans la transmission sans fil de l'énergie incluent la distance, qui affecte le coefficient de couplage, les pertes dues à la résistance parasitaire, et la gestion de la puissance apparente.
Comment l'efficacité de la transmission sans fil de l'énergie est-elle définie ?
-L'efficacité de la transmission sans fil de l'énergie est définie comme le rapport entre la puissance de sortie et la puissance totale entrante, qui inclut les pertes dues aux résistances parasitaires.
Quels sont les avantages de l'utilisation de l'inductance mutuelle dans un modèle de transmission sans fil de l'énergie ?
-L'utilisation de l'inductance mutuelle permet de modéliser la dépendance du courant secondaire en fonction du courant primaire, ce qui simplifie l'analyse et la compréhension du transfert d'énergie.
Quels sont les éléments à prendre en compte pour optimiser la transmission sans fil de l'énergie ?
-Pour optimiser la transmission sans fil de l'énergie, il est important de réduire les pertes dues à la résistance, d'utiliser des condensateurs à faible ESR (résistance série équivalente), et d'adapter la configuration du circuit en fonction de la résistance de charge.
Comment la simulation peut-elle aider dans l'analyse de la transmission sans fil de l'énergie ?
-La simulation peut aider à comprendre le comportement du circuit sous différentes conditions, à identifier les points d'optimisation et à prédire les performances du système en fonction des différentes configurations et paramètres.
Outlines
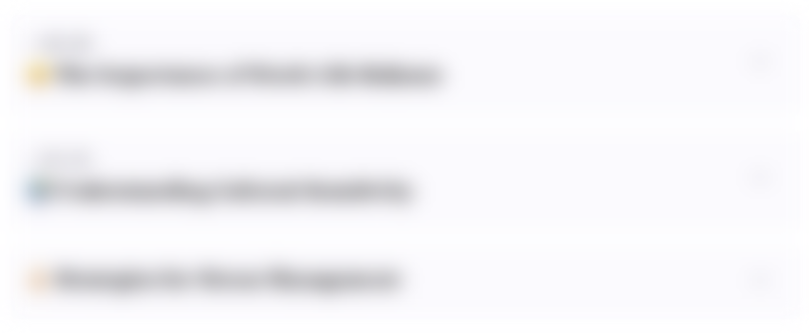
This section is available to paid users only. Please upgrade to access this part.
Upgrade NowMindmap
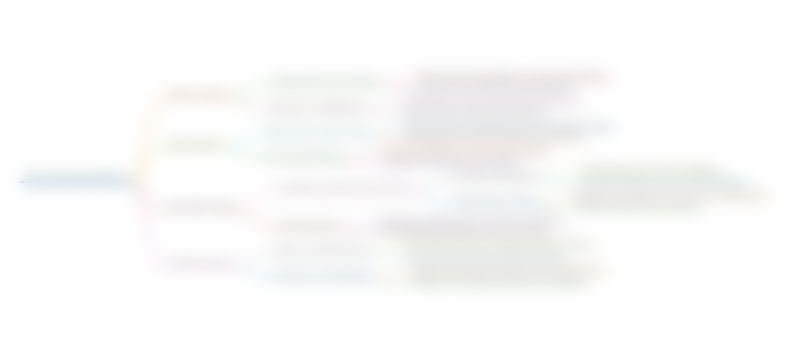
This section is available to paid users only. Please upgrade to access this part.
Upgrade NowKeywords
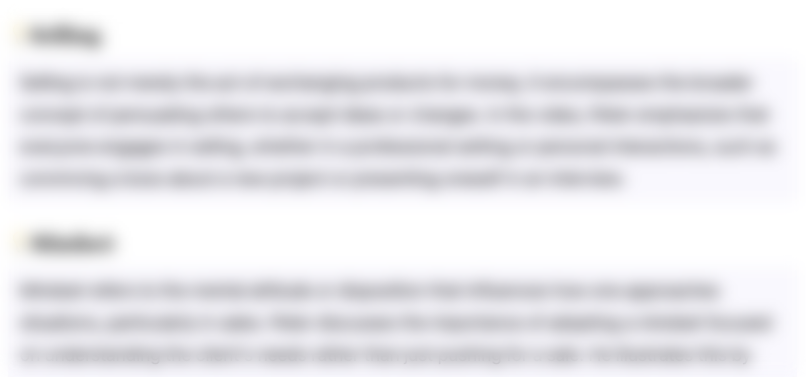
This section is available to paid users only. Please upgrade to access this part.
Upgrade NowHighlights
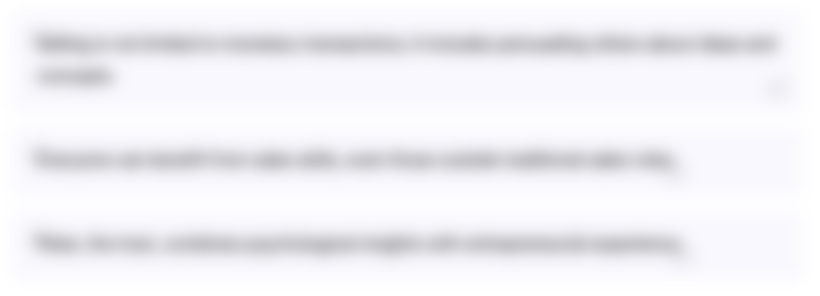
This section is available to paid users only. Please upgrade to access this part.
Upgrade NowTranscripts
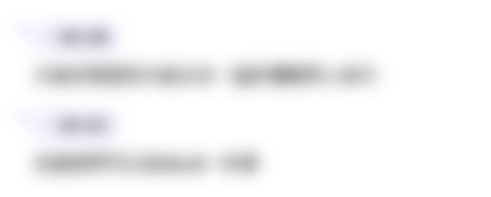
This section is available to paid users only. Please upgrade to access this part.
Upgrade NowBrowse More Related Video
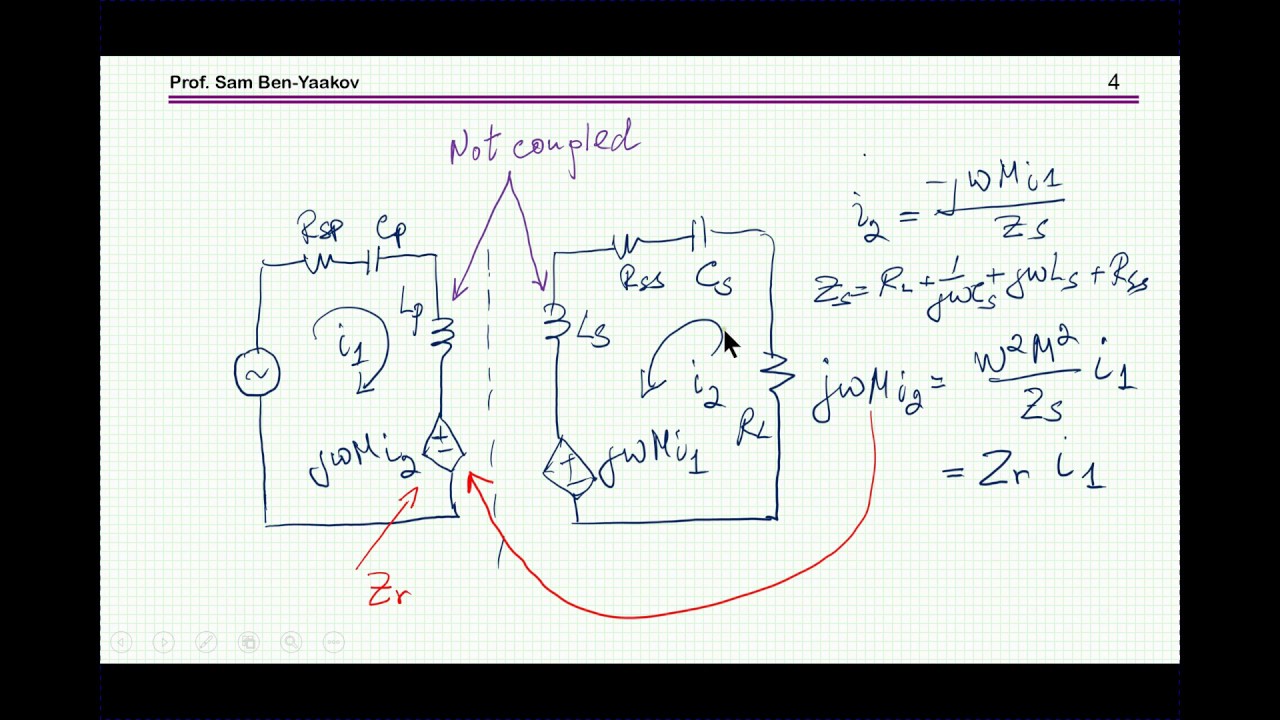
Wireless power Transfer (WPT): Circuit theory limitations of the classical design
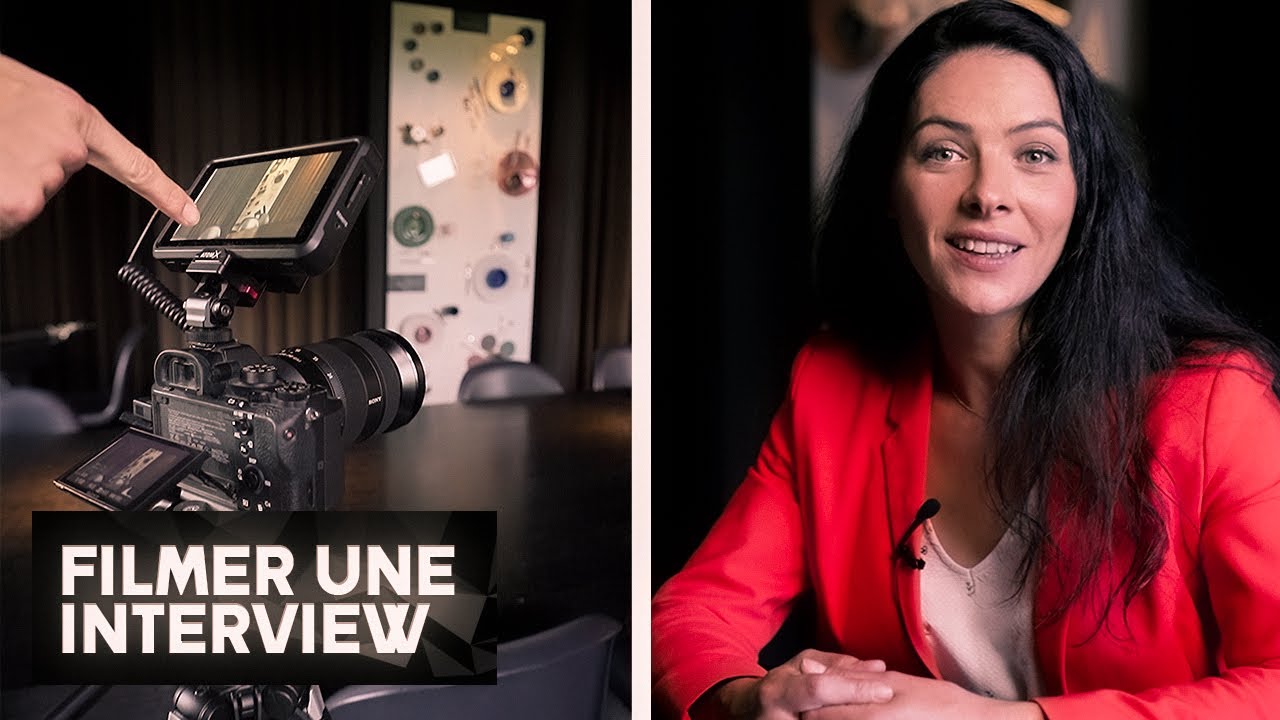
Comment FILMER une INTERVIEW professionnelle
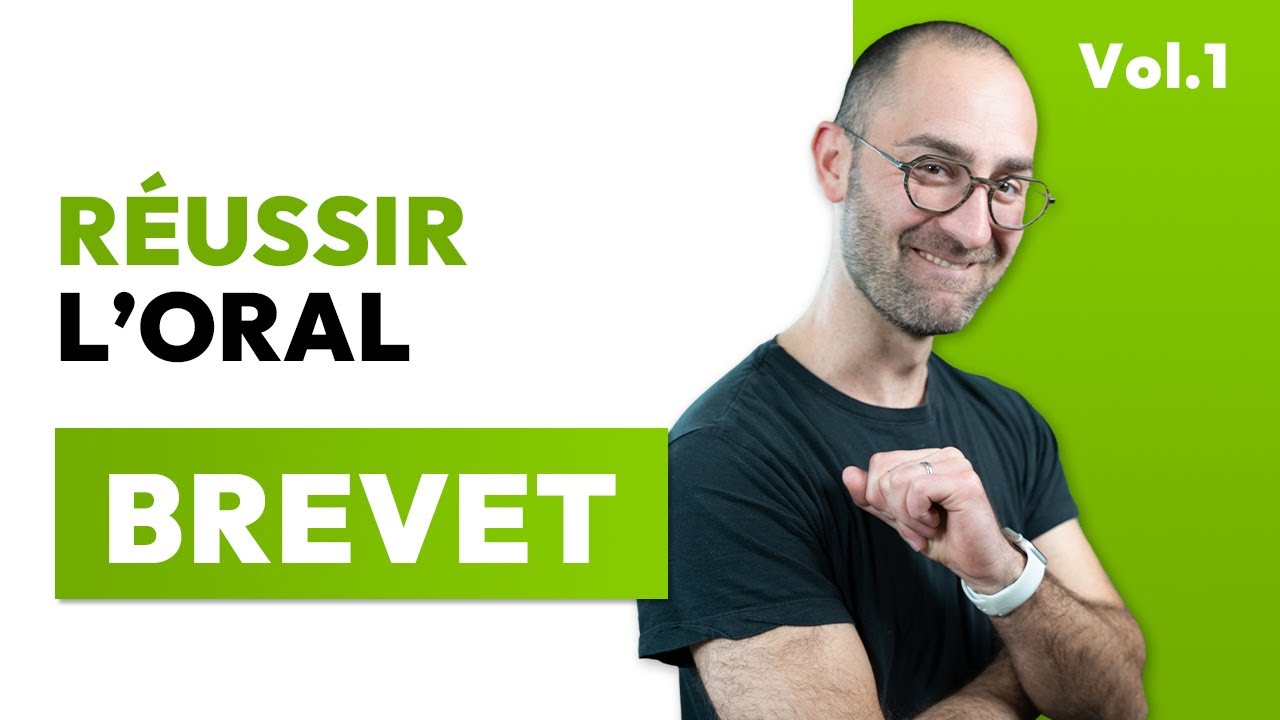
Réussir l'ORAL du BREVET 2022 ! (Partie 1/2)
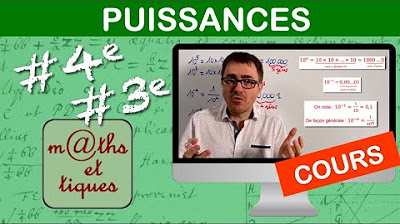
LE COURS : Les puissances - Quatrième - Troisième
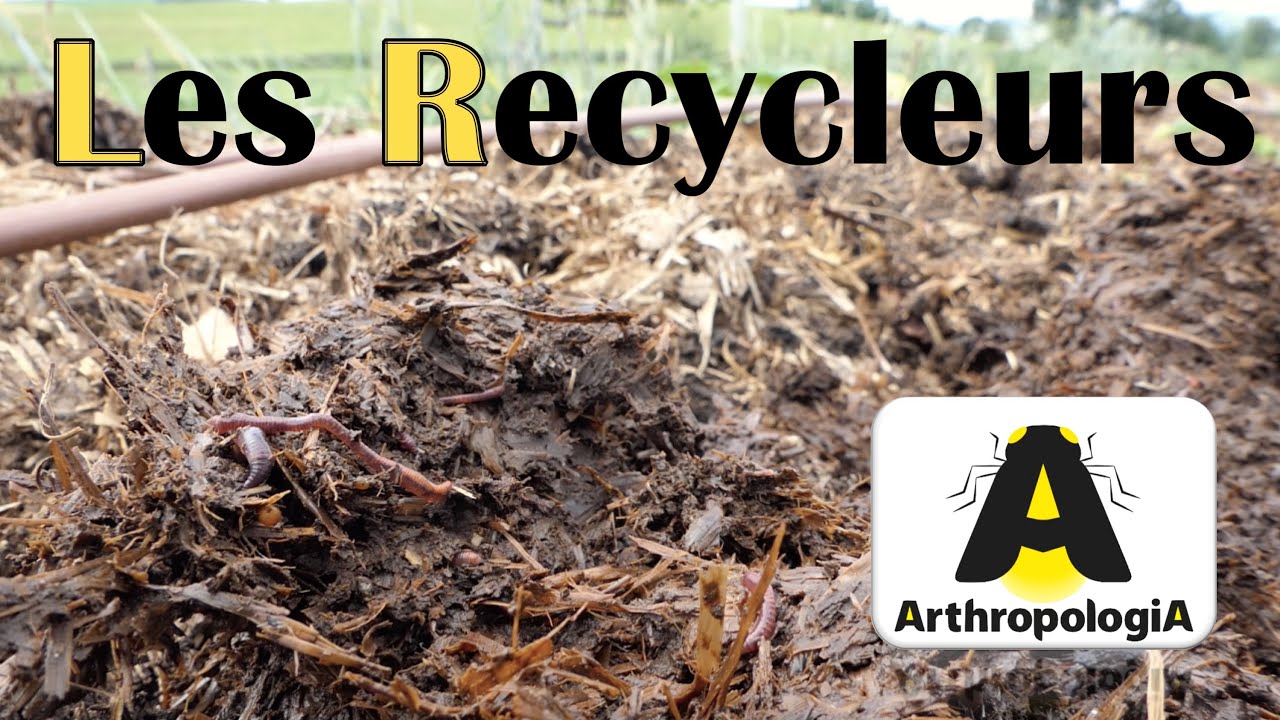
Biodiversité & agriculture : LES RECYCLEURS : Episode 3, Série du Vivant dans les champs
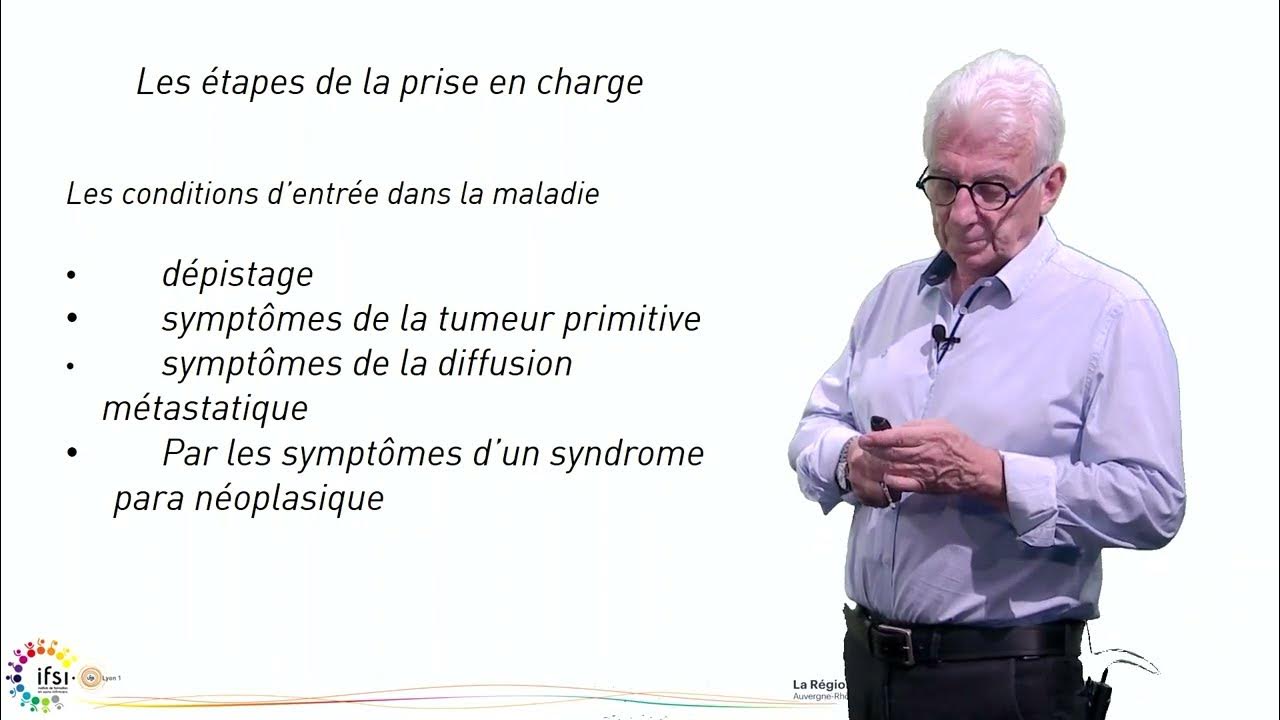
UE29_Martin_Generalites_Seq6_DiagnosticPartie1
5.0 / 5 (0 votes)