The First Law of Thermodynamics: Internal Energy, Heat, and Work
Summary
TLDRProfessor Dave explains the first law of thermodynamics, detailing its relation to internal energy, work, and heat. He outlines processes like isovolumetric, isothermal, and adiabatic, emphasizing their significance in thermodynamics. The tutorial also covers the importance of understanding the signs of heat and work in calculations, using an example to illustrate energy transfer as heat and work.
Takeaways
- 🔄 The first law of thermodynamics, also known as the law of energy conservation, describes the relationship between internal energy, work, and heat.
- ⚖️ The law is mathematically expressed as ΔU = Q - W, where ΔU is the change in internal energy, Q is the heat transferred, and W is the work done.
- 🔊 In an isovolumetric process, there is no change in volume, meaning no pressure-volume work is done, so ΔU = Q.
- 🌡️ An isothermal process is characterized by no change in temperature, implying no change in internal energy (ΔU = 0), and thus Q = W.
- 🌀 An adiabatic process involves no heat transfer (Q = 0), so any change in internal energy is due to work done on or by the system (ΔU = -W).
- 🏔️ In Earth's atmosphere, adiabatic processes can be observed as air masses move due to pressure differences without heat exchange.
- 🔒 An isolated system, where neither heat nor work is exchanged, will have no change in internal energy (ΔU = Q = W = 0).
- ✅ The signs of Q and W are crucial: Q is positive when heat is absorbed and negative when released; W is positive when work is done by the system and negative when work is done on the system.
- 📐 To solve for heat transfer in a system, rearrange the first law equation to Q = ΔU + W and substitute the appropriate values for ΔU and W.
- 📚 Understanding these principles and calculations is fundamental for further studies and applications in thermodynamics.
Q & A
What is the first law of thermodynamics?
-The first law of thermodynamics, also known as the law of energy conservation, outlines the relationship between internal energy, work, and heat. It can be expressed by the equation ΔU = Q - W, where ΔU is the change in internal energy, Q is the heat transferred, and W is the work done.
What does the equation ΔU = Q - W represent?
-The equation ΔU = Q - W represents that the change in internal energy (ΔU) of a system is equal to the energy transferred to or from the system as heat (Q) minus the energy transferred as work (W), with all quantities measured in joules.
What is an isovolumetric process?
-An isovolumetric process is a process where there is no change in volume for the system, meaning no pressure-volume work can be done on or by the system. In such a case, ΔU equals Q, and any change in internal energy must be the result of heat transfer.
What is an example of an isovolumetric process?
-An example of an isovolumetric process is a bomb calorimeter, where a combustion reaction produces a change in temperature, but the rigid walls result in no change in volume.
What is an isothermal process?
-An isothermal process is a process where there is no change in the temperature of the system, implying no change in internal energy (ΔU = 0), and thus any heat transferred into the system is used to do work (Q = W).
What is an adiabatic process?
-An adiabatic process is a process where there is no heat transfer (Q = 0), and the change in internal energy (ΔU) is solely due to work done on or by the system (W).
How is the sign of Q determined?
-The sign of Q (heat) is positive when heat is absorbed by the system and negative when heat is lost by the system.
How is the sign of W determined?
-The sign of W (work) is positive when work is done by the system (like an expanding gas) and negative when work is done on the system (like gas compression).
What is the significance of the equation ΔU = Q - W in thermodynamics calculations?
-The equation ΔU = Q - W is significant in thermodynamics calculations as it allows us to determine the changes in internal energy, the amount of heat transferred, and the work done in various processes, which is crucial for understanding energy transformations.
What does it mean if both Q and W are zero in a thermodynamic process?
-If both Q (heat transfer) and W (work done) are zero in a thermodynamic process, it indicates an isolated system where there is no exchange of energy with the surroundings, and thus no change in internal energy can occur.
Outlines
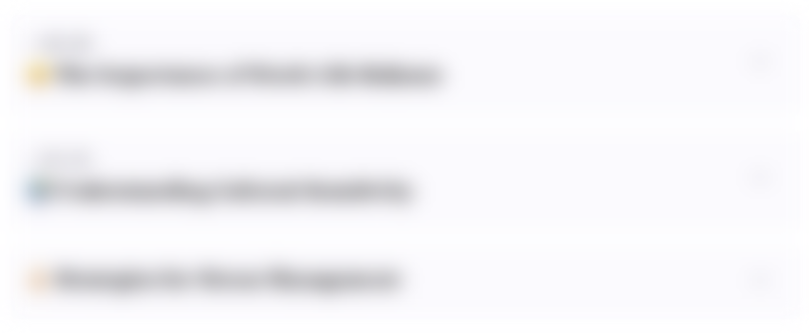
هذا القسم متوفر فقط للمشتركين. يرجى الترقية للوصول إلى هذه الميزة.
قم بالترقية الآنMindmap
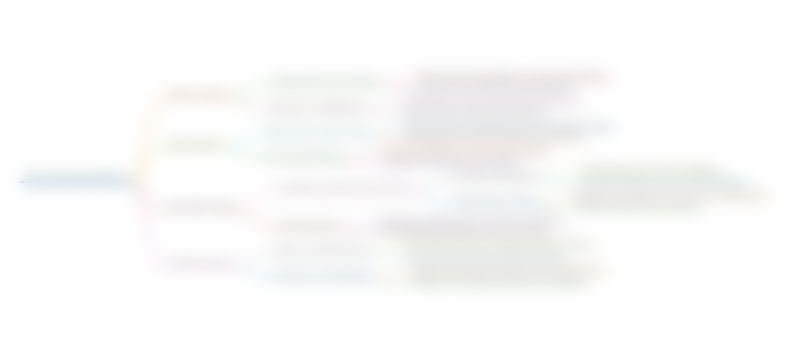
هذا القسم متوفر فقط للمشتركين. يرجى الترقية للوصول إلى هذه الميزة.
قم بالترقية الآنKeywords
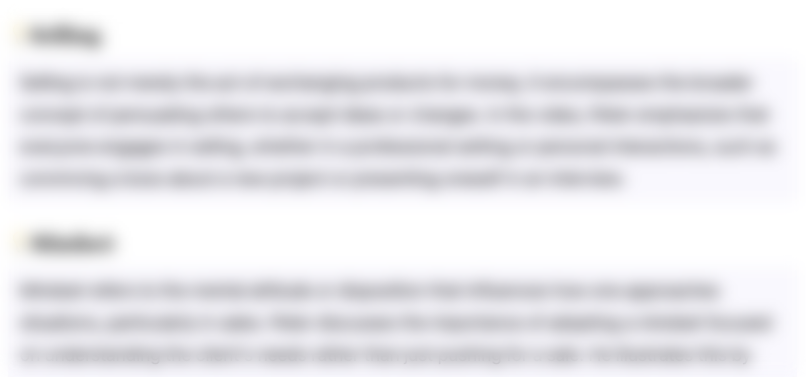
هذا القسم متوفر فقط للمشتركين. يرجى الترقية للوصول إلى هذه الميزة.
قم بالترقية الآنHighlights
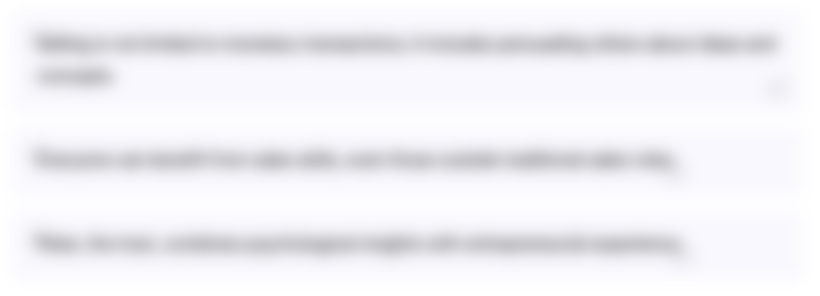
هذا القسم متوفر فقط للمشتركين. يرجى الترقية للوصول إلى هذه الميزة.
قم بالترقية الآنTranscripts
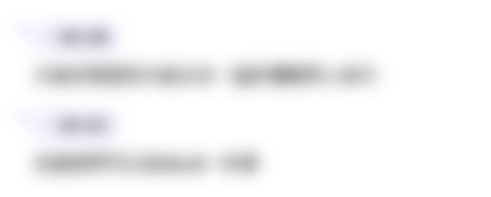
هذا القسم متوفر فقط للمشتركين. يرجى الترقية للوصول إلى هذه الميزة.
قم بالترقية الآنتصفح المزيد من مقاطع الفيديو ذات الصلة
5.0 / 5 (0 votes)