The Incredible Science of Bioprinting
Summary
TLDRThis script discusses the groundbreaking advancements in bioprinting, a technology that creates complex 3D biological structures like functioning lung scaffolds. It has the potential to transform healthcare by addressing organ shortages and revolutionizing drug development. The script explores the history of bioprinting, from its early days to current sophisticated techniques using bioinks. It also highlights the future possibilities, including integration with nanotechnology and AI, suggesting a future of personalized medicine and sustainable solutions.
Takeaways
- 🔬 The most complex 3D object ever created using additive manufacturing is a functioning scaffold of lungs with 200 million alveoli and 4000 kilometers of capillaries.
- 🐭 This technology has been successfully tested in animals and could revolutionize organ transplantation and drug testing.
- 🌐 Bioprinting has the potential to transform medicine by building living tissues and organs layer by layer.
- 🏥 It could help overcome the shortage of donor organs, saving lives and improving the quality of life for millions.
- 💊 Bioprinting could revolutionize drug development and testing, making processes safer and more efficient.
- 📚 The concept of creating living tissue has been around for decades, but significant strides have been made recently to turn theory into reality.
- 👨🔬 In the 1990s, scientists began experimenting with inkjet printers to build living cells, marking the beginning of bioprinting.
- 📈 By the 2000s, tissue engineering gained traction with successful implants of engineered bladders.
- 🖨 The first patent for a bioprinter was filed in 2003, marking a significant turning point for the technology.
- 🌟 Bioprinting uses a special material known as bio ink, which is a mixture of cells, nutrients, and support matrices.
- 📊 Bioprinting follows a blueprint based on CT or MRI scans, creating thousands of two-dimensional slices for the printer to build upon.
- 🧬 The future of bioprinting may involve integration with nanotechnology, robotics, and AI, leading to innovations like bio-hybrid robots and personalized medicine.
Q & A
What is the significance of the 3D printed lung scaffold mentioned in the script?
-The 3D printed lung scaffold is significant because it represents the state of the art in 3D bioprinting, with the ability to create a functioning structure for lungs, including 200 million alveoli and 4000 kilometers of lung capillaries. It has been successfully tested in animals and could revolutionize organ transplantation and healthcare.
How does bioprinting have the potential to transform medicine?
-Bioprinting has the potential to transform medicine by building living tissues and organs layer by layer, which could help overcome the shortage of donor organs, save lives, and improve the quality of life for millions. It could also revolutionize drug development and testing by enabling safer and more efficient processes.
What was the foundational technology that paved the way for bioprinting?
-Stereolithography, invented by Dr. Charles Hull in 1983, was the foundational technology that paved the way for bioprinting. It was originally intended for creating plastic prototypes but later inspired the use of similar principles to build biological structures.
Who is often credited as the father of bioprinting?
-Thomas Boland is often credited as the father of bioprinting, having filed the first patent for a bioprinter in 2003.
What is the role of bio ink in bioprinting?
-Bio ink plays a crucial role in bioprinting as it is a special material composed of cells, nutrients, and support matrices. It is used to create the living, breathing structures that form the basis of the printed tissues and organs.
How does the process of bioprinting begin?
-The process of bioprinting begins with a digital model, typically based on a CT or MRI scan, which is imported into slicing software to create thousands of two-dimensional slices. The printer then places material layer by layer to print the desired structure.
What are the different types of bio inks mentioned in the script?
-The script mentions three types of bio inks: alginate, which is a natural biomaterial often used for cartilage, tissue engineering, wound healing, and drug delivery; gelatin, which is artificially synthesized and often used for bone tissue, liver tissue, and skin regeneration; and DCM or decellular extracellular matrix, which uses existing material in human or animal tissue but with cells removed, leaving behind the natural scaffolding material.
What is the significance of the structural bio ink in bioprinting?
-The structural bio ink is significant as it forms the entire scaffold of the tissue or organ and provides the framework within which all the other inks operate. It is crucial for guiding cellular growth and development.
How does stereolithography (SLA) bioprinting work?
-Stereolithography bioprinting uses a light source, typically a laser or digital light projector, to transform light-sensitive bio ink into solid 3D structures layer by layer. The process starts with a pool of bio ink, and as each layer is exposed to light, it hardens, allowing the next layer to be formed until the entire structure is built.
What are some potential future developments in bioprinting mentioned in the script?
-The script mentions that future developments in bioprinting may involve integration with nanotechnology, robotics, and artificial intelligence, leading to innovations like bio-hybrid robots, smart implants, and more personalized medicine. It also suggests the potential for bioprinting tissues to model and study complex diseases.
How could bioprinting technology become more accessible in the future?
-As bioprinting technology advances, becomes more affordable, and accessible, it could be adopted across various industries, including medicine, research, food production, and fashion, transforming lives in countless ways.
Outlines
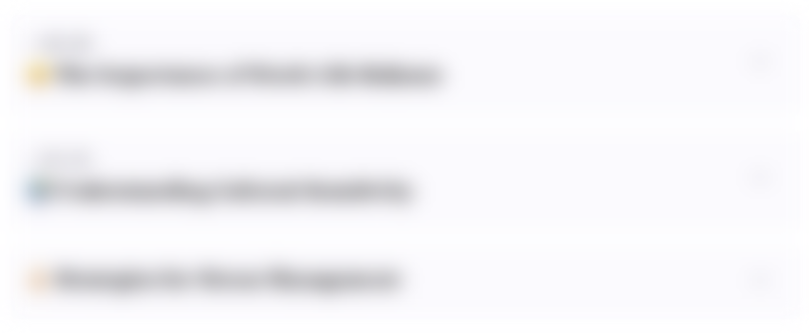
此内容仅限付费用户访问。 请升级后访问。
立即升级Mindmap
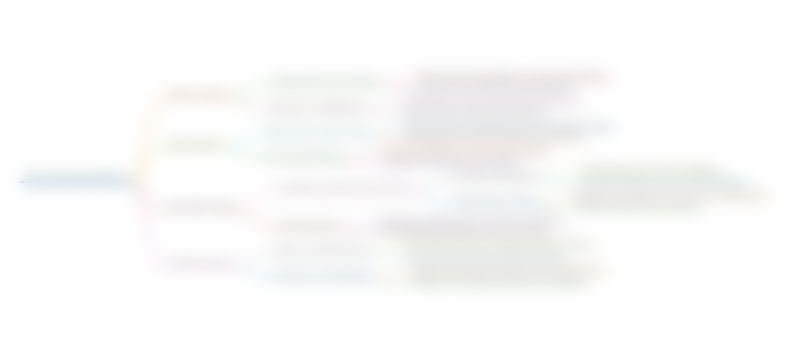
此内容仅限付费用户访问。 请升级后访问。
立即升级Keywords
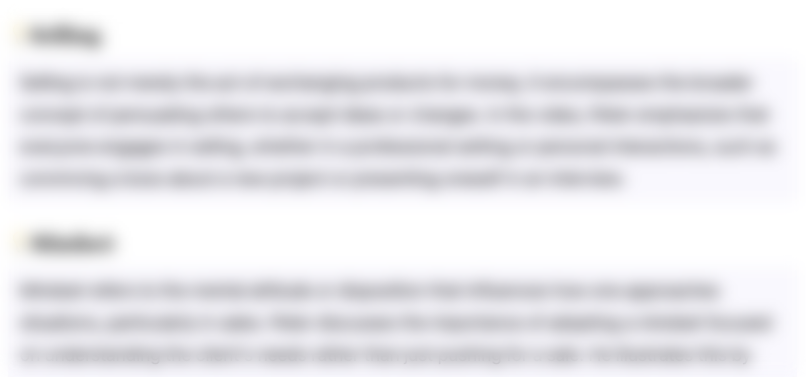
此内容仅限付费用户访问。 请升级后访问。
立即升级Highlights
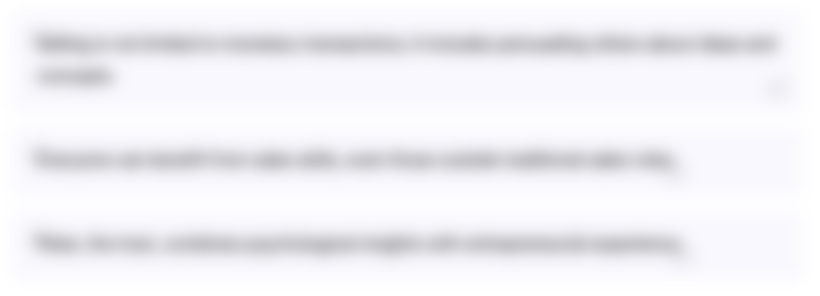
此内容仅限付费用户访问。 请升级后访问。
立即升级Transcripts
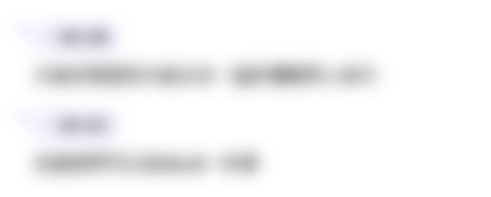
此内容仅限付费用户访问。 请升级后访问。
立即升级5.0 / 5 (0 votes)