How the Ancients Predicted Eclipses 3,000 Years Ago
Summary
TLDRThis script explores the history and science of eclipse prediction, from the ancient Babylonians' discovery of the 18-year saros cycle to the Antikythera mechanism, an early analog computer. It discusses the challenges of the three-body problem involving Earth, moon, and sun, and how modern methods like NASA's JPL Ephemeris and Besselian elements allow for precise eclipse predictions. The script highlights the evolution from early astronomical records to advanced mathematical models, showcasing the pinnacle of predictive astronomy.
Takeaways
- 🌞 The first known analog computer was designed 2000 years ago to predict solar eclipses, a significant event in astronomy and science.
- 📚 Eclipse prediction has evolved over 3000 years, with modern science achieving accuracy to within a second or two for hundreds of years into the future.
- 🌐 Eclipse prediction involves solving the Three-Body Problem, which considers the complex motions of the Earth, Moon, and Sun.
- 📈 Ancient civilizations like the Babylonians kept detailed astronomical records, identifying patterns and cycles in the Moon's movements.
- 🌕 The synodic month, draconic month, and anomalistic month are three lunar cycles identified by ancient astronomers, crucial for understanding eclipses.
- 🔄 The saros, a period of approximately 18 years, was discovered by the Babylonians and is when the relative positions of the Sun, Earth, and Moon repeat, potentially leading to an eclipse.
- 🎓 Greek astronomers combined the saros with mathematical models to create the Antikythera mechanism, an early device for predicting astronomical positions and eclipses.
- 🚀 Modern methods of eclipse prediction involve numerical approximations and solving differential equations based on Newton's laws of motion and gravitation.
- 🌌 NASA uses the JPL Development Ephemeris, a mathematical model, and laser-ranging data from the Moon to predict the positions of celestial bodies with high precision.
- 📊 Besselian elements, a set of numbers, are used in conjunction with the JPL Ephemeris to predict the timing and visibility of eclipses on Earth's surface.
- 🔮 The saros series, though no longer the primary method for predicting eclipses, remains a significant tool for approximating them and is part of the ongoing story of human innovation in astronomy.
Q & A
What is the significance of the Antikythera mechanism mentioned in the script?
-The Antikythera mechanism is significant as it is considered the first known analog computer, designed around 2000 years ago to predict astronomical events, particularly solar eclipses. It was an early attempt to understand and predict the complex motions of celestial bodies using mechanical means.
How accurate are modern eclipse predictions compared to ancient methods?
-Modern eclipse predictions are extremely accurate, with the ability to predict when an eclipse will occur to within a second or two for many hundreds of years into the future. This level of precision is a result of thousands of years of scientific and mathematical advancements beyond the capabilities of ancient methods.
What is the 'Three-Body Problem' mentioned in the script?
-The 'Three-Body Problem' refers to the challenge of accurately predicting the motion of three celestial bodies that interact gravitationally, such as the Earth, Moon, and Sun. It is a complex problem in physics that has no general closed-form solution, but can be approximated numerically.
What are the three periodic cycles of the Moon that ancient astronomers observed?
-Ancient astronomers observed three periodic cycles of the Moon: the synodic month (29.5 days, from one new moon to the next), the draconic month (27.2 days, the time for the Moon to pass through the plane of the ecliptic at two different nodes), and the anomalistic month (27.5 days, the time for the Moon to return to the same size in the sky due to its elliptical orbit).
What is a 'saros' and how does it relate to eclipse prediction?
-A 'saros' is a period of approximately 18 years (6,585 days and 8 hours) after which the cycles of the Moon's phases, its size, and its position relative to the ecliptic plane align in a way that can produce an eclipse. The saros cycle was used by ancient astronomers to predict eclipses, although it could only approximate when an eclipse would occur, not where it would be visible.
How did the Babylonians contribute to the understanding of eclipses?
-The Babylonians contributed to the understanding of eclipses by keeping detailed astronomical records, known as astronomical diaries, which recorded the positions of celestial bodies. They identified patterns and periodic cycles, leading to the discovery of the saros cycle, which was a significant step in predicting eclipses.
What role did the saros cycle play in the development of the Antikythera mechanism?
-The saros cycle played a pivotal role in the development of the Antikythera mechanism as it was encoded into the device to help predict eclipses. The mechanism used the saros cycle to approximate the timing of eclipses, although it could not predict their visibility on Earth.
How do modern methods for predicting eclipses differ from using the saros cycle?
-Modern methods for predicting eclipses use advanced mathematical models and numerical approximations, such as the JPL Development Ephemeris, which takes into account the positions and velocities of celestial bodies with high precision. This differs from the saros cycle, which is a simpler, more approximate method that does not account for the exact positions of the Earth, Moon, and Sun.
What is the role of the Deep Space Network in determining the Earth's position relative to the Sun?
-The Deep Space Network, an array of spacecraft missions across the solar system, provides data that helps NASA determine the Earth's position relative to the Sun. This data is crucial for accurate eclipse predictions and is processed through complex mathematical models.
How is the position of the Moon determined with such precision today?
-The position of the Moon is determined with precision using laser ranging to reflective mirrors placed on the Moon by Apollo astronauts. Laser pulses are sent to these mirrors, and the time taken for the pulses to bounce back allows for the calculation of the distance between the Earth and the Moon with centimeter-scale accuracy.
Outlines
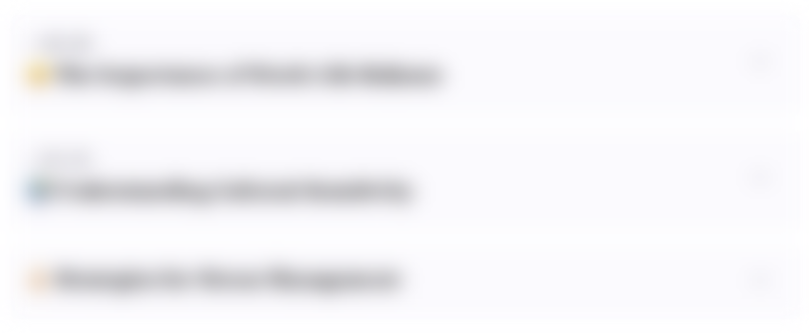
Cette section est réservée aux utilisateurs payants. Améliorez votre compte pour accéder à cette section.
Améliorer maintenantMindmap
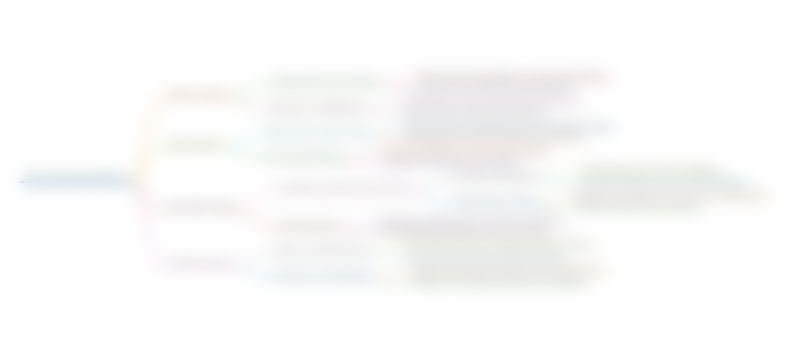
Cette section est réservée aux utilisateurs payants. Améliorez votre compte pour accéder à cette section.
Améliorer maintenantKeywords
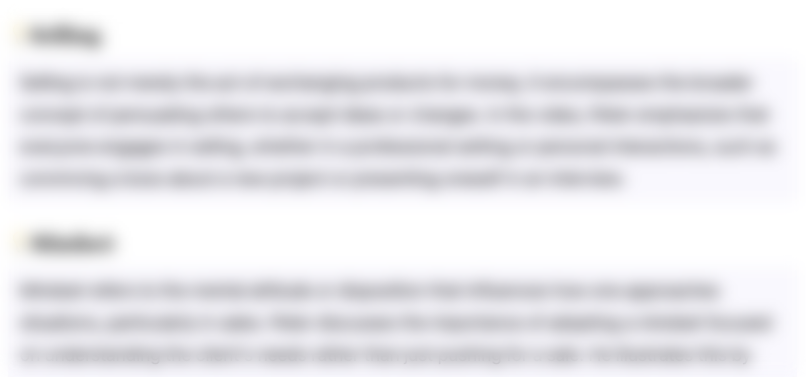
Cette section est réservée aux utilisateurs payants. Améliorez votre compte pour accéder à cette section.
Améliorer maintenantHighlights
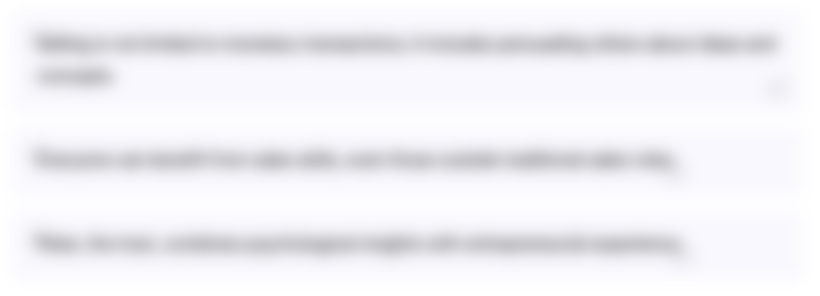
Cette section est réservée aux utilisateurs payants. Améliorez votre compte pour accéder à cette section.
Améliorer maintenantTranscripts
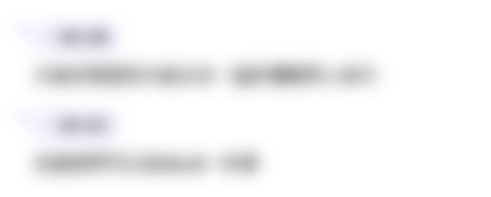
Cette section est réservée aux utilisateurs payants. Améliorez votre compte pour accéder à cette section.
Améliorer maintenantVoir Plus de Vidéos Connexes
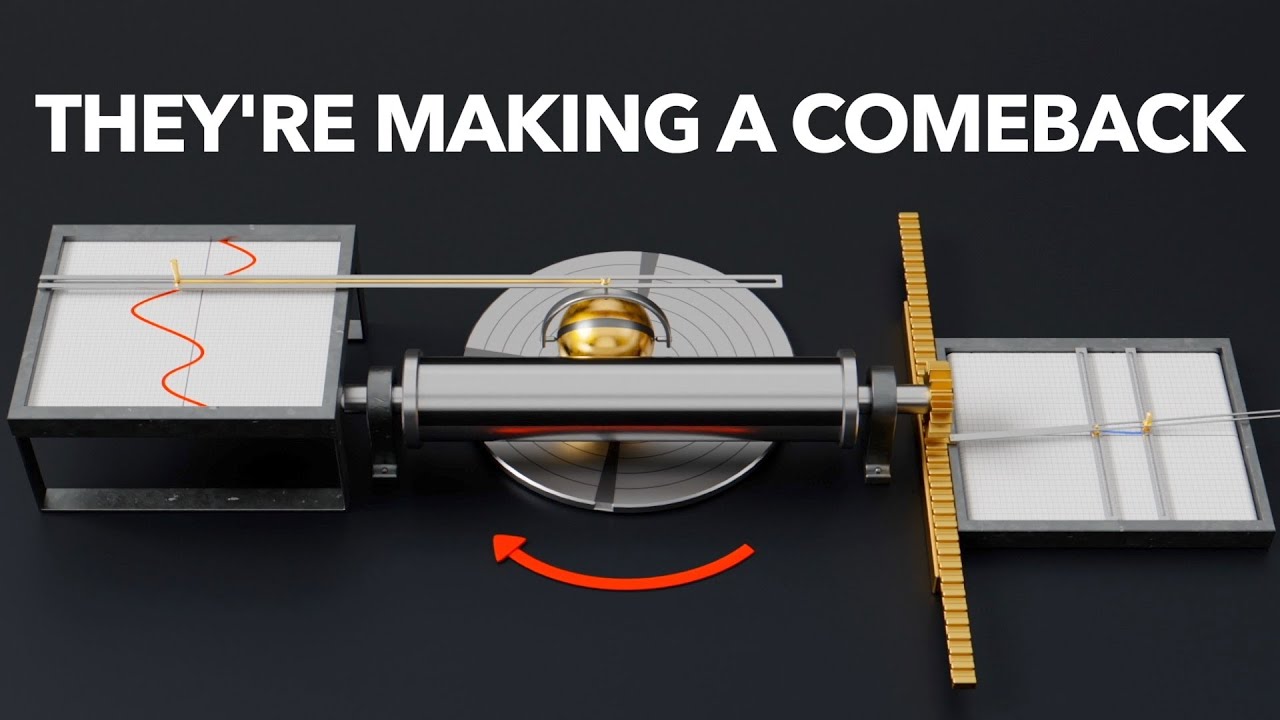
The Most Powerful Computers You've Never Heard Of
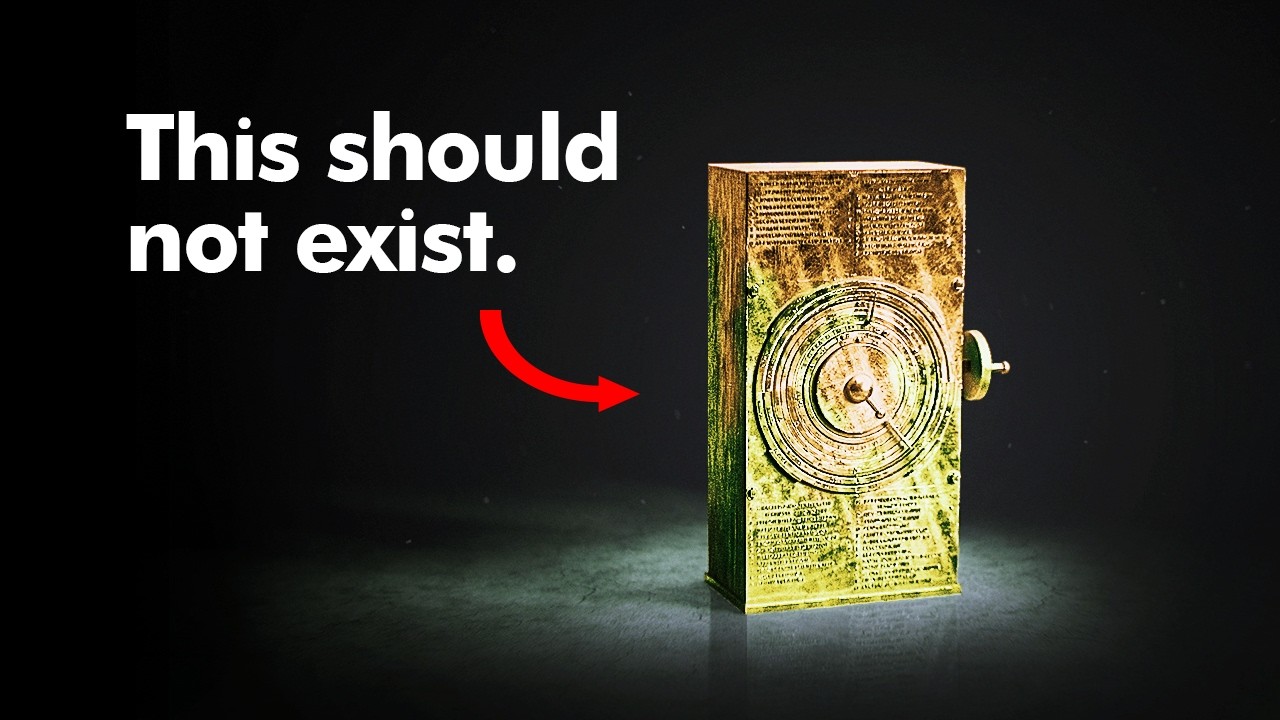
The Mystery of the World‘s First Computer
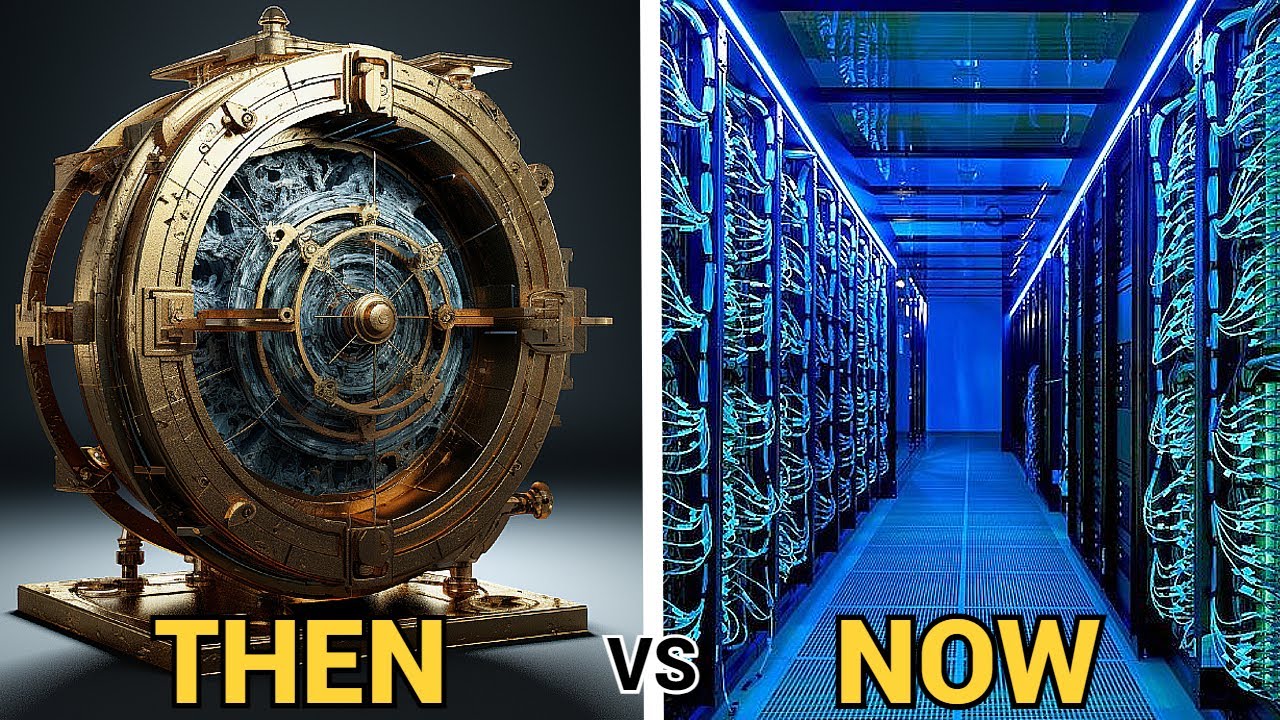
Ancient Greek Inventions That Are Still Used Today
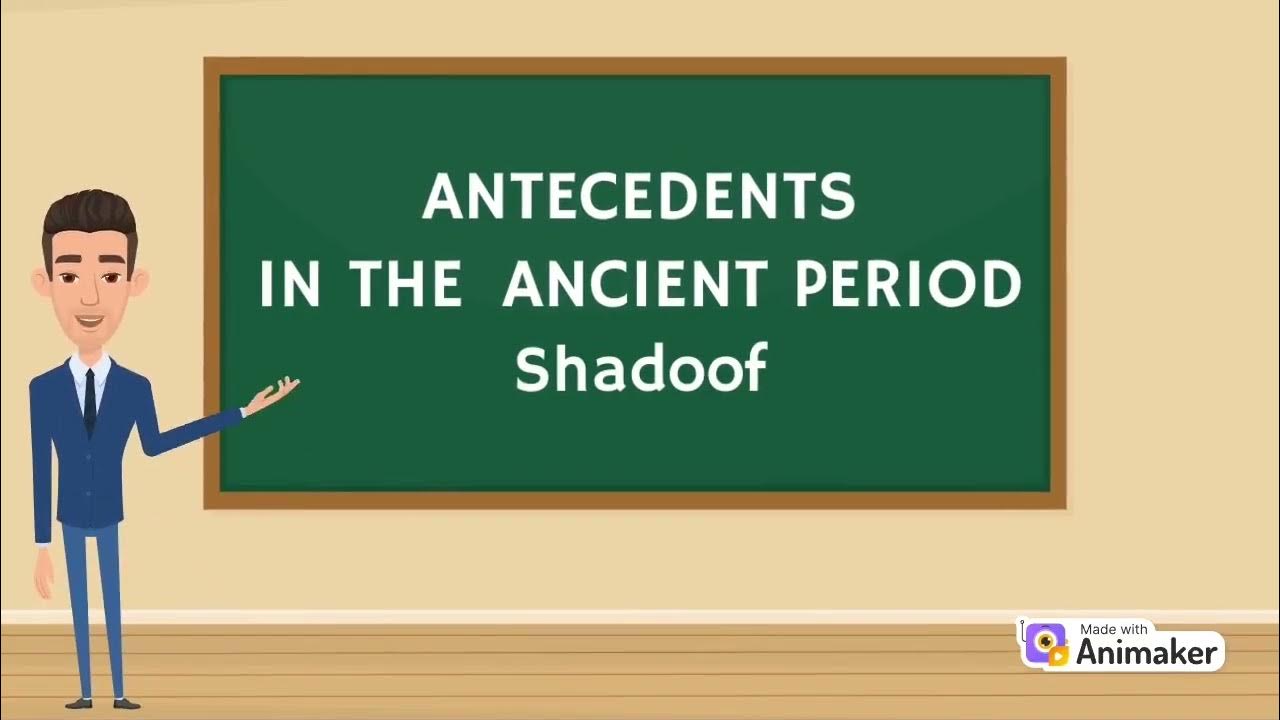
STS ANTECEDENTS IN THE ANCIENT PERIOD
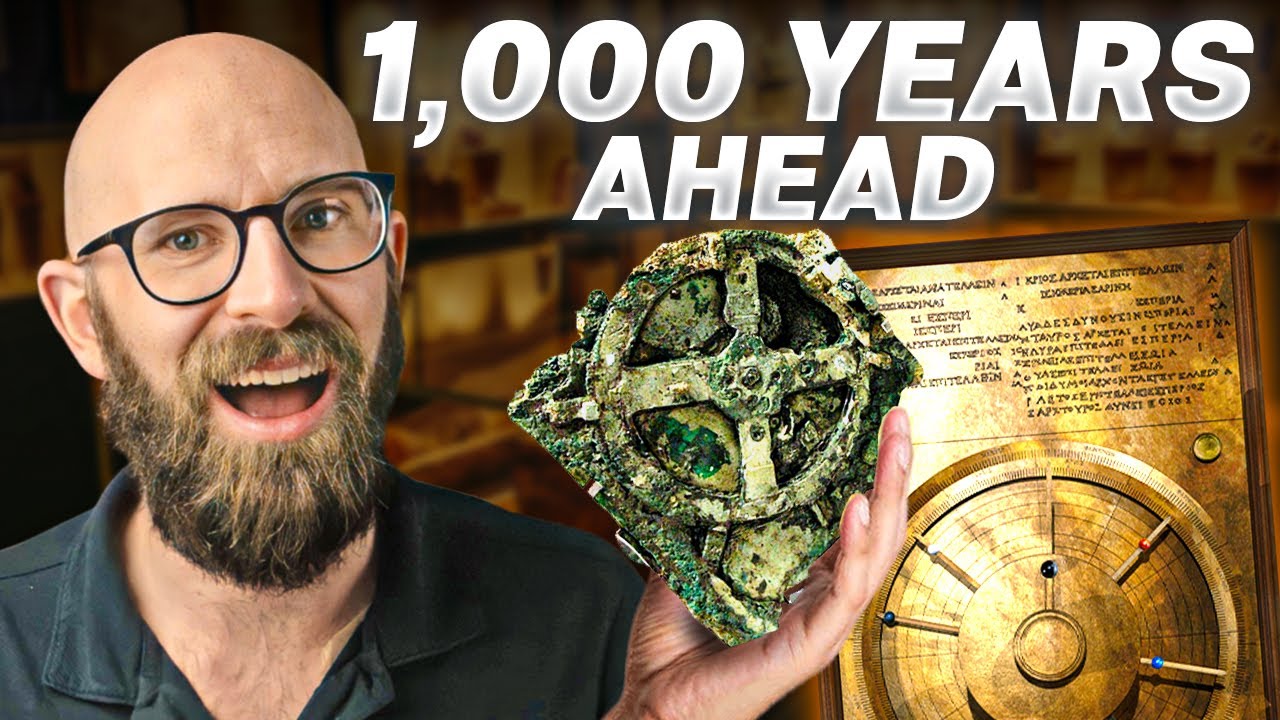
The Antikythera Mechanism: The Ancient Greek Super Computer
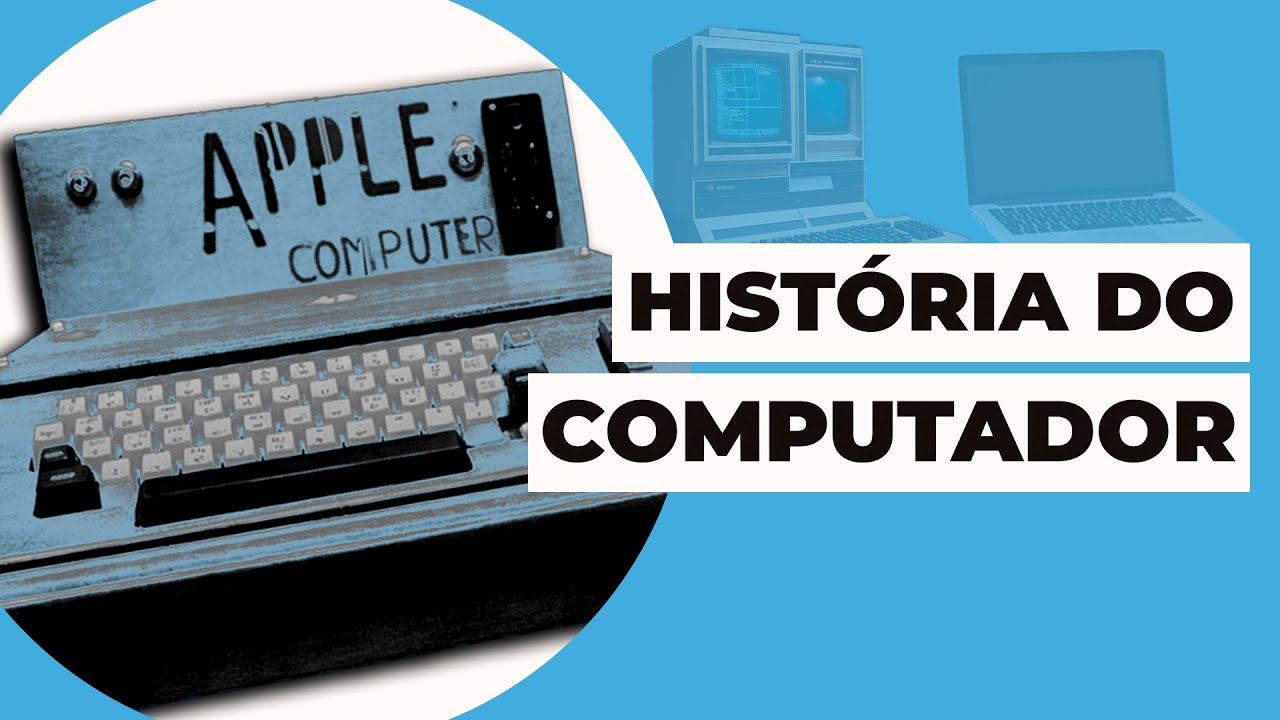
Conheça a história do computador e a sua evolução até os dias atuais
5.0 / 5 (0 votes)