Ligand Field Theory and the Jahn-Teller Effect
Summary
TLDRThe script delves into the bonding and properties of transition metal complexes, focusing on concepts like VSEPR and crystal field theory. It introduces ligand field theory, which expands on crystal field theory to predict properties of transition metal complexes using molecular orbital theory. The discussion highlights the Jahn-Teller effect, a distortion that occurs in transition metal complexes to remove degeneracy in unequally occupied orbitals, leading to stability changes. The script also explains how electron arrangements in d orbitals can lead to distortions such as tetragonal elongation or compression, crucial for understanding the geometry of these complexes.
Takeaways
- 🔬 Transition metal complexes require an understanding of bonding theories and molecular geometry.
- 📚 VSEPR theory helps explain simple molecular geometries through electron cloud repulsion.
- 🧪 Crystal field theory explains how d orbitals in transition metal complexes are split in energy when coordinating ligands.
- ⚡ Ligand field theory, an extension of crystal field theory, uses molecular orbital theory to predict bonding and properties in transition metal complexes.
- 🌟 In ligand field theory, 9 valence orbitals from a metal (s, p, and d) overlap with ligand orbitals to form molecular orbitals, affecting bonding and stability.
- 📉 The Jahn-Teller effect occurs when asymmetrically filled orbitals in a complex distort the geometry to reduce energy.
- ⚖️ Octahedral complexes with d-electrons may experience tetragonal distortion depending on the electron configuration in eg or t2g orbitals.
- 📐 High spin d4, low spin d7, or d9 configurations often trigger Jahn-Teller distortions.
- 🔄 Distortions can lead to tetragonal compression or elongation depending on the orbital stabilization, particularly in eg orbitals.
- 🔍 The Jahn-Teller effect's influence on molecular geometry is important for understanding the properties and reactions of transition metal complexes.
Q & A
What is the crystal field splitting energy?
-The crystal field splitting energy is the energy difference between the t2g (lower energy) and eg (higher energy) orbitals that arise when transition metal d orbitals interact with ligands, causing them to no longer be degenerate.
How does ligand field theory differ from crystal field theory?
-Ligand field theory is an extension of crystal field theory and incorporates molecular orbital theory. It more accurately predicts properties of transition metal complexes, such as those gathered from spectral analysis, by considering bonding interactions between the metal's s, p, and d orbitals and ligand orbitals.
What are bonding, antibonding, and nonbonding orbitals in ligand field theory?
-In ligand field theory, bonding orbitals are lower energy molecular orbitals formed from the overlap of metal and ligand atomic orbitals, while antibonding orbitals are higher energy. Nonbonding orbitals remain at the same energy as the original d orbitals of the metal.
What is the Jahn-Teller effect?
-The Jahn-Teller effect describes the distortion of a transition metal complex to lower its energy by removing the degeneracy of unequally occupied orbitals. This typically occurs in nonlinear molecules, especially when d orbitals are asymmetrically filled.
In which cases will the Jahn-Teller effect not be observed?
-The Jahn-Teller effect will not be observed if the d orbitals are symmetrically filled, such as in cases where either the t2g or eg orbitals are half-full or completely full.
How does Jahn-Teller distortion affect the geometry of a complex?
-Jahn-Teller distortion can cause a complex to either stretch or compress along the z-axis, depending on whether the eg or t2g orbitals are affected. If the asymmetry occurs in the eg orbitals, the distortion is strong, while asymmetry in the t2g orbitals causes weaker distortions.
What happens in a d4 high spin complex during Jahn-Teller distortion?
-In a d4 high spin complex, one electron in the eg orbitals will cause stabilization, either by compressing or elongating the metal-ligand bonds along the z-axis, depending on whether the dx2-y2 or dz2 orbital is lower in energy.
Why is there no Jahn-Teller distortion in a d5 high spin complex?
-In a d5 high spin complex, all the orbitals are half-full, and when distributed among the new orbitals after distortion, there is no net energy change, resulting in no Jahn-Teller distortion.
What role do metal-ligand bonds play in ligand field theory?
-In ligand field theory, metal-ligand bonds form through the overlap of metal's s, p, and d orbitals with the ligand orbitals, producing bonding, antibonding, and nonbonding molecular orbitals that influence the stability and geometry of the complex.
What is the significance of the dx2-y2 and dz2 orbitals in an octahedral complex?
-In an octahedral complex, the dx2-y2 and dz2 orbitals (eg orbitals) are higher in energy compared to the t2g orbitals, and their degeneracy can be broken by Jahn-Teller distortion, leading to different energy distributions and affecting the geometry of the complex.
Outlines
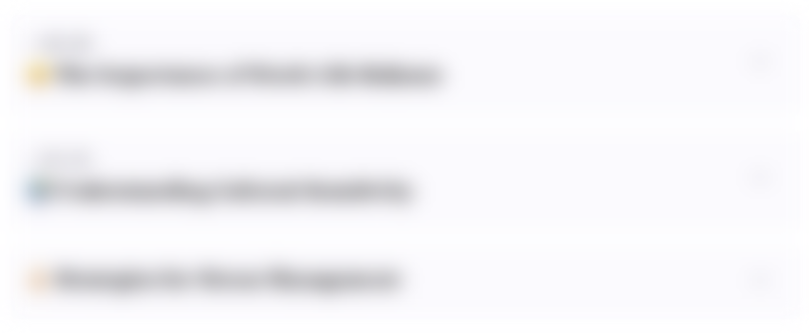
هذا القسم متوفر فقط للمشتركين. يرجى الترقية للوصول إلى هذه الميزة.
قم بالترقية الآنMindmap
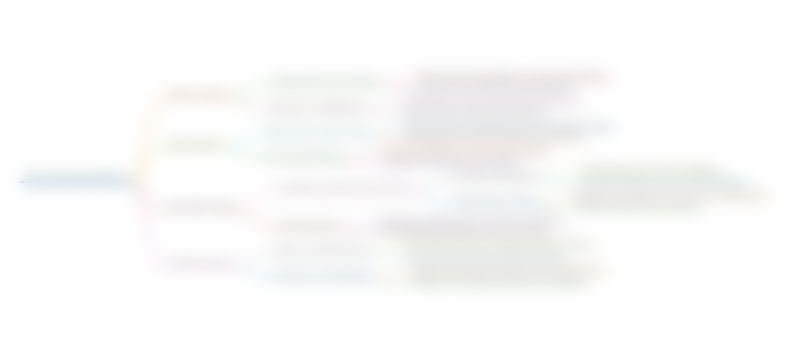
هذا القسم متوفر فقط للمشتركين. يرجى الترقية للوصول إلى هذه الميزة.
قم بالترقية الآنKeywords
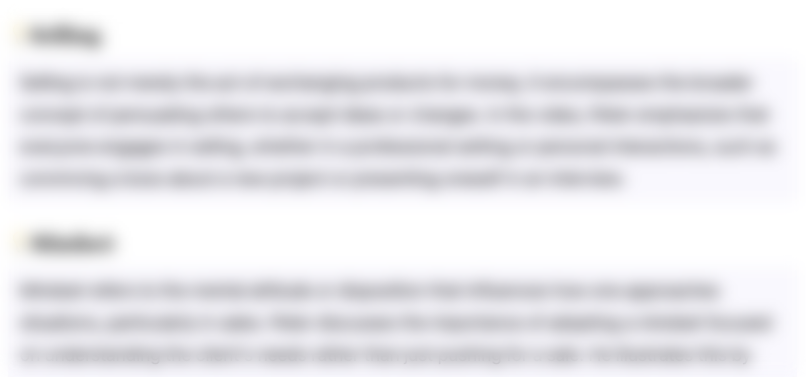
هذا القسم متوفر فقط للمشتركين. يرجى الترقية للوصول إلى هذه الميزة.
قم بالترقية الآنHighlights
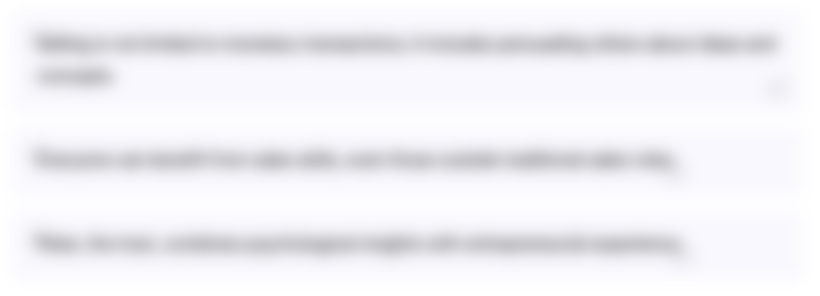
هذا القسم متوفر فقط للمشتركين. يرجى الترقية للوصول إلى هذه الميزة.
قم بالترقية الآنTranscripts
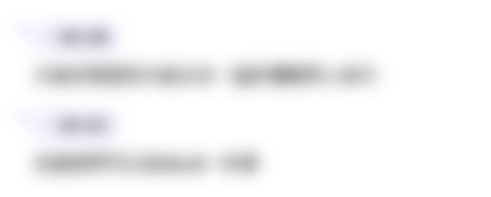
هذا القسم متوفر فقط للمشتركين. يرجى الترقية للوصول إلى هذه الميزة.
قم بالترقية الآنتصفح المزيد من مقاطع الفيديو ذات الصلة
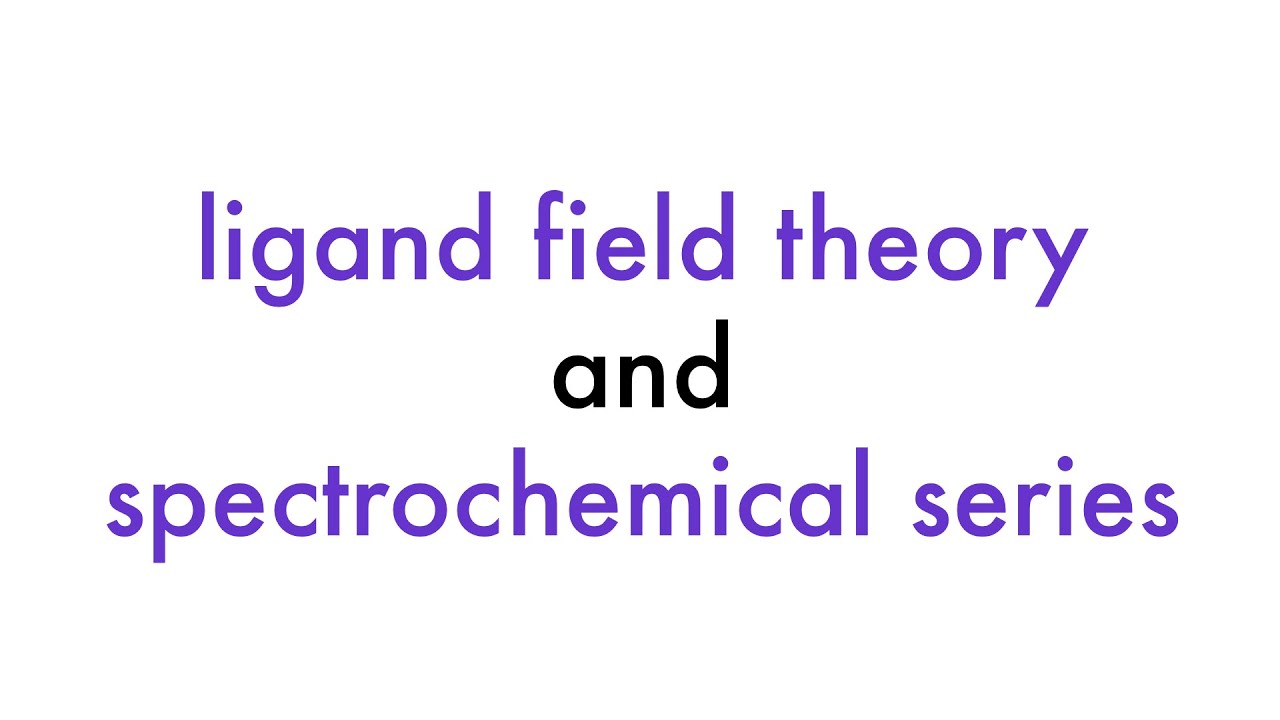
Ligand Field Theory and Spectrochemical Series | Professor Adam Teaches
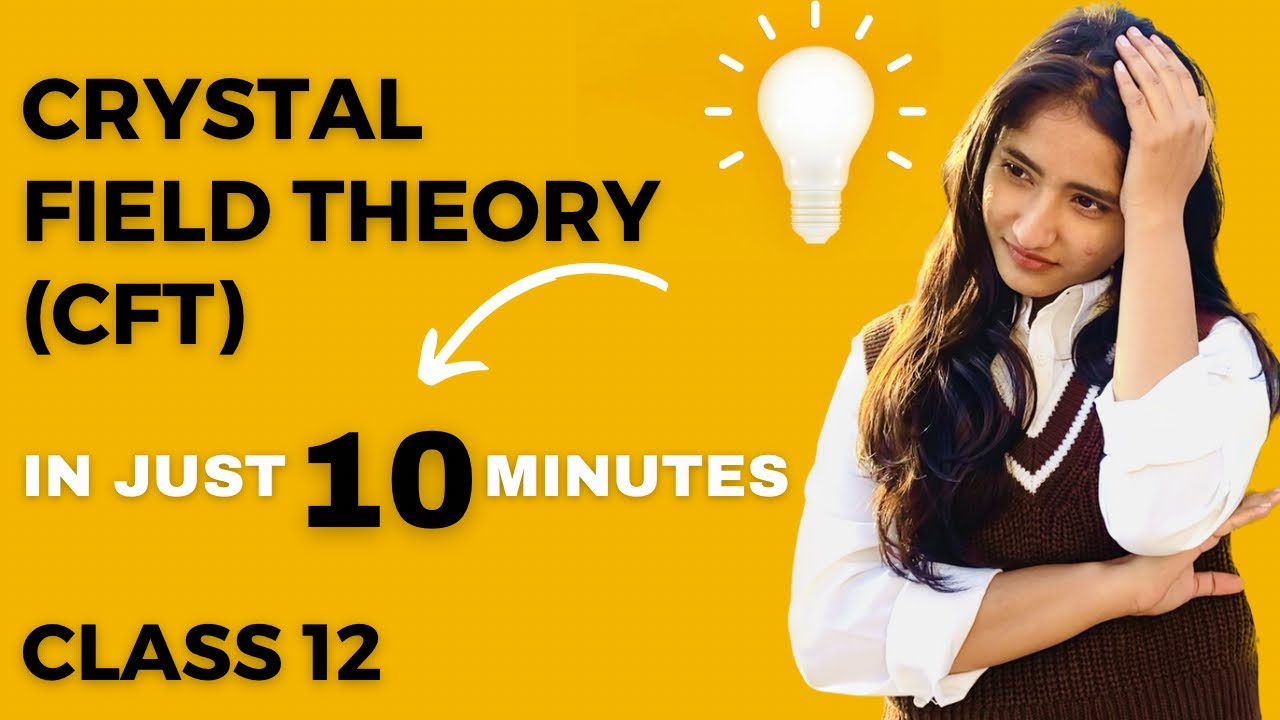
Crystal Field Theory | in just 10 minutes | Class 12 | Coordination Compounds | ISC CBSE board
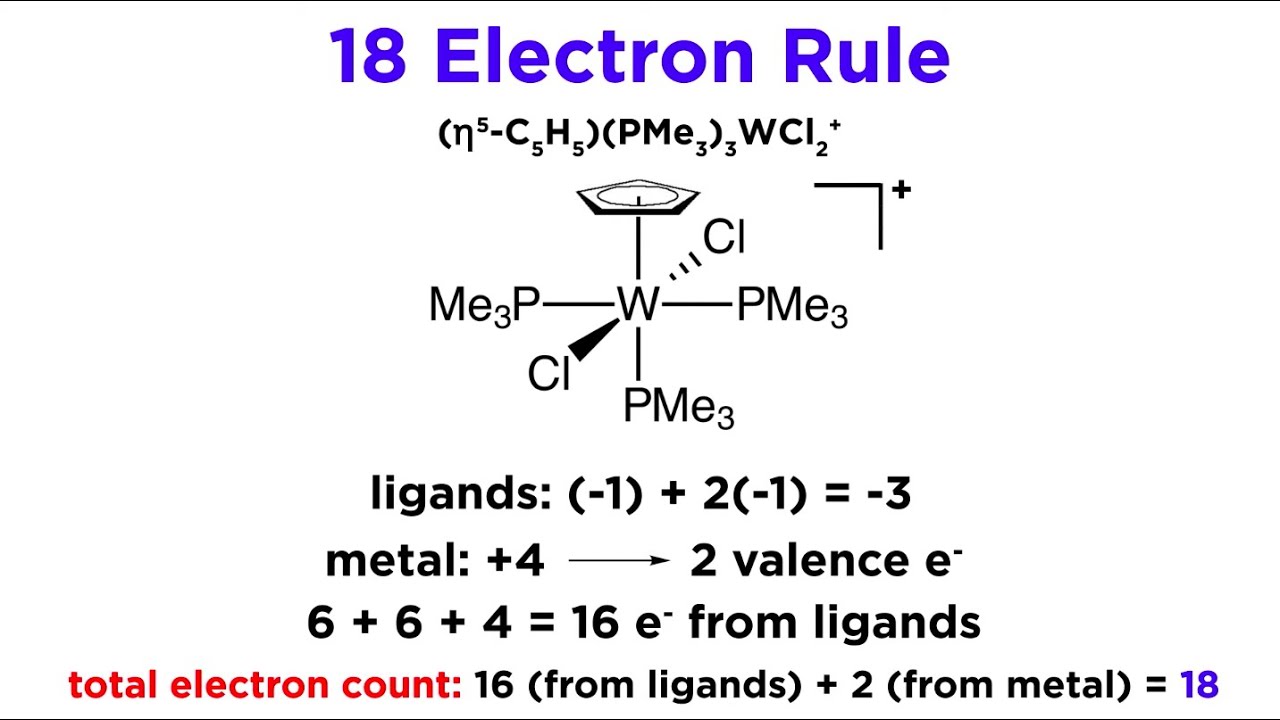
The 18 Electron Rule for Transition Metal Complexes
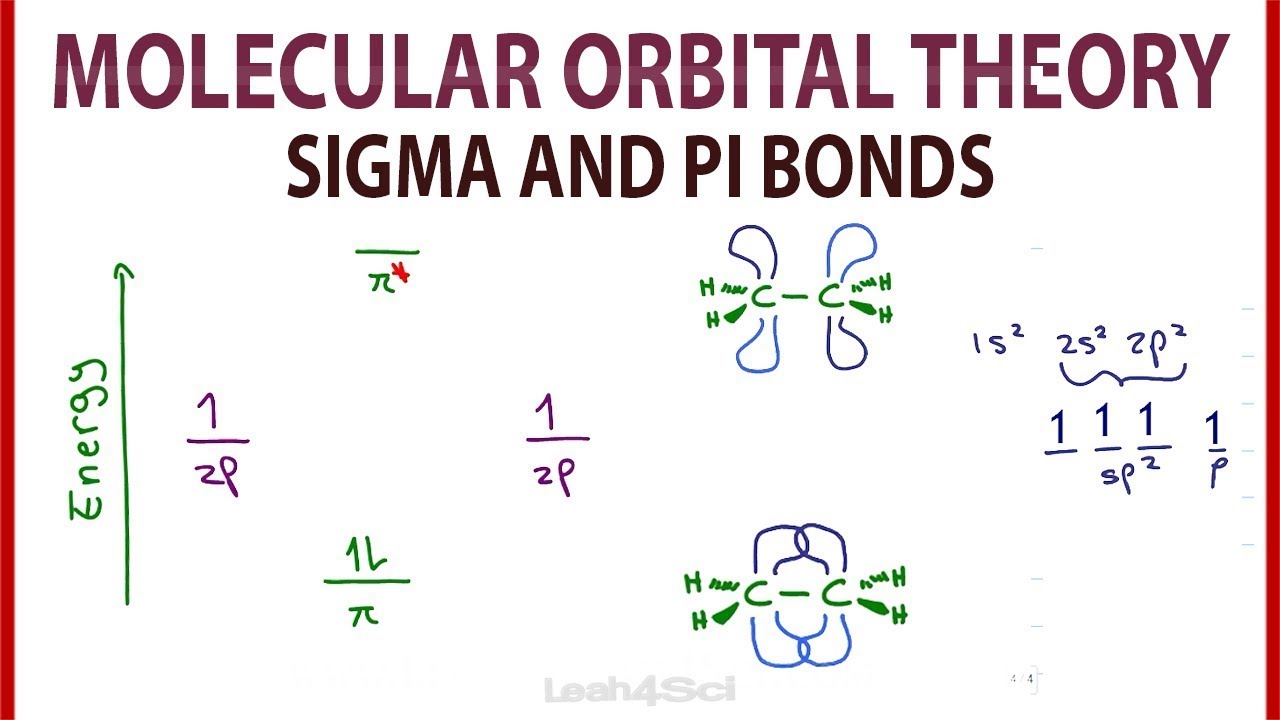
Molecular Orbital MO Theory Simplified for Sigma and Pi Bonds
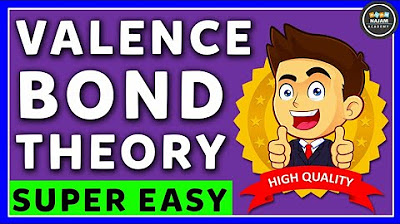
Valence Bond Theory | VBT | Chemistry
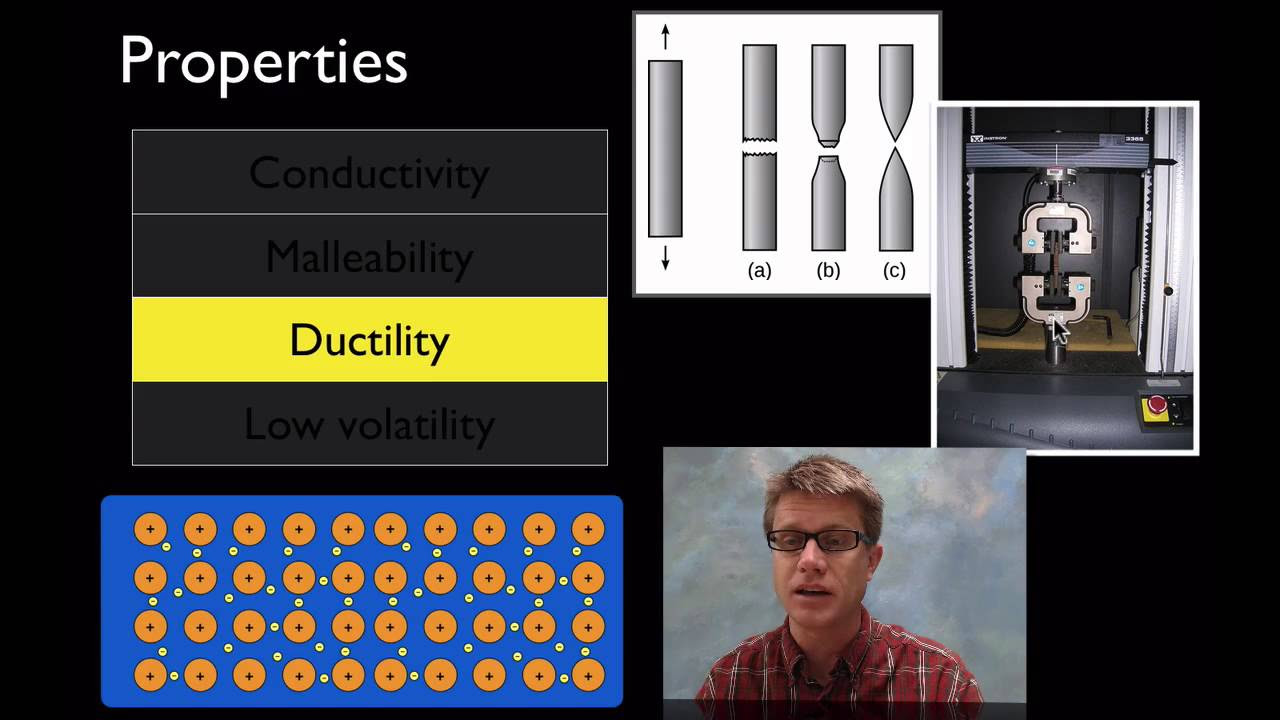
Metallic Bonding
5.0 / 5 (0 votes)