Finite wing effects [Aerodynamics #15]
Summary
TLDRThis aerodynamics video delves into the effects of finite span wings and wing tips on an aerodynamic body. It explains the concept of induced drag, effective angle of attack, and downwash caused by the tip vortex. The script introduces Biot-Savart's law to calculate the induced velocity of a semi-infinite vortex, which is crucial for understanding lift distribution and performance impacts on real-world aircraft. Practical implications include the design of wing tips to mitigate vortex effects and the preference for high aspect ratio wings for efficiency.
Takeaways
- 🛫 The script introduces the concept of drag on aerodynamic bodies, explaining that it's primarily due to viscous forces but can be dominated by pressure drag when flow separates quickly.
- 📚 It explains that the ideal two-dimensional (2D) wing principles don't fully apply to real-world three-dimensional (3D) wings, leading to the exploration of finite span wings and their effects.
- 📉 The script discusses the performance of a NACA 4412 airfoil section, highlighting the difference between 2D ideal sections and 3D real-world applications, particularly the change in lift and drag coefficients.
- 🔁 The concept of end effects is introduced, particularly focusing on the tip vortex and downwash, which are caused by the pressure difference near the wing edges and roll around the tips, affecting the wing's performance.
- 🌀 The tip vortex is explained as a result of the flow rolling from underneath to the top of the wing, creating a trailing vortex in the wake and influencing the wing's angle of attack and lift.
- 📊 The script differentiates between total lift and drag coefficients and those per unit span, emphasizing that they are not necessarily equal due to finite span effects.
- 📉 Induced drag is introduced as a new form of drag that occurs when the lift vector is tilted due to downwash, reducing lift efficiency and adding to the overall drag.
- 📐 The script discusses lift distribution across the span of a wing, noting that it varies and is influenced by factors such as the tip vortex and the design of the wing.
- 🔧 Practical applications of understanding tip vortices are highlighted, including their impact on aircraft performance, frequency of takeoffs and landings, and the development of wingtip devices to mitigate their effects.
- 📚 Biot-Savart Law is introduced as a tool for analyzing the induced velocity of a vortex, with a detailed explanation of how it applies to the semi-infinite vortex representing the tip vortex.
- 🌐 The script concludes with a discussion of the Helmholtz vortex theorems, which describe the behavior of vortex filaments in fluid dynamics, and their relevance to understanding aerodynamic effects.
Q & A
What are the two mechanisms through which force is transmitted from a fluid to an aerodynamic body?
-The two mechanisms are pressure, which acts normal to the surface, and shear, which is parallel to the surface.
Why is the drag of an aerodynamic body typically due to viscous forcing?
-The drag is mostly due to viscous forcing because of the friction between the fluid and the body's surface. However, if flow separates quickly, it becomes dominated by pressure drag.
What is the primary focus of the video regarding the effects that come with the wing tip?
-The primary focus is on the tip vortex and the downwash it produces, which leads to a change in the effective angle of attack and adds induced drag.
How does the lift coefficient and drag coefficient change when considering a 3D wing compared to a 2D ideal section?
-In a 3D wing, the lift and drag coefficients are different from those of a 2D ideal section due to finite span effects, such as the tip vortex and downwash, which alter the performance.
What is the significance of the tip vortex in the context of aerodynamics?
-The tip vortex is significant because it induces a downwash that changes the effective angle of attack on the wing, leading to a decrease in lift and an increase in induced drag.
What is the relationship between the lift per unit span and the total lift force?
-The lift per unit span is calculated using the lift divided by the span, whereas the total lift force uses the total lift with the addition of span in the denominator.
Why does the lift distribution across the span of a wing vary?
-The lift distribution varies because near the tips, the flow can travel from the bottom to the top, balancing out the pressure and reducing the pressure difference from the bottom and top of the foil.
What is the Biot-Savart Law and how is it applied in this context?
-The Biot-Savart Law describes the induced velocity on a point due to a segment of a vortex filament. It is applied here to calculate the downwash effect caused by the tip vortex on the wing.
How does the tip vortex influence the performance of a finite span wing?
-The tip vortex induces a downwash that changes the effective angle of attack, decreases lift, and adds induced drag, which negatively impacts the wing's performance.
What are the practical impacts of the tip vortex on aircraft operations?
-The tip vortex affects the frequency that aircraft can take off and land at airports, influences technology innovation to avoid the negative effects of the vortex, and encourages the design of high aspect ratio wings for more efficient flight.
What are the Helmholtz vortex theorems and why are they important in understanding vortex behavior?
-The Helmholtz vortex theorems are rules stating that vortex filament strength is constant along the filament, a vortex cannot end arbitrarily in a fluid but must terminate at a solid boundary or form a closed loop, and irrotational flow remains irrotational without external forcing. These theorems are important for predicting and describing the effects of vortices like the tip vortex.
Outlines
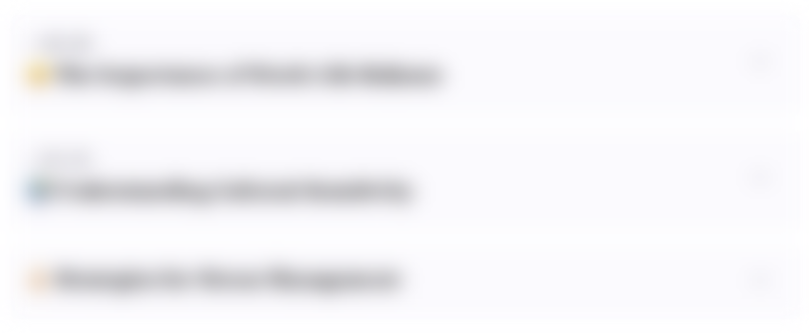
Dieser Bereich ist nur für Premium-Benutzer verfügbar. Bitte führen Sie ein Upgrade durch, um auf diesen Abschnitt zuzugreifen.
Upgrade durchführenMindmap
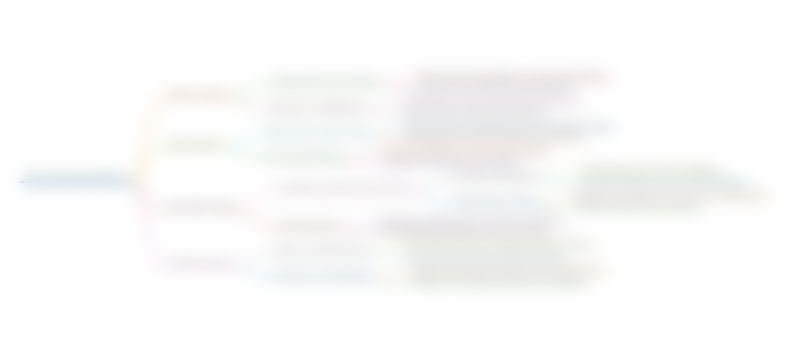
Dieser Bereich ist nur für Premium-Benutzer verfügbar. Bitte führen Sie ein Upgrade durch, um auf diesen Abschnitt zuzugreifen.
Upgrade durchführenKeywords
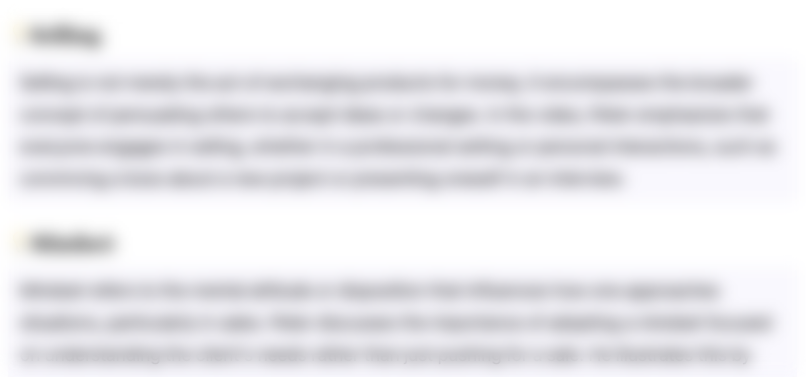
Dieser Bereich ist nur für Premium-Benutzer verfügbar. Bitte führen Sie ein Upgrade durch, um auf diesen Abschnitt zuzugreifen.
Upgrade durchführenHighlights
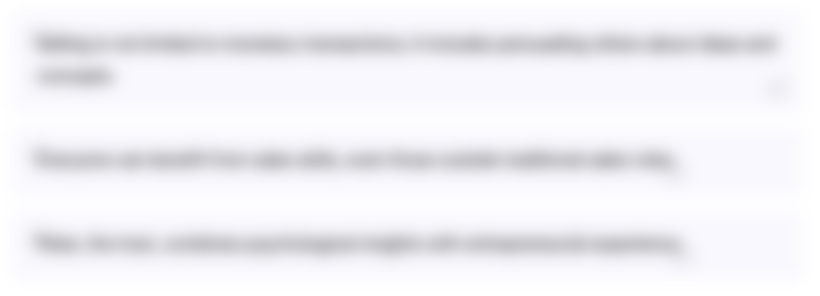
Dieser Bereich ist nur für Premium-Benutzer verfügbar. Bitte führen Sie ein Upgrade durch, um auf diesen Abschnitt zuzugreifen.
Upgrade durchführenTranscripts
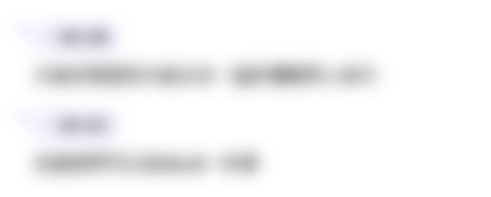
Dieser Bereich ist nur für Premium-Benutzer verfügbar. Bitte führen Sie ein Upgrade durch, um auf diesen Abschnitt zuzugreifen.
Upgrade durchführen5.0 / 5 (0 votes)