DNA Replication 2d'
Summary
TLDRThis lecture delves into DNA replication, focusing on the role of primers and DNA polymerases in the process. It explains that DNA polymerases, including DNA polymerase 1, 2, and 3, synthesize DNA in a 5' to 3' direction, requiring a pre-existing strand. DNA polymerase 3 is highlighted as the primary enzyme for chain elongation, possessing proofreading capabilities to ensure accuracy. The lecture also touches on the removal of RNA primers by DNA polymerase 1 and the joining of Okazaki fragments by DNA ligase. It contrasts prokaryotic and eukaryotic DNA replication, noting the involvement of more enzymes and the presence of telomeres in the latter, which are maintained by the enzyme telomerase to prevent shortening during cell division.
Takeaways
- 😀 DNA replication requires a primer, which is an RNA molecule that provides a starting point for DNA polymerase to add nucleotides.
- 🔬 Bacterial cells have five DNA polymerases, with polymerases 1, 2, and 3 being the most studied and essential for DNA replication.
- 🧬 All three main bacterial DNA polymerases synthesize DNA in a 5' to 3' direction and require a free 3' hydroxyl group to add the next nucleotide.
- 🔄 DNA polymerase 2 has a less clear role but is involved in DNA repair, while DNA polymerase 3 is the primary enzyme for chain elongation during replication.
- 🔍 DNA polymerase 1 is responsible for degrading the RNA primer and filling in the gap with DNA, as well as participating in general DNA repair.
- 🔗 DNA polymerases have proofreading capabilities, which improve the fidelity of DNA replication by correcting incorrect nucleotides.
- 🌐 DNA replication in vitro can be achieved with the right components: deoxynucleoside triphosphates, magnesium, a template, and a primer sequence, along with DNA polymerase 3.
- 🔁 The process involves the removal of RNA primers by DNA polymerase 1 and the joining of Okazaki fragments by DNA ligase to form continuous DNA strands.
- 📚 Eukaryotic DNA replication is similar to prokaryotic but involves more factors, including multiple origins of replication and the need to deal with nucleosomes and chromatin structure.
- 🧵 Telomeres at the ends of chromosomes pose a problem for DNA replication, but the enzyme telomerase helps maintain chromosome length by adding telomeric repeats.
Q & A
What is the role of a primer in DNA replication?
-A primer is necessary for DNA polymerase to add nucleotides to the growing DNA strand. It provides a starting point with a free 3' hydroxyl group for the DNA polymerase to attach the next nucleotide.
How many DNA polymerases are involved in DNA replication in E. coli?
-There are five DNA polymerases in E. coli, but the best studied ones for DNA replication are polymerase I, II, and III.
In what direction do DNA polymerases synthesize DNA?
-DNA polymerases synthesize DNA in the 5' to 3' direction, meaning they can only add nucleotides to the 3' end of the growing strand.
What is the function of DNA polymerase III in E. coli?
-DNA polymerase III is the primary enzyme involved in DNA replication and chain elongation in E. coli. It is responsible for the majority of the DNA synthesis during replication.
What is the role of DNA polymerase I in DNA replication?
-DNA polymerase I is responsible for degrading the RNA primer and filling in the gap with DNA, thus replacing the RNA primer with DNA.
What is the purpose of the 3' to 5' exonuclease activity in DNA polymerases?
-The 3' to 5' exonuclease activity allows DNA polymerases to correct errors by removing incorrect nucleotides that were added in the 3' to 5' direction, thus acting as a proofreading function.
How does DNA polymerase III ensure high fidelity during replication?
-DNA polymerase III ensures high fidelity through its proofreading function, which involves the 3' to 5' exonuclease activity to remove incorrect nucleotides and its active site that checks the diameter of the DNA for correct base pairing.
What are Okazaki fragments and how are they formed?
-Okazaki fragments are short segments of DNA that are synthesized on the lagging strand during DNA replication. They are formed due to the discontinuous nature of lagging strand synthesis, which requires the use of multiple RNA primers that are later replaced by DNA.
What is the role of DNA ligase in DNA replication?
-DNA ligase seals the nicks between Okazaki fragments by forming a phosphodiester bond between the 3' hydroxyl group on one fragment and the 5' phosphate group on the adjacent fragment, thus joining the fragments together.
How is DNA replication initiated in eukaryotic cells?
-In eukaryotic cells, DNA replication is initiated at multiple origins of replication by the action of proteins such as the origin recognition complex (ORC), Cdc6, and Cdt1, which function as helicases to unwind the DNA.
What is the function of telomerase and why is it important for maintaining chromosome ends?
-Telomerase is an enzyme that adds telomeric repeats to the ends of chromosomes, preventing them from getting shorter with each cell division, which would otherwise occur due to the inability of DNA polymerase to fully replicate the ends of linear DNA molecules.
Outlines
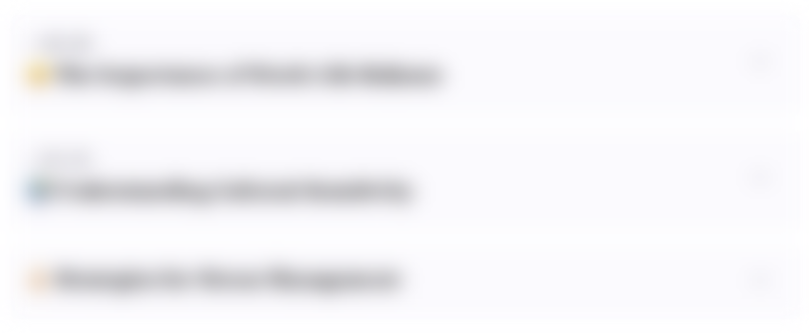
This section is available to paid users only. Please upgrade to access this part.
Upgrade NowMindmap
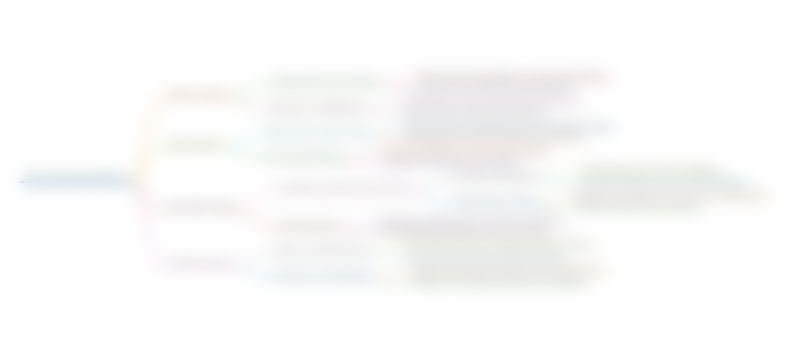
This section is available to paid users only. Please upgrade to access this part.
Upgrade NowKeywords
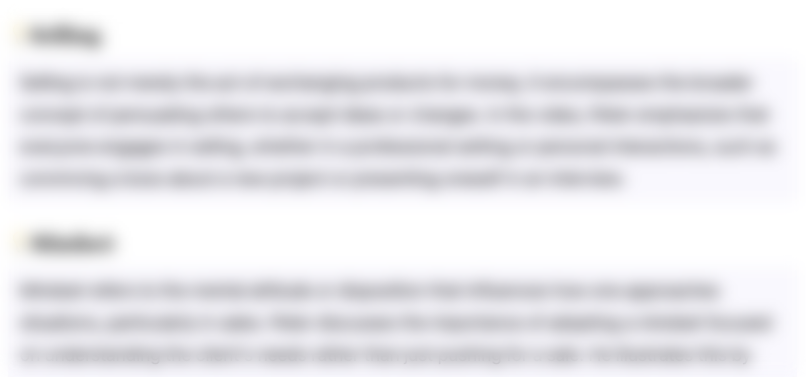
This section is available to paid users only. Please upgrade to access this part.
Upgrade NowHighlights
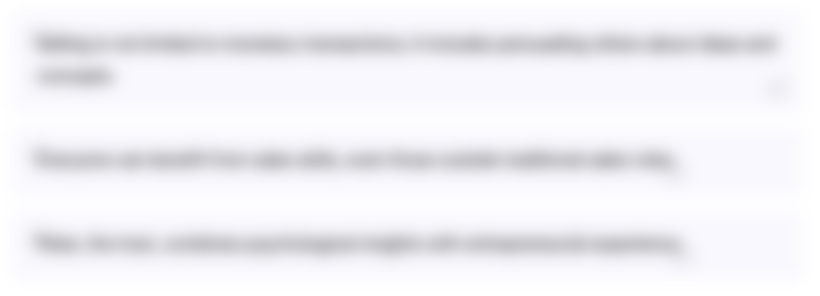
This section is available to paid users only. Please upgrade to access this part.
Upgrade NowTranscripts
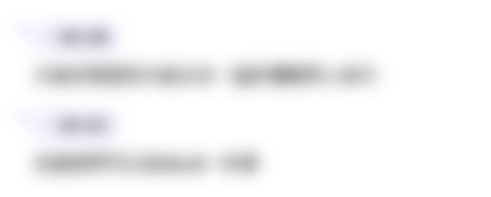
This section is available to paid users only. Please upgrade to access this part.
Upgrade Now5.0 / 5 (0 votes)