Electrons DO NOT Spin
Summary
TLDR量子力学には多くの奇妙な現象がありますが、量子自転という現象は特に理解が難しいとされています。この動画は、電子の量子自転がどのように物質の構造に影響を与え、またどのように量子力学の深遠な洞察につながるかを探求しています。実験と歴史的背景を交えて、量子自転の磁的性質、量子力学での自転の記述方法、そして自転がどのように粒子の相互作用と物質の構造に関わるかを解説しています。
Takeaways
- 量子力学には多くの奇妙な現象がありますが、量子自転という現象は誰も理解していません。
- 物理学の授業で行われる経典的なデモンストレーションは、角動量保存の説明に役立ちます。
- アイアンシリンダーが垂直方向の磁場に置かれ、急に定常速度で回転することを示す実験は、エインシュタイン・デハース効果と呼ばれます。
- 電子は自転していませんが、角動量を持っているようです。これは量子力学的なものであり、電子のような粒子の性質です。
- Stern-Gerlachの実験では、銀原子が磁場を通過し、原子内の電子が磁矩を与えるため、特定の方向に偏向します。
- 量子自転は、電子の性質として量子化されています。測定する方向に依存するため、古典的な回転とは異なります。
- 量子力学では、スピンを記述するために、特別な数学的对象であるスピンオルを使います。
- スピンオルは、360度の回転ではなく、720度の回転が必要とされます。これは、電子のような粒子がどのように振るうかを説明するのに役立ちます。
- 粒子の線形モメンタムは、その位置に根本的に関連しています。同様に、角動量は、粒子の方向性に関連しています。
- スピンは、粒子が持つ回転の自由度から生じるため、角動量は定義できます。
- 粒子はスピン数で区別され、半整数(fermion)または整数(boson)です。この違いは、粒子の相互作用に深い影響を与えます。
- fermionの「反社会的的な」性質は、物質の構造を形成するポアリンエクザル原理に責任があります。
- 量子統計学の定理は、スピンと粒子の性質に関連する現象を説明します。
Q & A
量子スピンとは何ですか?
-量子スピンは、電子などの粒子が持つ非常に奇妙な種類の角動量です。これは、古典的な回転のように考えられがちですが、電子は古典的に回転しているわけではありません。量子スピンは、粒子の基礎的な量子力学的プロパティであり、质量や各種の電荷と同様に重要です。
アインシュタイン・デハアス効果とは何ですか?
-アインシュタイン・デハアス効果は、1915年にアインシュタインとデハアスが行った実験によるものです。この実験では、鉄の円筒をスレッドからぶら下げ、垂直方向の磁場をかけることで、円筒がすぐに一定の速度で回転することを示しました。これは、角動量保存の原理に反するように見える現象です。しかし、実際には、外部の磁場が鉄を磁化し、鉄の外殻の電子が自らのスピンを整列させることで、角動量が保存されます。
Zeeman効果とは何ですか?
-Zeeman効果は、原子が外部の磁場に置かれると、電子がエネルギーレベル間を跳躍させる際に放出されるフォトンの特定の波長が、磁場の存在によって分裂する現象を指します。この効果は、古典的な物理学の考え方で説明できますが、さらに複雑な分裂が観察されたことから、異常Zeeman効果と呼ばれる新たな現象が発見されました。
Stern-Gerlach実験とは何ですか?
-Stern-Gerlach実験は、1921年にオットー・スターンが提案し、翌年ヴァルター・ゲルラークが行った実験です。この実験では、銀の原子が磁場のグラディエントを通過するように射出され、原子の外殻の単一電子が原子に磁矩を与えるため、外部の磁場によって原子に力が働き、それが原子を偏向させます。予想される乱反射の代わりに、銀の原子が2つの地点にのみ当たり、最も極端な偏向に対応する場所に現れます。
量子力学におけるスピンの数学的表現は何ですか?
-量子力学において、スピンはスピンオブジェクトである特別な数学的オブジェクトとして表現されます。これは、一般的なベクトルとは異なり、360度の回転で元の状態に戻る代わりに、720度の回転が必要であるという、非常に奇妙な回転特性を持っています。
fermionsとbosonsの違いは何ですか?
-fermionsは半整数のスピン量子数を持つ粒子で、電子、プロトン、中子などがそれに該当します。一方、bosonsは整数のスピン量子数を持つ粒子で、光子やグルーオンなどの力の媒介者粒子が該当します。fermionsはパウリ排他原理により、同じ量子状態を共有できず、bosonsは同じ量子状態に集積することができます。
スピンが物質の構造にどのように影響を与えるか?
-スピンは物質の構造に根本的な影響を与えます。fermionsのスピンは、パウリ排他原理をもたらし、電子が独自のエネルギーレベルを持つことになり、これが元素の周期表や物質の構造を形成する基礎となります。また、bosonsのスピンは、宇宙の基本的な力の媒介者であり、物質間の相互作用に関与しています。
スピンが現実の構造にどのように関連しているか?
-スピンは、物質の構造を決定するだけでなく、現実の構造にも深く関連している可能性があります。スピンオブジェクトは、空間時間の亜原子的な織物に沿って考えることができます。これらのオブジェクトは、奇妙な結び目のようなものです。スピンは、これらの結び目がどのように機能し、現実が形成されるかを理解する可能性のある手がかりです。
エントロピーと量子纠缠の関係は何ですか?
-エントロピーは、システムの混乱度を測定する指標であり、量子纠缠は、システムの部分間の関連性です。エントロピーは、観察者の視点によって相対的であり、量子纠缠は、システム全体の情報含量を決定する要素の一つです。量子纠缠が低い場合、システムのvon Neumannエントロピーも低くなります。
宇宙の初期状態におけるエントロピーはどのようになっていたか?
-宇宙の初期状態におけるエントロピーは、非常に低いとされています。これは、宇宙が非常に小型で高温で均一であったため、重力的な自由度がほとんど占有されておらず、低いエントロピーを有していたと考えられています。しかし、物質のエントロピーは非常に高かったため、重力エントロピーが物質エントロピーを圧倒していたのです。
電子が回転しているわけではないなぜスピンを如此く表現するのか?
-電子が回転しているわけではないが、「スピン」という言葉を使用するのは、電子が持つ非常に奇妙な種類の角動量を説明するためです。この角動量は、古典的な物理学的な回転とは別の現象であり、量子力学的な性質を持っています。そのため、別の言葉で説明することが難しいため、「スピン」という言葉が使われることがあります。
スピンが電子の磁矩にどのように影響を与えるか?
-スピンは、電子の磁矩に直接影響を与えます。電子はスピン量子数を持っているため、スピンが変化すると、電子の磁矩も変化します。これは、電子が磁场の中で力をを受けたり、銀の原子がStern-Gerlach実験で偏向するようにする現象の原因となります。
アインシュタイン・デハアス効果で観察された角動量保存は、どのようにして解明されたのか?
-アインシュタイン・デハアス効果で観察された角動量保存は、外部の磁場が鉄を磁化し、電子のスピンが整列化することで解明されました。この整列化によって、鉄の円筒が角動量を得、円筒の回転が起こることで角動量が保存されると考えられています。
Outlines
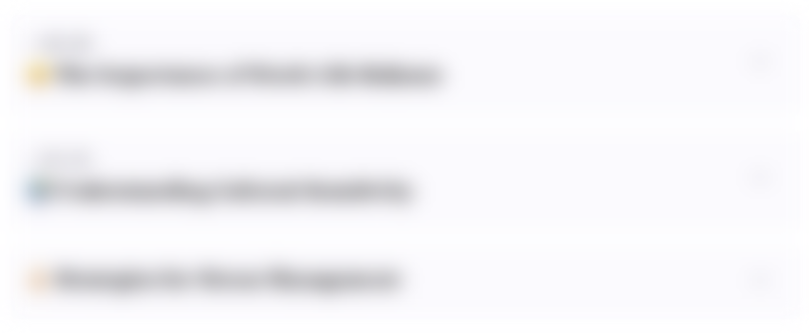
This section is available to paid users only. Please upgrade to access this part.
Upgrade NowMindmap
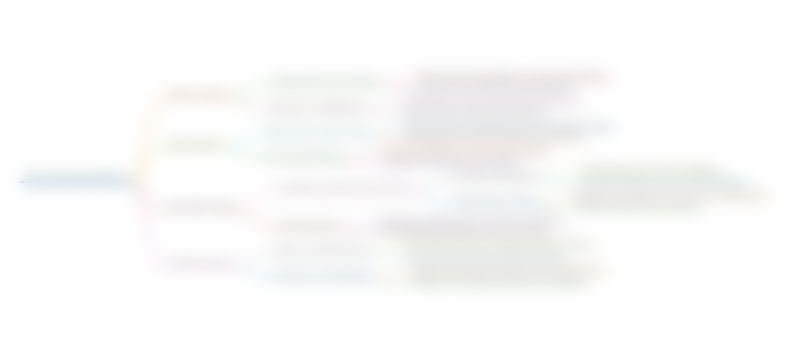
This section is available to paid users only. Please upgrade to access this part.
Upgrade NowKeywords
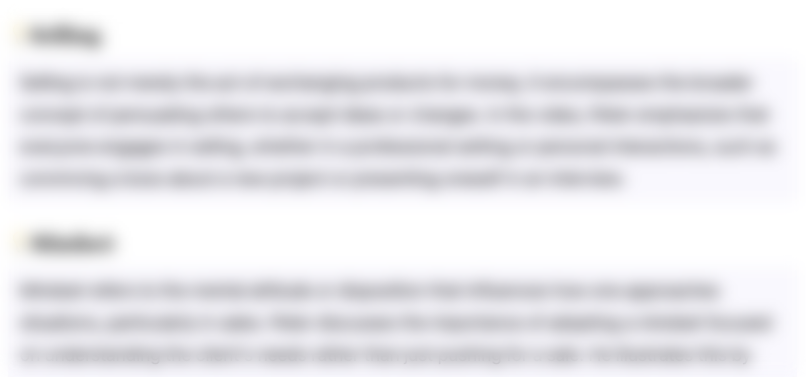
This section is available to paid users only. Please upgrade to access this part.
Upgrade NowHighlights
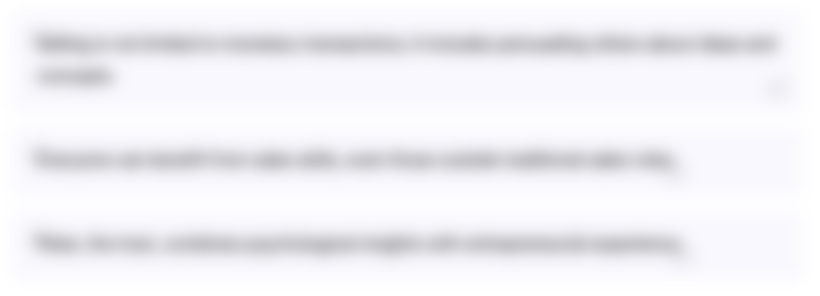
This section is available to paid users only. Please upgrade to access this part.
Upgrade NowTranscripts
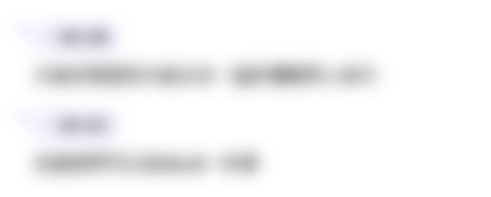
This section is available to paid users only. Please upgrade to access this part.
Upgrade Now5.0 / 5 (0 votes)