IMAT Biology Lesson 6.9 | Anatomy and Physiology | Nervous System II
Summary
TLDRIn this educational video, Andre from Med School EU delves into the intricacies of the nervous system, focusing on nerve pathways and action potentials. He explains the autonomic nervous system's structure, highlighting the roles of the sympathetic and parasympathetic divisions. The video clarifies the concept of synapses and details the process of action potential generation, including depolarization and repolarization. Andre also dispels common misconceptions about action potentials, emphasizing that their size and speed remain constant regardless of stimulus intensity, and that frequency is the key variable distinguishing strong from weak stimuli. The explanation of synapse function and neurotransmitter release provides a comprehensive understanding of neural signal transmission.
Takeaways
- 🧠 The nervous system's nerve pathways and action potentials are the focus of the video, discussing how signals are transmitted along the system.
- 🤔 The autonomic nervous system, which is involuntarily controlled by the brain, is divided into the parasympathetic and sympathetic divisions for controlling organ activity.
- 🌐 The preganglionic neuron originates from the central nervous system and synapses at the autonomic ganglion, while the postganglionic neuron transmits signals to the organs.
- 🔋 Myelinated preganglionic fibers have a myelin sheath composed of Schwann cells, which speed up signal transmission through nodes of Ranvier.
- ⚡ The action potential involves a rapid change in membrane potential from a resting state of -70 millivolts to a peak of +30 millivolts and back, facilitated by the opening and closing of sodium and potassium ion channels.
- 🚀 The speed of an action potential depends on the diameter of the axon and whether it is myelinated, with larger diameters and myelination increasing the speed of transmission.
- 🔗 The synapse is the connection between neurons, where neurotransmitters are released from the presynaptic neuron and bind to receptors on the postsynaptic neuron.
- 🔑 Neurotransmitters like acetylcholine and norepinephrine are chemical signals that transmit information across the synapse.
- 🔄 The sodium-potassium pump maintains the resting membrane potential and is essential for the action potential's depolarization and repolarization phases.
- 🌀 The action potential's size remains constant regardless of the stimulus intensity, but the frequency of action potentials can vary depending on the stimulus strength.
- 🏎️ Saltatory conduction, the process of signal jumping between nodes of Ranvier in myelinated axons, significantly increases the speed of signal transmission.
Q & A
What is the main topic of the video?
-The main topic of the video is the nerve pathway and action potentials of the nervous system.
What is the autonomic nervous system and how is it controlled?
-The autonomic nervous system is the part of the nervous system that is involuntarily controlled. It is completely controlled by the brain and operates automatically without conscious awareness.
What are the two divisions of the autonomic nervous system discussed in the video?
-The two divisions of the autonomic nervous system discussed are the parasympathetic nervous system (PNS) and the sympathetic nervous system (SNS).
What is the role of the preganglionic neuron in the nervous system?
-The preganglionic neuron is the part of the nervous system that comes off the spinal cord or the central nervous system. It is myelinated and plays a crucial role in transmitting signals to the autonomic ganglion.
Why are preganglionic fibers myelinated?
-Preganglionic fibers are myelinated because the myelin sheath, composed of Schwann cells, provides insulation for these fibers, allowing them to transmit signals much faster.
What are the neurotransmitters mentioned in the video that are used to transmit signals in the autonomic nervous system?
-The neurotransmitters mentioned in the video that are used to transmit signals in the autonomic nervous system are acetylcholine and norepinephrine.
How does the resting action potential of a neuron help in maintaining the membrane potential?
-The resting action potential is maintained by the sodium-potassium pump, which actively transports three sodium ions out of the cell and two potassium ions into the cell, keeping the membrane potential at around negative 70 millivolts.
What is the significance of the threshold potential in the generation of an action potential?
-The threshold potential is significant because it is the point at which an action potential is triggered. If the membrane potential reaches above negative 50 millivolts, it passes the threshold, leading to the opening of voltage-gated sodium channels and the initiation of an action potential.
What are the key steps in the process of an action potential?
-The key steps in an action potential are depolarization (influx of sodium ions), reaching the peak potential (positive 30 millivolts), repolarization (closing of sodium channels and opening of potassium channels), and hyperpolarization (overshoot below negative 70 millivolts due to potassium ion outflow).
How does the speed of an action potential transmission vary with the stimulus intensity?
-The speed of an action potential transmission does not vary with the stimulus intensity. The size and speed of action potentials remain constant regardless of the stimulus strength; it is the frequency of action potentials that increases with stronger stimuli.
What is the role of synapses in the nervous system?
-Synapses are the connections between neurons that allow for the transmission of signals from one neuron to another. They involve the release of neurotransmitters from the presynaptic neuron and the binding of these neurotransmitters to receptors on the postsynaptic neuron, potentially causing an action potential in the latter.
Outlines
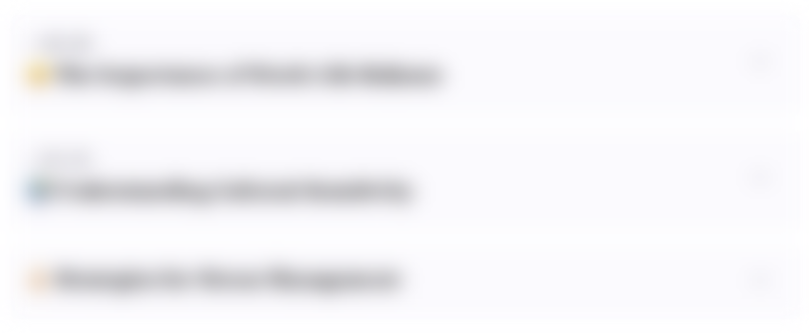
Esta sección está disponible solo para usuarios con suscripción. Por favor, mejora tu plan para acceder a esta parte.
Mejorar ahoraMindmap
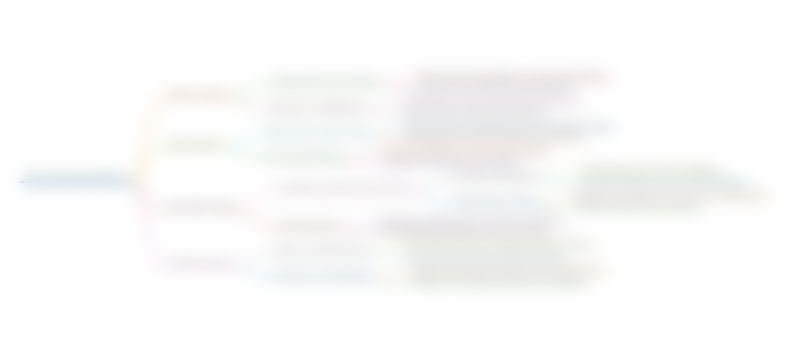
Esta sección está disponible solo para usuarios con suscripción. Por favor, mejora tu plan para acceder a esta parte.
Mejorar ahoraKeywords
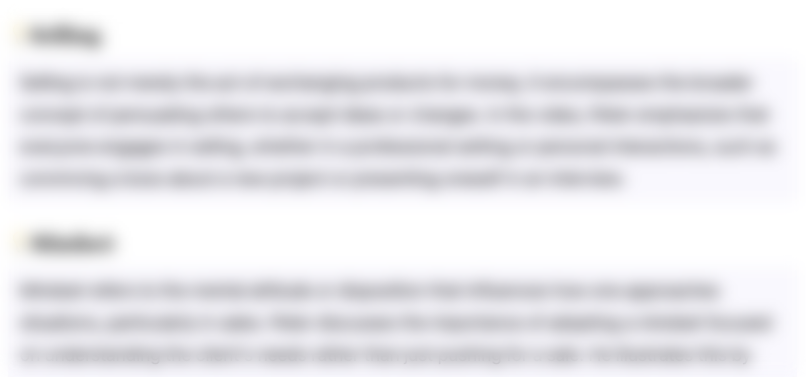
Esta sección está disponible solo para usuarios con suscripción. Por favor, mejora tu plan para acceder a esta parte.
Mejorar ahoraHighlights
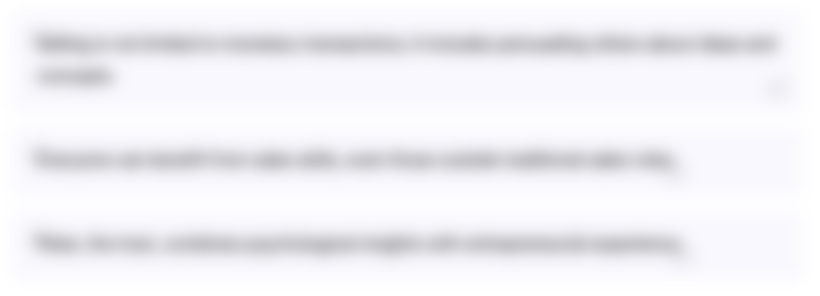
Esta sección está disponible solo para usuarios con suscripción. Por favor, mejora tu plan para acceder a esta parte.
Mejorar ahoraTranscripts
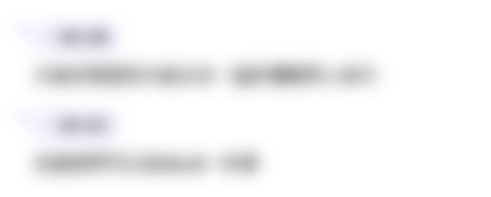
Esta sección está disponible solo para usuarios con suscripción. Por favor, mejora tu plan para acceder a esta parte.
Mejorar ahoraVer Más Videos Relacionados
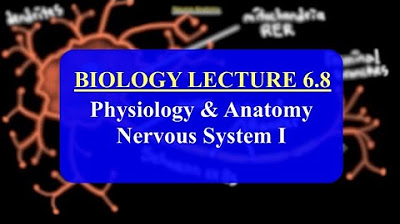
IMAT Biology Lesson 6.8 | Anatomy and Physiology | Nervous System I

IMAT Biology Lesson 6.1 | Anatomy and Physiology | Animal Tissues Part I

IMAT Biology Lesson 6.14 | Anatomy and Physiology | Digestive System I
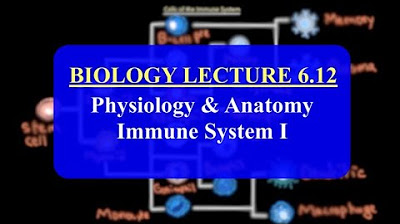
IMAT Biology Lesson 6.12 | Anatomy and Physiology | Immune System Part I
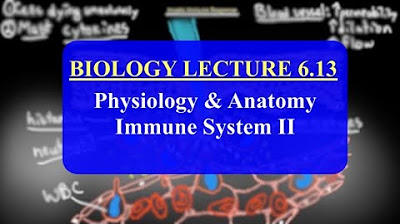
IMAT Biology Lesson 6.13 | Anatomy and Physiology | Immune System Part II

IMAT Biology Lesson 6.10 | Anatomy and Physiology | Muscle Contraction
5.0 / 5 (0 votes)