Low Level Laser Therapy (LLLT): What it is, how it is applied and an overview of the research.
Summary
TLDRThis lecture covers the principles and applications of low-level laser therapy (LLLT), an electro-therapeutic modality that uses lasers to relieve pain and stimulate cell function. The lecture explains how lasers work, emphasizing key properties such as monochromaticity, coherence, and collimation. It also describes how factors like wavelength, power, and fluence influence tissue interaction. Despite its growing use in treating musculoskeletal conditions, evidence for LLLT’s effectiveness remains inconsistent, with conflicting research results across various conditions, including tendinopathy, neck pain, and low back pain. Ongoing research aims to clarify its therapeutic potential.
Takeaways
- 🔬 Lasers produce light through stimulated emission of radiation, and are characterized by coherent, monochromatic light with minimal divergence.
- 💡 Low-level laser therapy (LLLT) is used in physiotherapy to relieve pain and enhance cell function, though its mechanisms and effectiveness are still under research.
- 📜 LLLT is theorized to follow the Grotthus-Draper law, where light must be absorbed to trigger photochemical reactions, potentially boosting ATP production through enzymes like cytochrome C oxidase.
- ⚡ The unique properties of lasers include monochromaticity (same wavelength), coherence (synchronized photons), and collimation (nearly parallel beams).
- 📏 Various factors influence laser interactions with tissues, including wavelength, power, pulse rate, and spot size, all of which affect penetration and energy absorption.
- 📊 LLLT parameters such as energy density, power output, and pulse rate are adjustable, and machines used in therapy typically operate in the 600-1000 nm wavelength range for deeper tissue penetration.
- 🧪 Evidence on the effectiveness of LLLT is mixed, with studies on conditions like tendinopathy and low back pain showing inconsistent results.
- 🧠 Placebo effects may play a role in LLLT’s perceived benefits, emphasizing the need for high-quality randomized controlled trials in research.
- 📝 Systematic reviews on LLLT for conditions such as rheumatoid arthritis, osteoarthritis, and frozen shoulder show conflicting results, often due to study bias or varying methodologies.
- 🧑⚕️ LLLT treatment protocols differ by condition, with recommendations such as 8 joules per square centimeter for Achilles tendinopathy, though treatment effectiveness can vary significantly.
Q & A
What is a laser and how does it function?
-A laser is a device that amplifies light through stimulated emission of radiation to produce a coherent, monochromatic beam. It consists of an energy source, a resonant chamber, and an active medium. Electrons in the active medium are excited and emit photons when they return to their ground state. These photons reflect between mirrors in the chamber and exit as a focused beam directed at a target tissue.
What are the unique properties of laser light compared to conventional light?
-Laser light has three unique properties: monochromaticity (all photons have the same wavelength), coherence (photons are synchronized in time and space), and collimation (laser beams are nearly parallel and can be focused to a small area with little divergence).
How does low-level laser therapy (LLLT) theoretically work?
-LLLT works on the principle of the Grotthus Draper law, which states that light must be absorbed by a chemical substance for a photochemical reaction to occur. LLLT is believed to stimulate cellular processes like oxidative phosphorylation and the production of ATP by affecting enzymes such as cytochrome C oxidase in mitochondria.
What are some of the factors that influence how a laser interacts with tissue?
-Several factors influence laser-tissue interaction: the wavelength of the laser, the power and power density, pulse duration, spot size, fluence (energy density), properties of the target tissue, and the duration of exposure. Longer wavelengths penetrate deeper into tissues, and energy density affects the intensity of the treatment.
How is energy density calculated in laser therapy?
-Energy density (fluence) is calculated by dividing the total energy (in joules) by the area of application (in square centimeters). The energy itself is determined by multiplying the power (in watts) by the treatment time.
What is the role of pulse duration in low-level laser therapy?
-Pulse duration (or pulse rate) is crucial for determining how the laser energy is delivered. For more acute and superficial injuries, lower pulse rates are used, while chronic conditions typically require higher pulse rates. The pulse duration is related to the thermal relaxation time, which is the time it takes for tissue to return to its baseline temperature after being heated.
What evidence exists for the effectiveness of low-level laser therapy?
-The evidence for LLLT's effectiveness is mixed and often of variable quality. Some studies show benefits for conditions like tendinopathy and low back pain, while others find no significant effects. Systematic reviews often highlight inconsistencies and methodological flaws in existing research.
What are some of the common applications of low-level laser therapy?
-LLLT is commonly applied to treat musculoskeletal conditions, such as tendinopathies, low back pain, neck pain, and frozen shoulder. However, the efficacy of treatment varies depending on the condition and the parameters used in the therapy.
How do the wavelength and spot size affect tissue penetration in laser therapy?
-Wavelength determines the depth of tissue penetration; longer wavelengths penetrate more deeply. Spot size also affects energy delivery, with larger spot sizes leading to less scattering and deeper penetration compared to smaller spot sizes.
What are the primary challenges in conducting research on low-level laser therapy?
-Challenges in LLLT research include variability in treatment protocols (wavelength, power, duration), difficulty controlling for the placebo effect, and the lack of high-quality randomized control trials. Many studies also suffer from low quality, small sample sizes, and inconsistent results.
Outlines
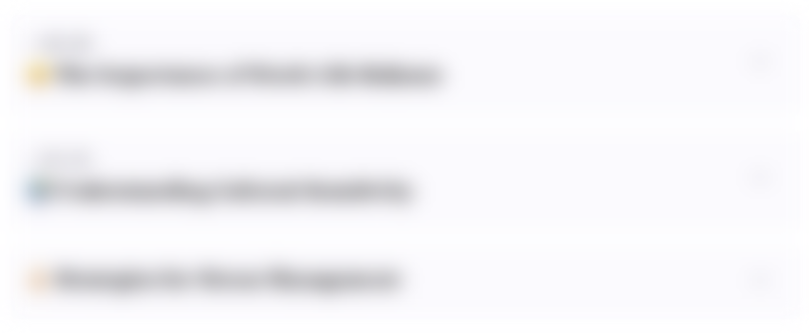
Esta sección está disponible solo para usuarios con suscripción. Por favor, mejora tu plan para acceder a esta parte.
Mejorar ahoraMindmap
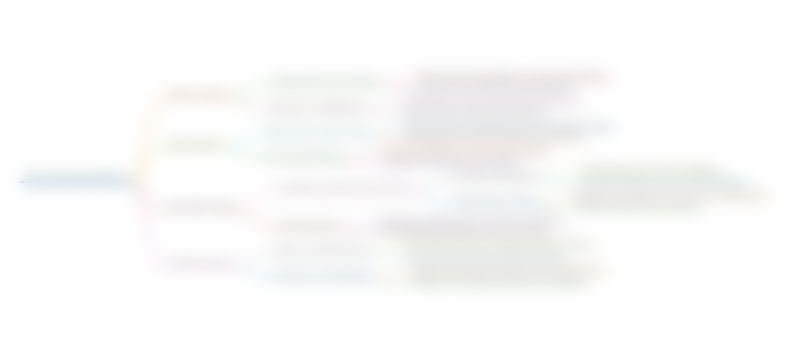
Esta sección está disponible solo para usuarios con suscripción. Por favor, mejora tu plan para acceder a esta parte.
Mejorar ahoraKeywords
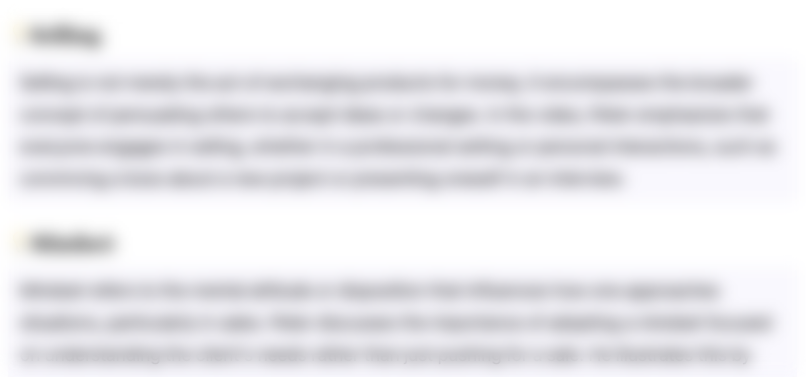
Esta sección está disponible solo para usuarios con suscripción. Por favor, mejora tu plan para acceder a esta parte.
Mejorar ahoraHighlights
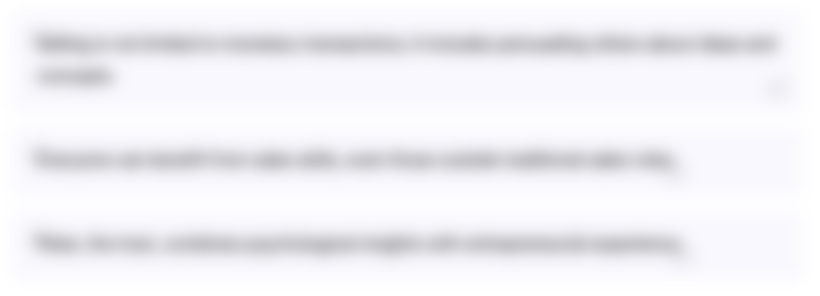
Esta sección está disponible solo para usuarios con suscripción. Por favor, mejora tu plan para acceder a esta parte.
Mejorar ahoraTranscripts
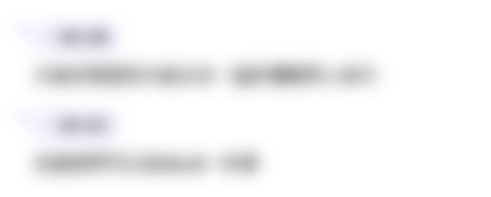
Esta sección está disponible solo para usuarios con suscripción. Por favor, mejora tu plan para acceder a esta parte.
Mejorar ahora5.0 / 5 (0 votes)