The Incredible Properties of Composite Materials
Summary
TLDRこのビデオ脚本では、複合素材の開発が過去数十年間でどのように進歩し、最も先進的な工学問題の解決方法を変えたかを解説しています。自然由来の木材から人工的に設計された様々な複合素材まで、機械的、電気的、熱的、磁性の特性を持ち、特定の応用に最適化されています。特に、繊維強化プラスチック基複合素材は、航空宇宙や自動車業界、スポーツ用品などで広く使用されており、その強さと軽量性に優れています。しかしながら、製造コストや設計の複雑性、もしくは欠陥を持つこともあります。この分野は絶えず進化しており、新しい創造の可能性を広げています。
Takeaways
- 🚀 複合材料の発展は、最先端のエンジニアリング問題の解決方法を変えた。
- 🔥 複合材料は独特の熱的性質を持ち、大気圏再入時の高温にも対応できる。
- ✈️ 軽量で機械的な特性を細かく調整したジェットエンジンのファンブレードが開発された。
- 🌿 複合材料は自然に存在するものもあれば、人工的に組み合わせて開発されたものもある。
- 🔍 複合材料は分散相と基質相の2つ以上の異なる構成材料からできている。
- 📚 分散相は材料の良い特性を提供し、セラミックスや金属が一般的である。
- 🧬 基質相は分散相と機械的化学的結合を形成し、環境からの保護も提供する。
- 🌐 繊維強化、粒子強化、サンドイッチ構造など、複合材料には様々なカテゴリーがある。
- 📈 繊維方向に応力がかかった場合、材料は非常に強力で硬くなるが、垂直方向には弱い。
- 🛠️ 繊維を異なる方向に重ねることで、複数の方向に強度と硬度を持たせることが可能。
- 📊 比強度と比弾性率を見ると、複合材料は従来の材料をはるかに上回る。
- 🛡️ 繊維強化材料は、その強靭さと耐傷性により、衝撃に強いアプリケーションに適している。
- 🔥 非常に高い温度に耐えるためのセラミックス基質と繊維を組み合わせた複合材料も存在する。
- 💡 金属マトリックスを使用した複合材料は、金属の強度や硬度を改善することを目的としている。
- 🏗️ 粒子強化材料は、様々な応用のための材料の特性を調整するための柔軟性を提供する。
- 🍰 サンドイッチ複合材料は、軽量で曲げりやすい構造を持ち、高い曲げり強度を持つ。
Q & A
複合材料とは何で、なぜ特別な性質を持っているのか説明してください。
-複合材料は2つ以上の異なる構成材料からできた材料であり、自然に存在する木材もその一例です。人工的に組み合わせることで、特定の応用に合わせて機械的、電気的、熱的、磁的特性を調整できます。分散相は望ましい材料特性を提供し、基質相は分散相を環境から保護し、負荷を分散します。
複合材料における分散相と基質相とは何を意味するか説明してください。
-分散相は材料の良い特性を提供する部分で、通常はセラミックや金属であり、基質相は分散相と機械的化学的な結合を形成し、負荷を分散させる役割を持ちます。
繊維強化複合材料の主な特徴は何ですか?
-繊維強化複合材料は、繊維と樹脂基質を組み合わせたもので、特に航空宇宙、自動車、スポーツ用品などで広く使用されています。繊維の方向に応じて異方向性があり、非常に高い強度と硬さを持ちます。
何が繊維を細かくすることで複合材料の強度を高める理由ですか?
-繊維の直径が小さいほど、内部の欠陥も少なく小さくなり、強度が向上します。また、細かい繊維では、1本の繊維が折れた場合でも、残りの繊維にかかる負荷が大きく増加しないため、全体の強度が向上します。
複合材料の欠点は何ですか?
-複合材料は製造コストが高いと同時に、設計が難しい一面があります。その異方向性や多様な失敗モードのために、正確なモデル化や失敗予測が困難です。また、金属に比べて割れ易い性質を持っています。
セラミックマトリックス複合材料とは何であり、その利点は何ですか?
-セラミックマトリックス複合材料は、セラミックを基質として繊維を分散させたもので、高温耐性があり、金属や樹脂基質複合材料よりも頑強です。
金属マトリックス複合材料の特徴は何ですか?
-金属マトリックス複合材料は、金属の強度や硬さを改善することを目的としており、例えばアルミニウムやチタンに炭素繊維を組み込むことで、その強度やその他の特性を改善します。
サンドウィッチ複合材料の構造とその利点は何ですか?
-サンドウィッチ複合材料は、薄い外層と軽いコアを挟み込む構造で、軽量化と高反発硬さを兼ね備えています。コアは通常のフォームやハニカム構造であり、外層は金属やCFRPなどの強力で硬い材料です。
OnShapeはどのようなCADプラットフォームですか?
-OnShapeはクラウドベースのCADプラットフォームで、ウェブブラウザ上で動作し、パラメトリックモデリングツールセットやコラボレーションシステム、バージョン管理など、高度な機能を備えています。
複合材料の応用分野には何がありますか?
-複合材料は航空宇宙、自動車、スポーツ用品、構造材料、医療分野、電子部品、構造物など、さまざまな分野で応用されています。
複合材料の強度と硬さをどのように調整するか説明してください。
-複合材料の強度と硬さを調整するためには、繊維の方向、種類、および基質の選択を通じて行われます。例えば、軸方向に強い力がかかる場合、繊維を軸方向に整列させることで、その方向に強い力を持つことができます。
複合材料の設計において、どのようなツールが役立つと言えるのか?
-複合材料の設計では、OnShapeのようなクラウドベースのCADプラットフォームが役立ちます。これは、モデリングツール、コラボレーション機能、バージョン管理などを備えており、設計プロセスを効率化します。
複合材料の製造方法にはどのようなものがあり、それぞれの特徴は何ですか?
-複合材料の製造方法にはウェットレイアップ、プリプレグ、フィラメントウィンディング、インジェクションモールディングなどがあります。それぞれが異なる特性を持っており、製造プロセスや応用に応じて選択されます。
複合材料の内部ダンピング特性とは何であり、その利点は何ですか?
-内部ダンピング特性は、複合材料が動的負荷に対処する能力を指しており、振動や衝撃を吸収する能力が高いことを意味します。これは、自動車や航空機の構造材料など、動的負荷に曝されるアプリケーションにおいて有利です。
複合材料の耐食性とは何であり、その重要性はどの程度ですか?
-耐食性は、複合材料が環境からの腐食や化学的影響にどの程度耐えるかを指します。樹脂基質は繊維を保護し、腐食から守る役割を持ちます。これは特に海洋や化学プラントなど、化学的腐食に曝される環境での応用において重要です。
複合材料の熱的性質にはどのような特徴がありますか?
-複合材料は通常、熱伝達性が低いと同時に、金属に比べて熱膨張係数も低いため、広範囲の温度変化下で寸法の安定性を保ちます。
Outlines
🚀 複合材料の基礎と応用
複合材料とは、2種以上の異なる材料が組み合わさった素材であり、自然の木材もその一例です。人工的に開発された複合材料は、特定の応用に特化した機械的、電気的、熱的、磁的特性を持つことができます。分散相と基質相という2つの相で構成され、分散相は材料の優れた特性を提供し、基質相は機械的・化学的な結合を形成し、分散相を保護します。複合材料は粒子強化型、短繊維強化型、長繊維強化型などがあります。特に、繊維強化型の複合材料は、航空宇宙や自動車産業などで広く使われています。
📚 繊維強化複合材料の構造と製造方法
繊維強化複合材料は、軸方向への力がかかった時に非常に強靭で、垂直方向には弱いという各向异性を持つ。圧力容器など特定の方向に力がかかる場合、繊維をその方向に整列させることで強さを高めることができます。複数の層を異なる方向に重ねることで、各方向に対称な特性を持つ「准同質」のラミネートを作成することが可能です。ウェーブパターンの繊維も存在し、柔軟性があり曲面に適合しやすい特性があります。これらの材料はウェットレイアップやプレプレグ、フィラメントウィンディングなどの方法で製造されます。
📉 繊維強化材料の力学的特性と応用
繊維強化複合材料は、比強度と比弾性率という指標において、従来の材料をはるかに上回る性能を持ちます。そのため、航空宇宙、自動車、スポーツ用品などで軽量化が求められる産業で広く使われています。また、繊維径が小さいほど材料は強力で、多くの小さな繊維を持つ束の方が大きな繊維径を持つものよりも強いという性質があります。しかし、これらの材料は製造の制約やコスト、設計の複雑さ、もしくは割りやすい性質などの欠点もあります。
🔥 高温耐性と金属・セラミック基の複合材料
高温耐性の高い複合材料は、航空宇宙や自動車産業のタービンブレード、宇宙船の熱シールド、ブレーキシステムなどで使われています。セラミック基や金属基の複合材料は、基質材料の強度や硬度を向上させるだけでなく、他の物性も改善することが可能です。例えば、マグネシウムとセラミック粒子の複合材料は、骨の骨折癒着のためのインプラントに適しており、生物相容性があり体内で分解する利点があります。
🏗️ パーティクル強化材とサンドウィッチ構造
パーティクル強化材は、電子部品のヒートスプレッドアーやコンクリートの改良などに使われています。サンドウィッチ構造は、軽量なコア素材を薄い強硬なスキン層の間に挟むことで、高い曲げしん度を持つ構造を作ります。この構造は衛星の構造パネルなど、様々な産業分野で応用されています。複合材料の分野は、新しい素材の開発とイノベーションのための刺激を提供する、絶え間ない進化を続けています。
🛠️ 設計支援ツールの重要性
複合材料を使った設計プロセスには、適切なツールが不可欠です。OnShapeはクラウドベースのCADプラットフォームで、モダンなCADソフトウェアと同じ機能を持ち、さらに多くの利便性を提供しています。パラメトリックモデリングツールセットがあり、パーツのモデリングやアセンブリの管理が容易です。また、コラボレーションシステムが強力で、複数の人々が同じファイルを同時に作業することができます。クラウドベースであるため、強力なマシンは必要ありません。
Mindmap
Keywords
💡複合材料
💡分散相
💡基質相
💡粒子強化複合材
💡繊維強化
💡FRP(ファイバー強化プラスチック)
💡ラミネート
💡ウェーブパターン
💡ウェットレイアップ
💡プレプレグ
💡フィラメントウィンディング
💡サンドウィッチコンポジット
Highlights
复合材料的发展在过去几十年彻底改变了解决一些最先进工程问题的方式。
复合材料允许开发具有独特热性能的材料,例如更好地承受大气再入的高温。
复合材料推动了通过使用轻质风扇叶片来设计喷气发动机的极限,这些叶片具有精心定制的机械性能。
复合材料是由两种或更多不同组成的材料制成的,可以在自然界中找到,例如木材。
复合材料可以工程化,通过精心结合不同材料开发具有特定应用定制的机械、电气、热或甚至磁性属性的复合材料。
复合材料中的一种材料,称为分散相,被另一种材料,称为基体相所包含。
分散相通常提供所需的材料属性,如高强度或改善的延展性,并且通常是陶瓷或金属。
复合材料通常根据分散材料的形式进行分类,例如颗粒增强复合材料或纤维增强复合材料。
基体材料用于与分散相的元素形成机械和化学键,并允许负载在它们之间转移。
复合材料还根据基体材料的类型进行分类,可以是聚合物、陶瓷或甚至是金属。
在工程应用中最广泛使用的复合材料是纤维增强聚合物基复合材料,包括玻璃增强聚合物和碳纤维增强聚合物。
纤维增强材料的基本形式是单向带,所有纤维沿相同方向运行。
纤维增强材料具有高度各向异性,其材料属性在不同方向上会有所不同。
通过堆叠具有不同纤维方向的多层材料,可以构建出具有不同方向上的强度和刚度的组件。
纤维也可以以编织图案排列,具有两种不同方向的纤维。
复合材料的制造方法之一是湿法铺层,其中纤维层在模具中建立,并使用滚筒或刷子将树脂涂在每层上。
另一种方法是使用预浸料,即已经部分固化的环氧树脂预浸渍的纤维带或片。
纤维增强材料之所以特别,是因为它们的机械性能,例如拉伸强度和杨氏模量。
当考虑到它们的质量时,复合材料的比强度和比刚度远远超过传统材料。
纤维增强聚合物基复合材料不仅因其良好的比强度和比刚度而被使用,还因为它们具有其他有用的属性。
陶瓷基复合材料在高温下具有高熔点、高热冲击性和低热膨胀系数等特性。
金属基复合材料通常用于提高金属的强度或刚度,例如将碳纤维掺入铝或钛基体中。
粒子增强材料可以为各种不同的应用而开发,例如具有铜基体和金刚石粒子的复合材料具有比标准材料更高的热导率。
混凝土是一个更常见的粒子增强材料的例子,其中水泥是基体相,骨料是分散相。
夹层复合材料由轻质芯材料夹在由更强更硬的材料制成的薄皮层之间。
复合材料的研究是材料科学中一个令人兴奋且不断发展的领域,为创新开辟了新的机会。
Transcripts
The development of composite materials over the last few decades has completely
transformed how some of the most advanced engineering problems out there can be solved.
It's allowed the development of materials with unique thermal properties that can
better handle the blistering temperatures of atmospheric re-entry, for example.
And has pushed the limits of jet engine design through the use of lightweight
fan blades that have carefully tailored mechanical properties.
But what exactly are composite materials, and what makes them so special? Let's find out.
A composite is really just any material made from two or more distinct constituent materials. They
can be found in nature - wood is just one example of a natural composite material.
But they can also be engineered, where different materials are carefully combined
to develop all sorts of incredible and exotic composites that have mechanical, electrical,
thermal or even magnetic properties that have been tailored to suit a specific application.
In most composites, one material, called the dispersed phase, is contained within another,
called the matrix phase. The ability to carefully select each phase to optimise
the properties of the material for a specific application is what makes composites so powerful.
The dispersed phase is usually what provides the desirable material properties, like high
strength or improved ductility, and is usually either a ceramic or a metal. Composites are often
categorised based on the form of the dispersed material. This is a particle-reinforced composite,
but they can also be fiber-reinforced, either with short, or with continuous fibers.
The matrix material is used to form a mechanical and chemical bond with the elements
of the dispersed phase, and allows loads to be transferred between them. It holds everything
together, and it protects the dispersed phase from the environment. Composites are also categorised
based on the type of matrix material, which can be a polymer, a ceramic, or even a metal.
Probably the most widely used composite materials in engineering applications are the
fiber-reinforced, polymer-matrix composites. This category of composites includes Glass Reinforced
Polymers, also called GRP or Fiberglass, and Carbon Fiber Reinforced Polymers, or CFRP.
These composites usually have an epoxy matrix, which is a thermosetting polymer,
and the dispersed material is glass or carbon fibers, which make up around 60%
of the material by volume. The most basic form of fiber reinforcement is unidirectional tape,
which has all of the fibers running in the same direction.
The individual fibers are grouped together into bundles, which are held together with stitching
or using a chemical binder. In the case of carbon fibers these bundles are called tows.
Each tow usually contains anywhere from 3 thousand to 24 thousand individual fibers.
A typical fiber is around 10 microns in diameter, which is ten times thinner than a human hair.
Any fiber-reinforced material that has fibers all running in the same direction will be highly
anisotropic - its material properties will be different in different directions. If you apply
a load along the axis of the fibers, the material will be much stronger and stiffer than if you
apply it perpendicular to the axis, because the load is taken by the stronger and stiffer fibers
instead of by the matrix. This can be a good thing. If you know that your material will be
loaded mainly in one direction you can orient the fibers to make it very strong in that particular
direction. In pressure vessels for example, fibers can be aligned mostly in the hoop direction,
because the hoop stress is the largest stress when the vessel is pressurised.
In most cases though you need good strength and stiffness in several directions at the
same time. In the case of this pressure vessel there will be axial stresses too,
so we'll also need some reinforcement in the axial direction, either with axial or helical fibers.
This is why components made from fiber-reinforced materials are built up by stacking multiple layers
that have different fiber orientations. Each layer is called a lamina, or a ply,
and the stack is called the laminate. In this laminate the 0 degree layer provides strength and
stiffness in the axial direction. The 90 degree layer provides it in the transverse direction.
And the 45 degree layers provide it in the shear directions.
If enough layers are stacked with the correct orientations, the laminate can have very similar
properties in all of the in-plane directions. This is called a "quasi-isotropic" laminate.
Fibers can also be arranged in weave patterns,
which have fibers running in two different directions.
There are hundreds of possible weave patterns - this is a plain weave, but the twill weave
pattern is also commonly used. There are slight differences in how different patterns behave. A
twill weave is more flexible and will conform more easily to a curved surface, for example.
Weave patterns have good stiffness and strength along the two fiber axes but they're weak at 45
degrees, so they should be layered in different orientations if quasi-isotropic properties are
needed. Once the laminate structure has been defined, the different fiber layers need to
be assembled and combined with the polymer matrix to create the final composite part.
One way of doing this is the wet layup method, where fiber layers are built up in a mould,
and the resin is applied to each layer using a roller or a brush.
The number of plies and ply orientation are carefully selected to achieve the
required properties. An alternative method involves the use of "pre-preg",
tapes or sheets of fibers that have been pre-impregnated in a partially cured epoxy resin,
meaning they can be applied to the mold without needing any additional resin.
The laminate can then be vacuum bagged to ensure it conforms well with the mould and
to remove any voids, and it will then need to cure. The polymer matrix is usually a thermoset,
a polymer that irreversibly hardens when heated, in which case curing is done at
elevated temperatures in an oven.
Filament winding is another manufacturing method where a machine is used to
wind unidirectional tape that has been impregnated with resin around a mandrel.
Once complete the mandrel can either be left in place or removed, and the structure is cured.
Other methods like injection moulding can be used for composites reinforced with short fibers, since
the orientation of the fibers can be arbitrary. So why are fiber-reinforced materials so special?
To find out, let's look at their mechanical properties. This graph shows tensile strength
on the vertical axis, and Young's modulus, which represents the stiffness of a material,
on the horizontal axis. Let's plot a few common engineering materials - titanium alloys, aluminum
alloys, mild steel and high strength steel. Next we can add carbon-fiber reinforced polymers.
A plain weave carbon fiber material has a tensile strength of around 600 Megapascals, and a Young's
modulus similar to Aluminum, although the exact properties will depend on a number of factors,
including the type of polymer matrix that's used and the layup configuration. A unidirectional
carbon fiber material is much stronger than the plain weave, and has higher stiffness as well,
although remember that this is only true if the load is applied along the fiber axis.
These materials correspond to standard carbon fiber grades,
but there are also high strength, high modulus and ultra-high modulus variants.
We can also plot glass-fiber reinforced polymers, which have lower stiffness but very good tensile
strength. E-glass and S-glass refer to different glass fiber compositions that are optimised
for different applications. E-glass is the most commonly used type and was originally developed
for electrical insulation applications, and S-glass was developed for structural applications
and has improved strength. The really amazing thing about these fiber-reinforced composites only
becomes apparent when considering their mass. If we plot specific strength and specific stiffness
on this graph, by dividing by the material density, it's clear that the composites far
outperform traditional materials. The unbelievable strength-to-weight and stiffness-to-weight ratios
of CFRP materials are why they're so commonly used in industries where weight reduction is critical,
like aerospace, the automotive industry, and even in sports like cycling and sailing.
Glass fiber-reinforced composites have lower stiffness than CFRP,
but excellent strength properties on a per-weight basis, and are much more cost effective than CFRP.
They're often used in wind turbine blades and in the construction of boats, where light weight,
high strength and low cost are critical parameters. The impressive strength of
fiber-reinforced composites is in large part due to the small diameter of the reinforcing fibers.
The strength of a fiber, like any material, is limited by the presence of defects within its
microstructure, from which cracks can form and grow to failure. The larger a fiber is,
the more likely it is that it will contain more defects, and that the defects will be larger.
This means that if you take two fiber bundles with the same cross-sectional area,
but different fiber diameters, the bundle with the smaller fibers will be stronger. Not only that,
but in the bundle of smaller fibers, failure of a single fiber can occur without hugely increasing
the load on the remaining fibers. And the smaller the fibers the larger the surface area between the
fibers and the matrix, which means better load transfer between the two. The result is that the
strength of a fiber-reinforced material increases significantly as the fiber diameter reduces.
The main thing limiting the use of ever thinner fibers is manufacturing constraints.
Fiber-reinforced polymer matrix composites aren't only used for their good specific
strength and specific stiffness. They have many other useful properties
that provide advantages over traditional materials like steel and aluminum alloys.
They have excellent internal damping properties, which can be useful for applications involving
dynamic loads, and they have good corrosion resistance the polymer matrix does a great
job of protecting the reinforcing fibers from the environment. They also have interesting thermal
properties - they're usually relatively poor conductors of heat, and have very low thermal
expansion coefficients compared to metals, which can be useful for applications requiring good
dimensional stability over a wide range of temperatures. But they also have drawbacks.
The cost is one - they're significantly more expensive than using standard metals. They can
also be difficult to design with, because their highly anisotropic nature and complex and varied
failure modes make it difficult to accurately model their behaviour and to predict failure
And integration of fiber-reinforced parts into a larger assembly isn't always straightforward
welding isn't an option, and although mechanical fasteners can be used, they tend not to perform
as well as they do in metals, so fiber-reinforced polymers are usually bonded to other parts using
adhesives. Another drawback is the brittleness of these materials. Let's compare stress-strain
curves for a few fiber-reinforced polymers alongside steel and an aluminum alloy.
Fibers tend to be made from materials like glass and carbon because they have high strength and
stiffness, but they're also very brittle. This means the resulting composite material is also
quite brittle - CFRP in particular will fail at very low strains compared to steel and aluminum
alloys. A well known fiber-reinforcement we haven't mentioned yet is Kevlar,
a type of Aramid fiber. Kevlar-reinforced polymers are stiffer and stronger than GRP,
more ductile than CFRP, and lighter than both. This makes them ideal materials
for applications where excellent impact resistance is required, like in body armor.
Another issue with materials that have a polymer matrix is that above temperatures
not much higher than 100 or 200 degrees Celsius the polymer will typically start breaking down,
limiting the maximum temperatures the composites can be used at to well below the level of metals.
If you're working with extremely high temperatures, you'll have to turn to
ceramic materials, like Alumina, Silicon Carbide, and Silicon Nitride, because they have very high
melting points, much higher than metals and polymers. They can withstand temperatures
upwards of 1000 degrees Celsius. Ceramics have other properties that make them useful at these
high temperatures, including high thermal shock resistance and low thermal expansion
coefficients. Plus they have high strength and high stiffness. Carbon has similar properties
to these ceramics with a melting point above 3000 degrees it can handle extremely high temperatures.
But all of these materials are very brittle. They fracture suddenly at very low strains,
which limits how useful they are. And this is where once again the use of composites
can make a big difference. Adding Silicon Carbide fibers to a Silicon Carbide matrix, for example,
results in a material with significantly increased toughness. To see how this works let's compare
two ceramic materials, with and without fiber reinforcement. Both contain an initial crack.
When a load is applied, the crack in the pure ceramic propagates very quickly, resulting
in failure of the material. In the composite though the fibers bridge any cracks that form
in the matrix, which prevents them from growing, increasing the overall toughness of the material.
Unlike polymer-matrix composites, where the aim is to have a strong bond between
the matrix and fibers, so that loads can be transferred between the two,
in ceramic-matrix composites the fibers are coated to allow them to slide somewhat within the matrix,
so that cracks in the matrix don't overstress the fibers. The resulting composite is extremely
resistant to temperature without being too brittle. Composites with a silicon carbide
matrix and silicon carbide fibers are used in high temperature jet engine turbine blades.
And carbon-carbon composites have applications in spacecraft heat
shields to protect from the extremely high temperatures during atmospheric re-entry.
They're also used in the braking systems of some aircraft and even in high performance cars.
Composites with a metal matrix are usually used to try and improve the strength or stiffness
of a metal, which often involves incorporating carbon fibers into an aluminum or titanium matrix.
But sometimes the goal is to modify other properties of the metal. One example of this is
the use of magnesium in biomedical engineering. Magnesium is a very promising metal for use in
implants designed to heal bone fractures, because it's lightweight and has excellent
biocompatibility. Another advantage it has over commonly used metals like titanium is that it
biodegrades in the body, so a second surgery isn't needed to remove the implant once the
injury has healed. But it has quite low strength, and biodegrades too quickly to be all that useful.
Researchers have found that by replacing pure magnesium with a composite that has a
magnesium matrix and a dispersed phase of ceramic particles, the degradation rate can be controlled
and the material strength and other properties are greatly improved. It's really quite incredible.
Particle-reinforced materials can be developed for all sorts of different applications.
Designing an electronic component that dissipates a lot of power? A heat spreader
made from a composite with a copper matrix and diamond particles will have higher thermal
conductivity than standard materials, allowing you to dissipate the heat more effectively.
The composite also allows the thermal expansion coefficient of the heat spreader to be tailored
to match the properties of the chip die, which helps avoid high shear stresses between the two.
Concrete is an example of a much more common particle-reinforced material.
The matrix phase is cement, and the dispersed phase is aggregate, a mixture of sand and
crushed stone. The cement binds everything together, and the aggregate improves strength,
and has the added advantage of being less costly than the cement. A more recent development is the
use of engineering cementitious composites. These composites incorporate short randomly-oriented
polymer fibers into a concrete matrix to obtain a material that has the properties
of concrete but is also ductile, which is why it's sometimes called bendable concrete.
This just illustrates that the possibilities for innovation using advanced composites really
are endless. There are so many different ways materials can be combined to obtain something
useful. A final category of composites is sandwich composites, where a lightweight
core material is sandwiched between thin skin layers made of a stronger and stiffer material.
The lightweight core is typically a foam or honeycomb structure, and the skin layers are
either metals, like aluminum, or a composite, like CFRP. The layers are bonded together using
an adhesive, and the result is a lightweight structure that has high bending stiffness.
Under loading the sandwich composite behaves in a similar way to an I-beam.
The outer layers carry bending loads, like the flanges of an I beam, one side being in tension
and the other in compression. The core is like the web it carries shear loads, but also increases the
distance between the outer layers, increasing the second moment of area of the cross-section.
Inserts are incorporated into the panel to allow the use of threaded fasteners.
Honeycomb panels are used extensively in satellites as structural panels to which
instruments and communication equipment can be attached.
There's no doubt that the study of composites is an exciting and constantly evolving field
in materials science, that opens up new opportunities for innovation.
Whether for fun or for professional projects, having an understanding of the different
composite types will help you design and build stronger, lighter, and better performing products.
Equally important in the design process is having access to the right tools to bring
your projects to life, and that's why I'd like to tell you about this video's sponsor OnShape.
OnShape is a really impressive and unique cloud-based CAD platform that has all of the
functionality you would expect from modern CAD software, but also much more. And it all runs
in your web browser. It has a rich parametric modelling toolset that makes modelling parts
and managing assemblies easy, with loads of tools to make your workflow really efficient,
like standard part libraries, and the Frame and Sheet Metal features. You can even develop
your own custom features, or download ones that have been created by the community.
One of OnShape's most powerful and unique features is its amazing collaboration system
that allows multiple people to work on the same files at the same time - really
useful if you're working with others and building something as a team.
It also has a great version control system that lets you easily log changes and roll back to
previous versions if needed. And because it's cloud-based you don't need a powerful machine
to run it, since all of the processor heavy tasks are done in the cloud. You can access
your files from any machine just by logging in, and they even have Android and iOS apps.
You can get started creating designs of your own using OnShape, for free, in just a few minutes.
Just head over to OnShape.pro/EfficientEngineer to create your free account and start designing.
It's genuinely a great CAD platform and I highly recommend you check it out.
And that's it for this look at composite materials. Thanks for watching!
関連動画をさらに表示
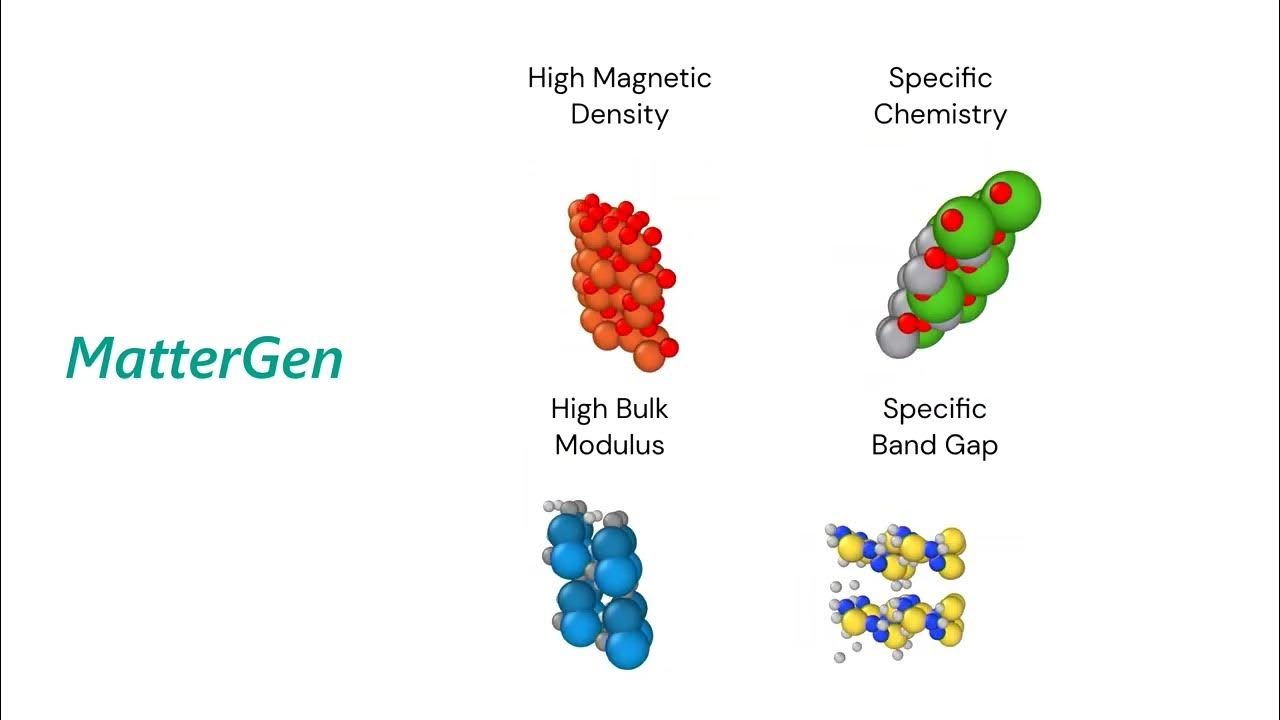
Unlocking Real world solutions with AI – Chris Bishop
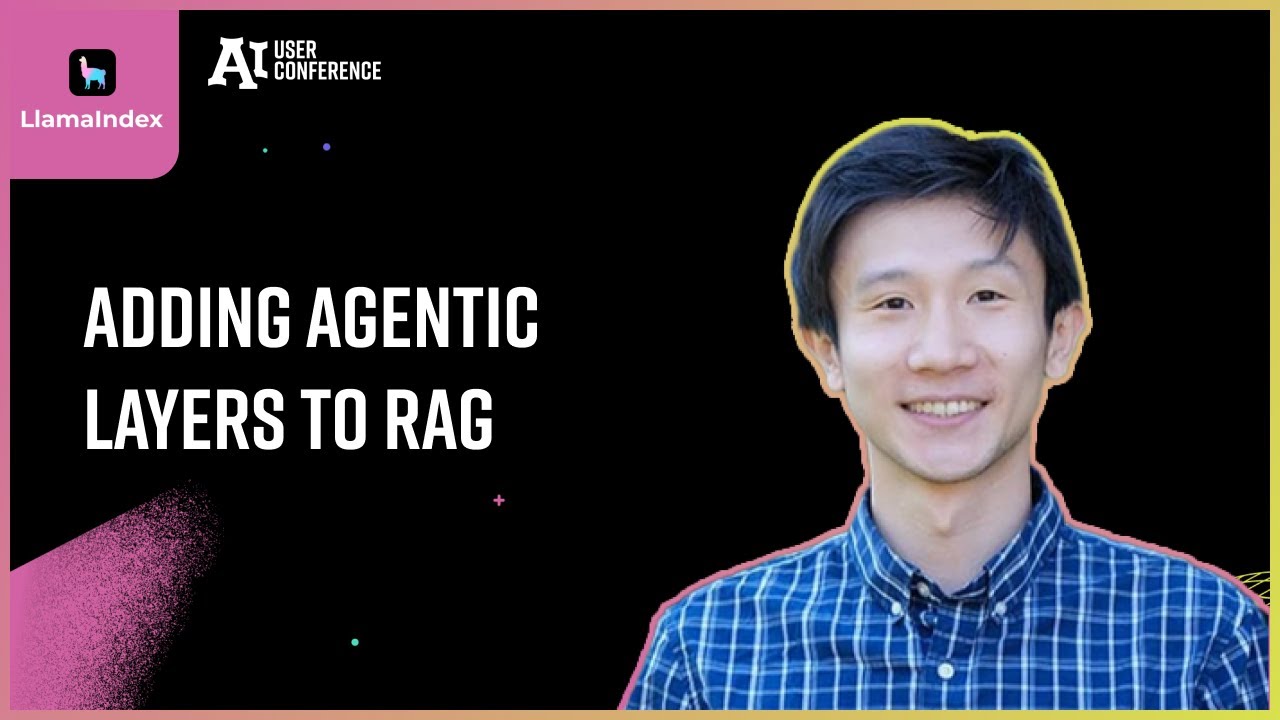
Adding Agentic Layers to RAG

【わかりやすい】量子コンピュータ解説 実用化はいつ?どんな分野に応用できる?(キーワードで振り返る1週間)

【GPT-4oを超えた】OpenAI o1 が公開 【ChatGPTは次のフェーズへ】| ホリエモンAI解説
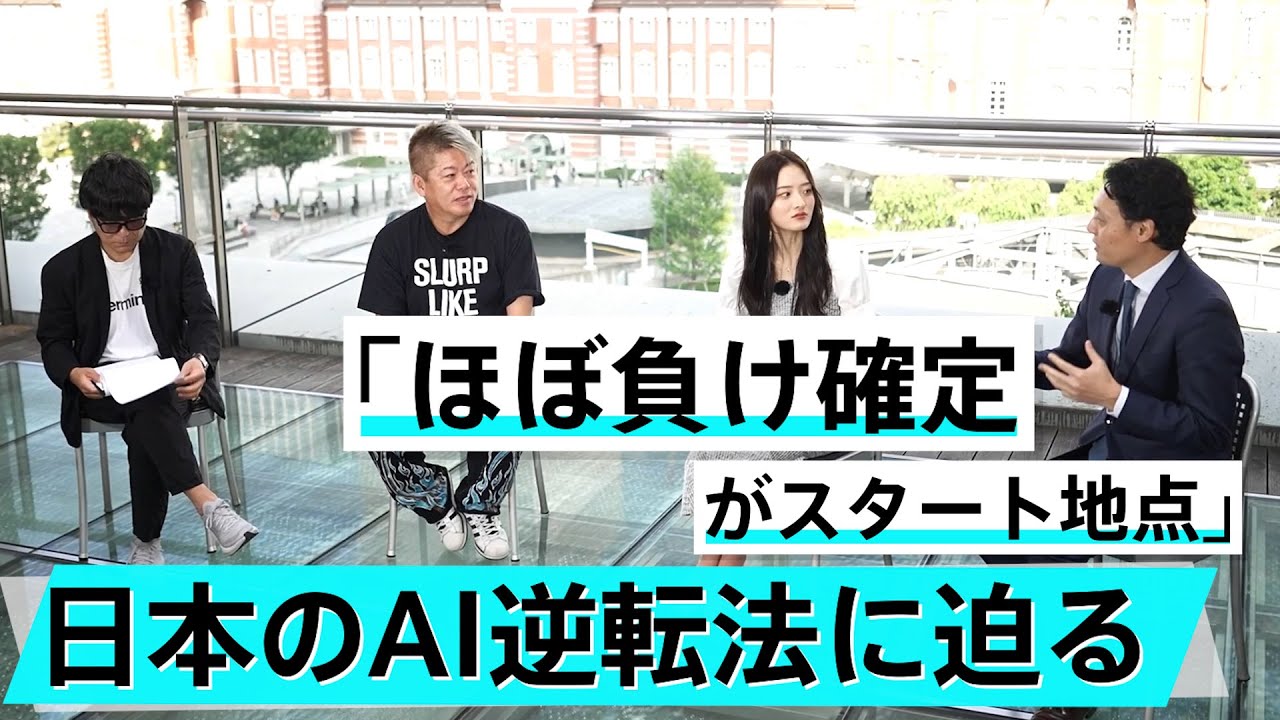
AIならアインシュタイン級の発見ができる?日本に残されたAI開発の逆転ストーリー【松尾豊×堀江貴文】
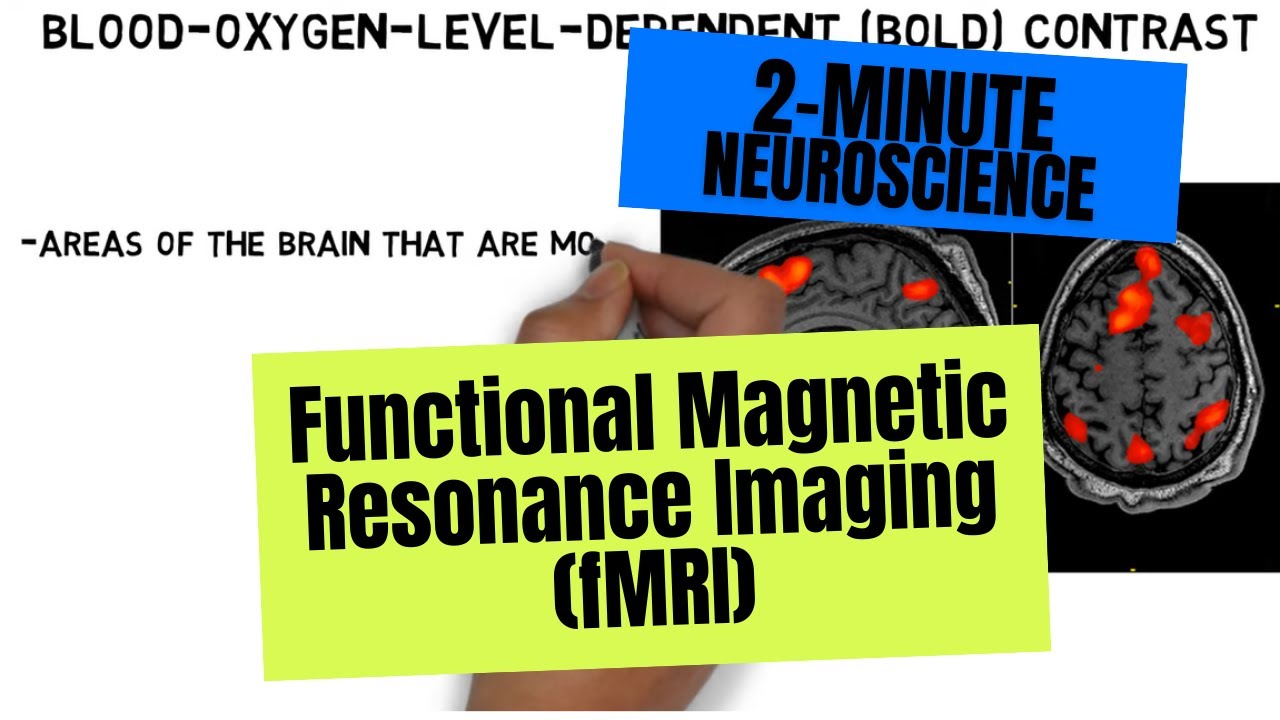
2-Minute Neuroscience: Functional Magnetic Resonance Imaging (fMRI)
5.0 / 5 (0 votes)