Ultrasound Beam | Ultrasound Physics | Radiology Physics Course #15
Summary
TLDRThis educational video script delves into the intricacies of ultrasound beams, explaining the principles that govern their shape and behavior. It distinguishes between single element and multi-element transducers, highlighting how they affect beam properties like focal point and divergence angle. The script explores Huygen's principle to illustrate wavelet interference and beam convergence, and discusses how adjusting transducer diameter and ultrasound frequency impacts the near field distance and divergence. It also touches on side lobes and grating lobes, offering insights into optimizing ultrasound imaging for better resolution and penetration.
Takeaways
- 🌟 Ultrasound probes are categorized into single element transducers and multi-element transducer arrays, each affecting the ultrasound beam differently.
- 📡 The ultrasound beam shape is influenced by properties such as the transducer type, diameter, and frequency, which can be manipulated to optimize image quality.
- 🎯 The ultrasound beam naturally converges to a focal point within the tissue, known as the near field or Fresnel zone, before diverging in the far field or Fraunhofer zone.
- 🔍 The focal distance, or the distance from the transducer to the focal point, can be adjusted on ultrasound machines to affect image resolution and depth.
- 📏 The near field distance is calculated based on the transducer's diameter and the wavelength of the ultrasound wave, with larger diameters and higher frequencies resulting in a deeper focal point.
- 📉 The divergence angle of the ultrasound beam is determined by the transducer's diameter and the frequency of the ultrasound wave, with larger diameters and higher frequencies reducing divergence.
- 🛠 Huygen's principle explains how the ultrasound beam converges and diverges, as each point on the wavefront can be considered a source of spherical wavelets that interfere constructively or destructively.
- 📋 The width of the ultrasound beam at the transducer face is crucial for image resolution, with the beam width doubling at the focal point in the near field.
- 🌐 Side lobes and grating lobes are secondary ultrasound phenomena that can affect image quality, and can be mitigated by adjusting transducer design and wave properties.
- 🔧 In multi-element transducer arrays, the effective diameter used for calculating beam properties is the combined diameter of the active elements, allowing for deeper focal points and less divergence.
Q & A
What are the two broad categories of transducers mentioned in the script?
-The two broad categories of transducers mentioned are single element transducers and multi-element transducer arrays.
What is the term used to describe the region where the ultrasound beam narrows down to its most intense point?
-The region where the ultrasound beam narrows down to its most intense point is known as the focal point.
What is the near field or Fresnel zone in the context of an ultrasound beam?
-The near field or Fresnel zone is the distance from the transducer to the narrowest part of the beam, where it converges to the focal point.
How is the far field or Fraunhofer zone different from the near field in an ultrasound beam?
-The far field or Fraunhofer zone is the region after the near field where the ultrasound beam starts to diverge and continues until it is fully attenuated, unlike the near field which is the converging region up to the focal point.
What is Huygen's principle as it relates to the ultrasound beam?
-Huygen's principle states that a wave can be separated into an infinite amount of small discrete wavelets, which can act independently as small sources of wave energy, affecting how the ultrasound beam converges and diverges.
How does the diameter of the transducer affect the near field distance?
-As the diameter of the transducer element increases, the near field distance also increases, meaning the focal point is deeper within the tissue.
What is the relationship between the frequency of the ultrasound wave and the near field distance?
-Higher frequency ultrasound waves result in a deeper focal point, thus increasing the near field distance.
What is the formula used to calculate the near field distance in soft tissue?
-The near field distance in soft tissue is calculated as the diameter in millimeters squared times the frequency in megahertz over four times the speed of sound in soft tissue.
How does the divergence angle of an ultrasound beam relate to the diameter and frequency of the transducer?
-The divergence angle decreases as the diameter of the transducer element or the frequency of the ultrasound probe increases, resulting in less beam divergence.
What are side lobes in the context of an ultrasound beam and how can they be reduced?
-Side lobes are secondary ultrasound waves that propagate in the forward direction and can interfere with the primary beam. They can be reduced by dampening the ultrasound wave, reducing the quality factor, narrowing transducer elements to less than half the wavelength, or reducing the amplitude of peripheral waves.
What is a grating lobe and how does it differ from a side lobe?
-A grating lobe is a type of wave produced by a multi-element transducer array due to the interference of waves along the array. It differs from a side lobe as it generally occurs more in transducer arrays and is less likely to interfere with the primary image.
Outlines
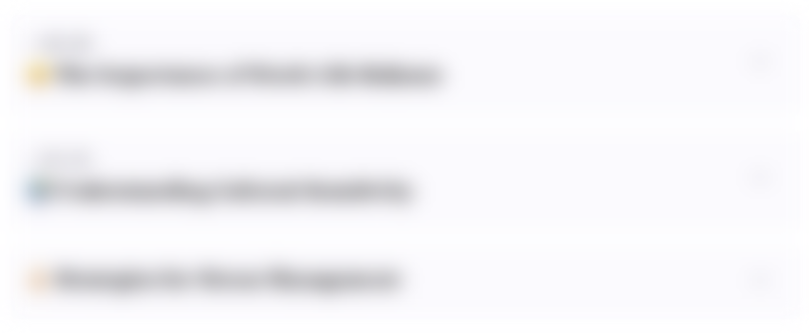
هذا القسم متوفر فقط للمشتركين. يرجى الترقية للوصول إلى هذه الميزة.
قم بالترقية الآنMindmap
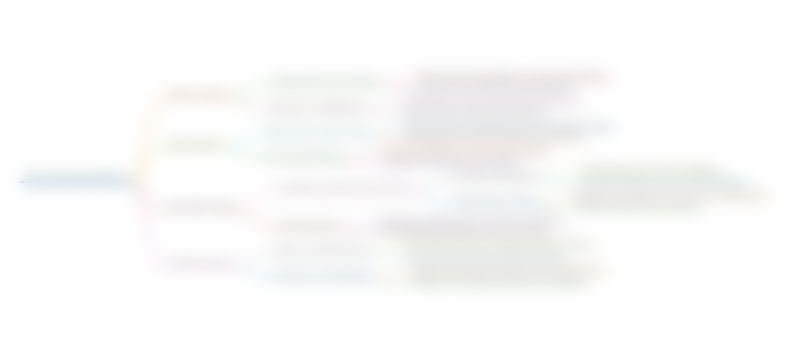
هذا القسم متوفر فقط للمشتركين. يرجى الترقية للوصول إلى هذه الميزة.
قم بالترقية الآنKeywords
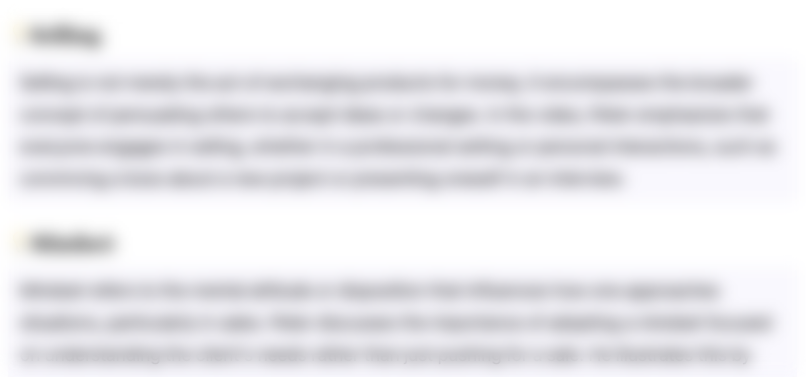
هذا القسم متوفر فقط للمشتركين. يرجى الترقية للوصول إلى هذه الميزة.
قم بالترقية الآنHighlights
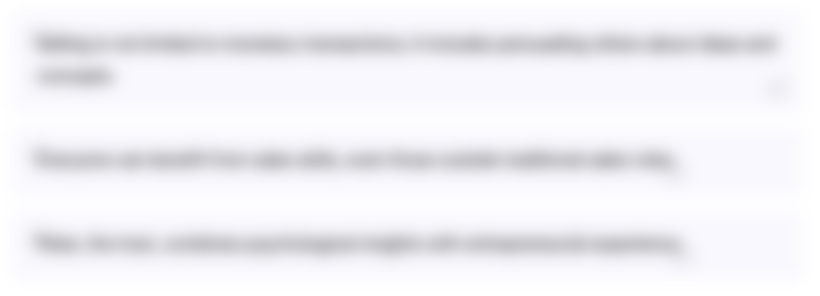
هذا القسم متوفر فقط للمشتركين. يرجى الترقية للوصول إلى هذه الميزة.
قم بالترقية الآنTranscripts
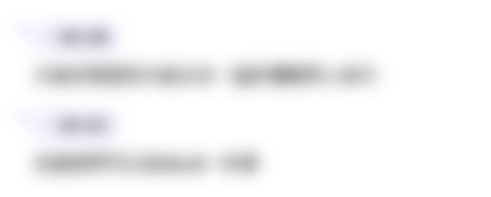
هذا القسم متوفر فقط للمشتركين. يرجى الترقية للوصول إلى هذه الميزة.
قم بالترقية الآنتصفح المزيد من مقاطع الفيديو ذات الصلة

Ultrasound Physics 3 - Beam Formation and Transducers
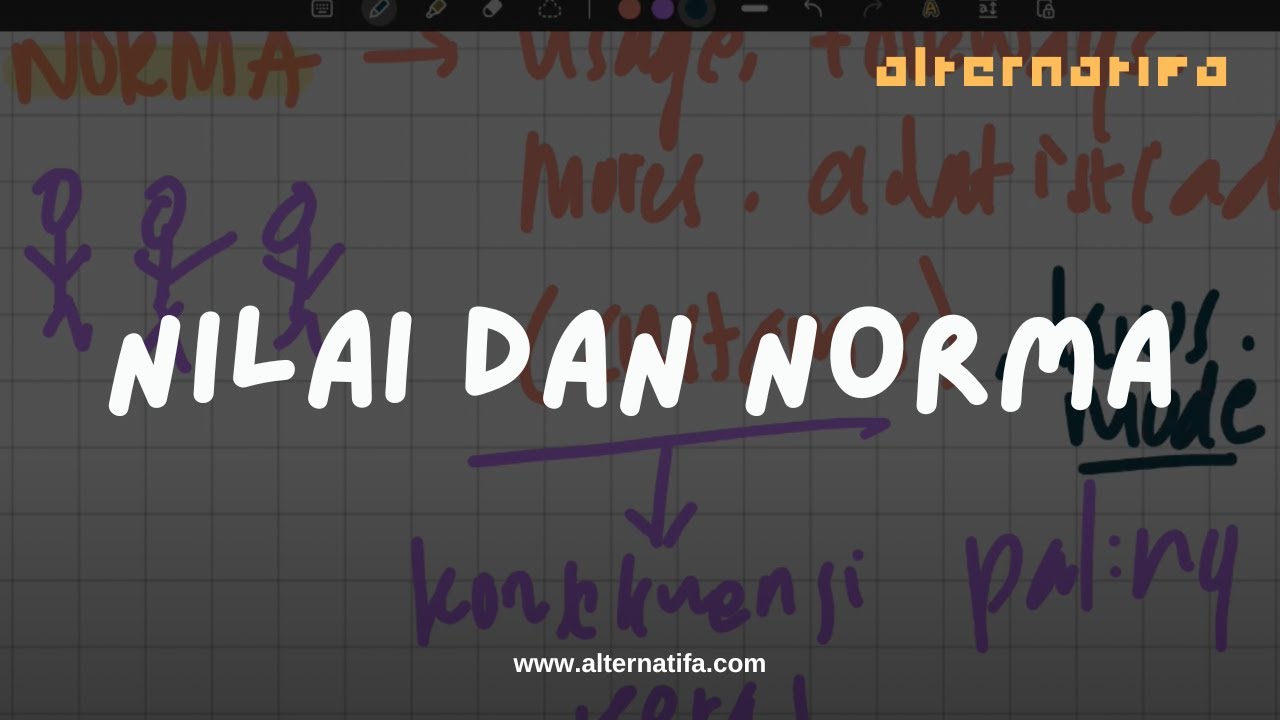
Chapter 2: Nilai dan Norma | Sosiologi | Alternatifa
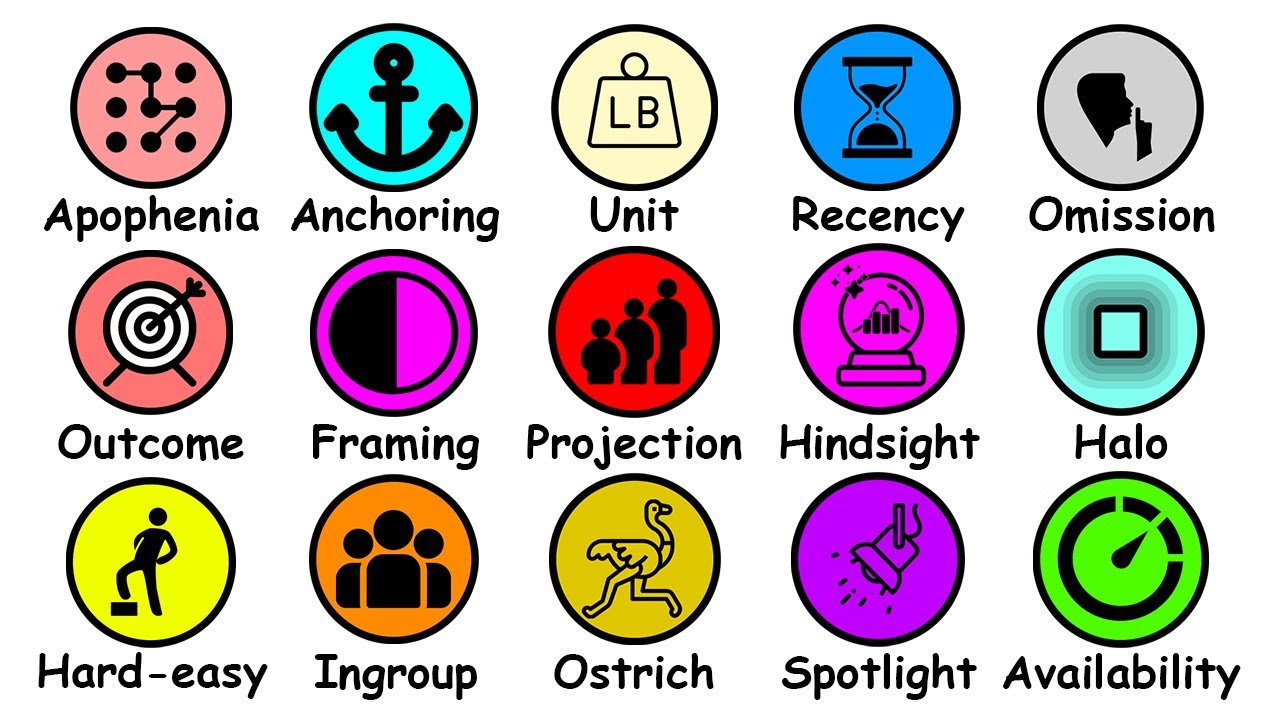
Every Bias Explained in 8 Minutes
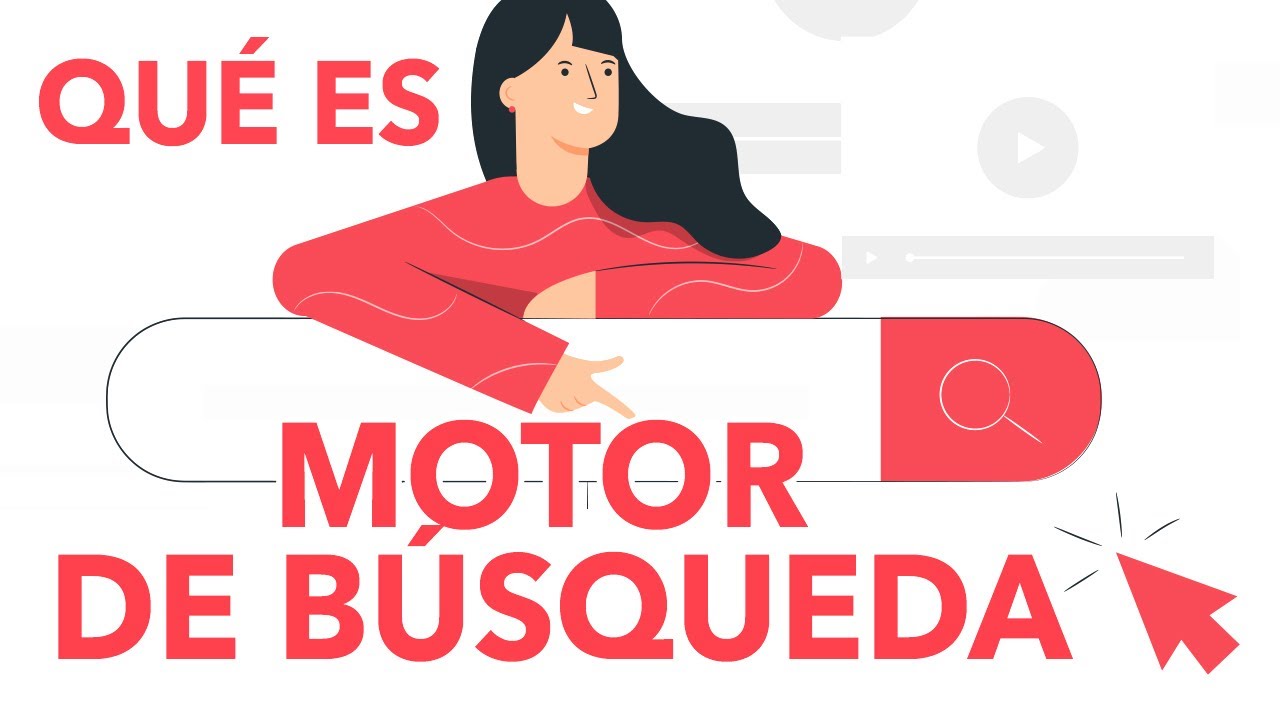
¿Qué es y cómo funciona un Motor de Búsqueda en Internet?
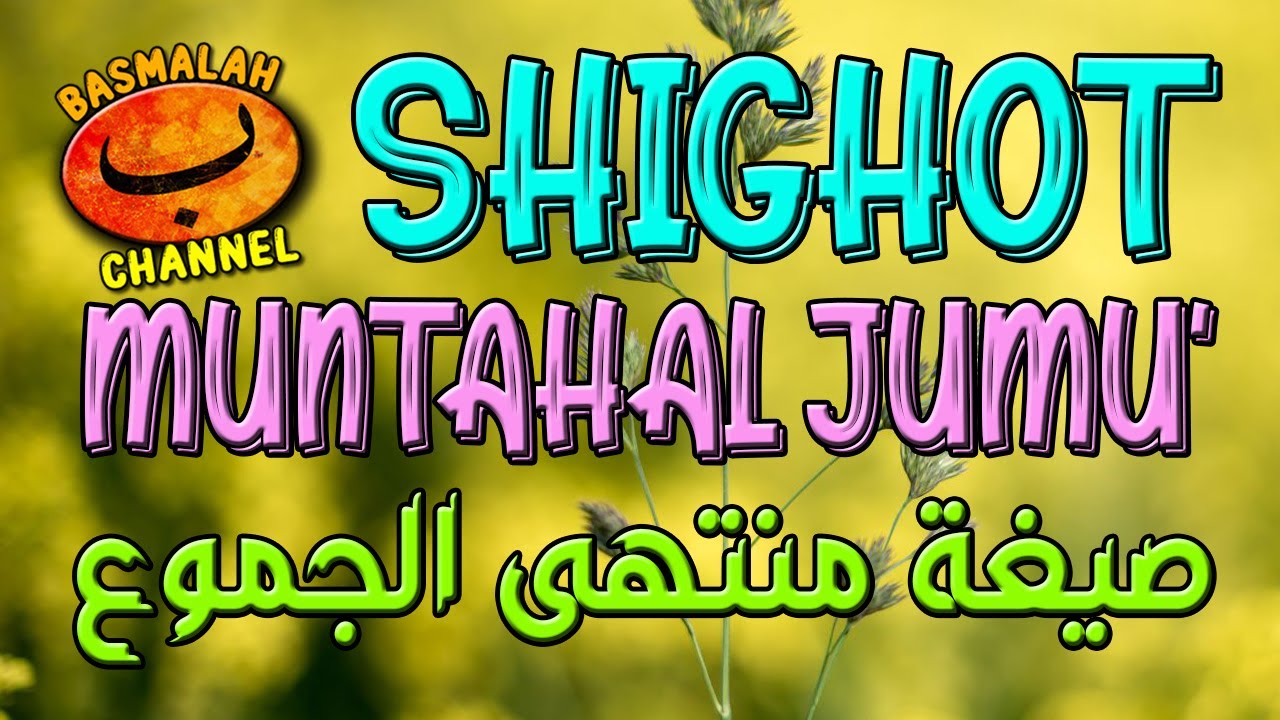
LEARN THAT - SHORROF : Wazan-wazan shighot vomiting jumu' (rules of nahwu)

Will the Universe Expand Forever?
5.0 / 5 (0 votes)