VSEPR for 5 electron clouds (part 1) | AP Chemistry | Khan Academy
Summary
TLDRThis video explains how to use VSEPR theory to predict the molecular shapes of phosphorus pentachloride (PClโ ) and sulfur tetrafluoride (SFโ). It begins by drawing Lewis structures to represent valence electrons, followed by identifying electron clouds around the central atoms. Using VSEPR theory, it demonstrates that PClโ adopts a trigonal bipyramidal shape, while SFโ forms a seesaw shape due to the lone pair on sulfur. Bond angles and electron pair repulsion are discussed to explain the stability of these molecular geometries.
Takeaways
- ๐งช **VSEPR Theory Application**: The script uses VSEPR theory to predict molecular geometry, focusing on electron pair repulsion.
- ๐ **Dot Structure Creation**: It explains how to draw a dot structure for molecules, showing valence electrons and their distribution.
- ๐ **Electron Counting**: The process of counting valence electrons for phosphorus pentachloride and sulfur tetrafluoride is detailed.
- ๐ฌ **Central Atom Placement**: Phosphorus is placed at the center of its molecule due to chlorine's higher electronegativity, similarly sulfur for its compound.
- ๐ **Electron Clouds Identification**: The script identifies electron clouds around the central atoms and their influence on molecular shape.
- ๐ **Repulsion and Geometry**: It discusses how electron pairs repel each other to achieve the most stable molecular geometry.
- ๐ **Trigonal Bipyramidal Shape**: For molecules with five electron clouds, a trigonal bipyramidal shape is predicted by VSEPR theory.
- ๐ **Bond Angles**: The script explains the ideal bond angles in a trigonal bipyramidal geometry: 120ยฐ for equatorial, 90ยฐ for axial-equatorial, and 180ยฐ for axial-axial.
- ๐งฉ **Lone Pairs Impact**: The significance of lone pairs in determining molecular shape and their repulsion effects are highlighted.
- ๐ **Formal Charge Consideration**: Assigning formal charges helps in understanding the stability and structure of molecules.
- ๐ ๏ธ **Molecular Shape Prediction**: The final shape of a molecule is predicted by ignoring lone pairs and considering the positions of bonding atoms.
Q & A
What is the first step in predicting the structure of phosphorus pentachloride (PCl5) using VSEPR theory?
-The first step is to draw the Lewis dot structure to show the valence electrons. Phosphorus has 5 valence electrons, and each of the five chlorine atoms has 7 valence electrons, giving a total of 40 valence electrons.
Why does phosphorus exceed the octet rule in phosphorus pentachloride (PCl5)?
-Phosphorus exceeds the octet rule because it is in Period 3 of the periodic table. Atoms in Period 3 and beyond can expand their valence shells to accommodate more than 8 electrons.
How many electron clouds surround the central phosphorus atom in phosphorus pentachloride (PCl5), and what is their arrangement?
-There are five electron clouds around the central phosphorus atom, corresponding to the five P-Cl bonds. These electron clouds are arranged in a trigonal bipyramidal shape.
What are the bond angles in the trigonal bipyramidal structure of phosphorus pentachloride (PCl5)?
-In the trigonal bipyramidal structure, the bond angles between the three equatorial chlorines are 120ยฐ, the bond angle between the two axial chlorines is 180ยฐ, and the bond angles between the axial and equatorial chlorines are 90ยฐ.
What determines the geometry of the electron clouds in VSEPR theory?
-The geometry of the electron clouds is determined by the repulsion between negatively charged valence electrons. These electron clouds arrange themselves to be as far apart as possible, minimizing repulsion.
Why is sulfur tetrafluoride (SF4) considered to have a lone pair of electrons?
-Sulfur in SF4 has 6 valence electrons, and after forming bonds with four fluorine atoms, two electrons remain as a lone pair on the sulfur atom, exceeding the octet rule due to sulfur being in Period 3.
How many electron clouds are around the central sulfur atom in sulfur tetrafluoride (SF4), and how do they arrange themselves?
-There are five electron clouds around the central sulfur atom in SF4 (four bonding pairs and one lone pair). These electron clouds arrange themselves in a trigonal bipyramidal geometry.
Why is the lone pair of electrons placed in the equatorial position in sulfur tetrafluoride (SF4)?
-The lone pair is placed in the equatorial position to minimize repulsion. Lone pairs take up more space than bonding pairs, and placing it in the equatorial position results in fewer 90ยฐ interactions, reducing electron pair repulsion.
What is the molecular geometry of sulfur tetrafluoride (SF4) after considering VSEPR theory?
-The molecular geometry of sulfur tetrafluoride (SF4) is a seesaw shape because the lone pair of electrons distorts the trigonal bipyramidal arrangement.
How does the seesaw shape of sulfur tetrafluoride (SF4) relate to the VSEPR model?
-The seesaw shape arises because the lone pair of electrons in SF4 causes greater repulsion and distorts the ideal trigonal bipyramidal geometry. By ignoring the lone pair, the remaining bonds form a seesaw-like structure.
Outlines
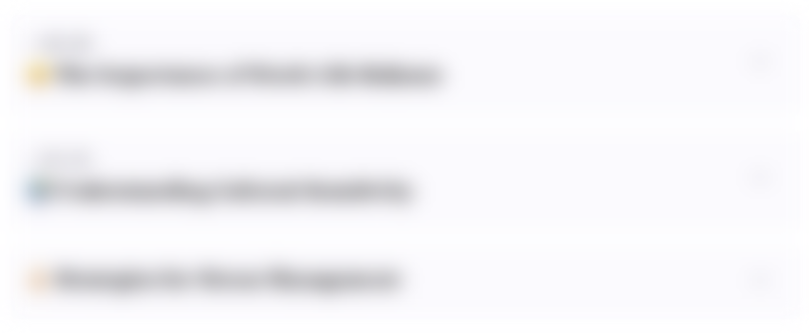
This section is available to paid users only. Please upgrade to access this part.
Upgrade NowMindmap
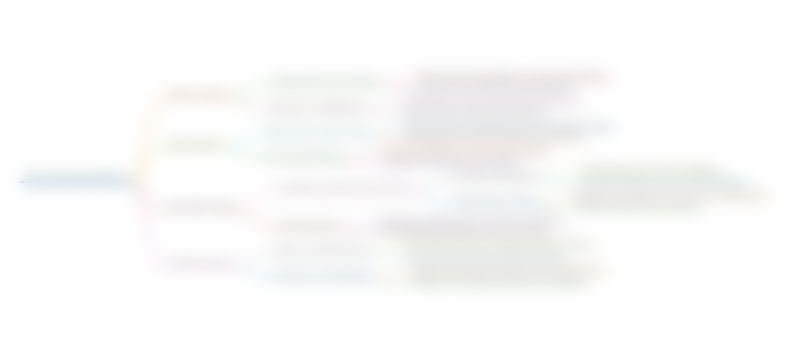
This section is available to paid users only. Please upgrade to access this part.
Upgrade NowKeywords
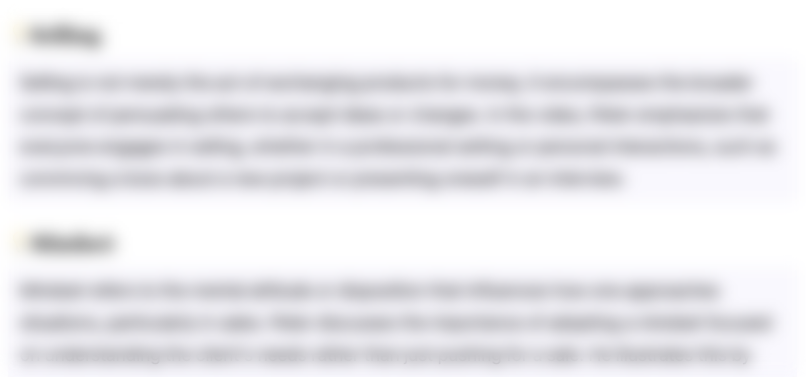
This section is available to paid users only. Please upgrade to access this part.
Upgrade NowHighlights
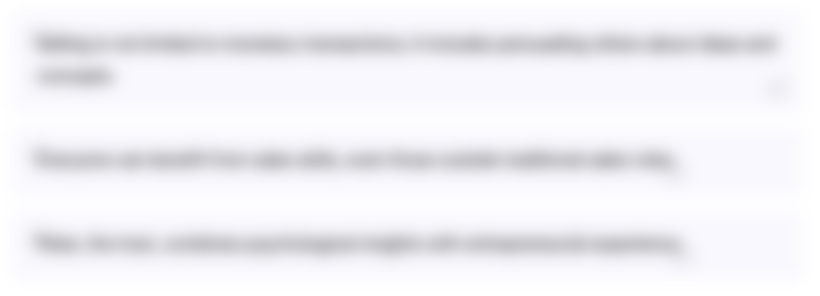
This section is available to paid users only. Please upgrade to access this part.
Upgrade NowTranscripts
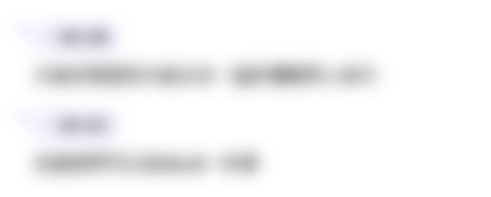
This section is available to paid users only. Please upgrade to access this part.
Upgrade Now5.0 / 5 (0 votes)