The Laws of Thermodynamics, Entropy, and Gibbs Free Energy
Summary
TLDRProfessor Dave's tutorial on thermodynamics explains the fundamental laws that govern energy flow and direction. The first law emphasizes energy conservation, while the second introduces entropy, the measure of disorder in a system. The third law discusses absolute zero entropy. The video clarifies that entropy doesn't preclude order, as enthalpy and entropy together determine the spontaneity of processes through Gibbs free energy. Practical examples, like soap forming micelles, illustrate how order can emerge spontaneously under certain conditions.
Takeaways
- 🔄 The First Law of Thermodynamics emphasizes the conservation of energy, stating that energy can change forms but is not created or destroyed.
- 🔑 The Second Law introduces entropy, which can be understood as a measure of disorder within a system, and it always increases for the universe as a whole.
- 🛌 An analogy for entropy is a bedroom that naturally becomes messier over time, illustrating the tendency towards higher disorder.
- 📈 Entropy can also be viewed as a measure of how dispersed the energy of a system is, with higher entropy indicating more randomness.
- 💻 Entropy is related to the amount of information needed to describe a system, with more ordered states requiring more detailed descriptions.
- ☕️ Heat spontaneously flows from hot to cold due to the increase in entropy when energy is dispersed.
- ❄️ The Third Law of Thermodynamics states that at absolute zero, a perfect crystal has zero entropy, representing the most ordered state.
- 📏 Entropy is measured in joules per kelvin and is distinct from energy, which is described by enthalpy.
- 📉 Gibbs free energy (G) determines the spontaneity of a process, calculated by the equation ΔG = ΔH - TΔS, where a negative ΔG indicates a spontaneous process.
- 🔄 Both enthalpically and entropically favorable processes can be spontaneous, as can processes that are only one or the other under certain conditions.
- 🧼 An example of a process that is entropically unfavorable but energetically favorable is the formation of soap micelles, which can spontaneously trap dirt and be washed away.
Q & A
What are the laws of thermodynamics and why are they important?
-The laws of thermodynamics are fundamental principles that govern the flow and transformation of energy. They are important because they help us understand why energy flows in certain directions and ways, and they are essential for making predictions about systems at various scales, from the microscopic to the macroscopic.
What does the first law of thermodynamics state and what does it imply about energy?
-The first law of thermodynamics, also known as the law of conservation of energy, states that energy cannot be created or destroyed, only converted from one form to another. This implies that the total energy of a closed system remains constant, although it may change forms, such as from potential to kinetic energy.
How is entropy defined in the context of the second law of thermodynamics?
-Entropy, in the context of the second law of thermodynamics, is defined as a measure of disorder or randomness in a system. It quantifies the degree to which energy is dispersed within the system, with higher entropy indicating a more disordered state.
What does it mean for the entropy of the universe to always be increasing?
-The statement that the entropy of the universe always increases means that over time, the overall disorder or randomness in the universe tends to increase. This is often interpreted as a tendency towards a more dispersed energy state within the system and its surroundings.
Can you provide an example of how entropy is related to everyday life, as mentioned in the script?
-An example from the script is the analogy of a bedroom becoming messy over time. It's more likely for a room to become disordered than to spontaneously organize itself, illustrating the natural tendency towards increased entropy.
How does the concept of entropy relate to the way we describe the states of matter, such as solids and liquids?
-In the context of states of matter, a solid is more ordered and has lower entropy compared to a liquid, which is more disordered and has higher entropy. The script uses the analogy of computer code to illustrate that less information is needed to describe a liquid state due to the randomness of molecular motion.
What is the third law of thermodynamics and what does it tell us about entropy at absolute zero?
-The third law of thermodynamics states that the entropy of a perfect crystalline solid at absolute zero is zero. This indicates that at absolute zero, the substance is in its most ordered state with the lowest possible entropy.
How is entropy different from enthalpy, and what does each measure?
-Entropy measures the degree of disorder or randomness in a system, indicating how energy is distributed. Enthalpy, on the other hand, is a thermodynamic quantity that describes the total energy of a system, including both internal energy and the energy related to pressure and volume.
What is Gibbs free energy and why is it significant in understanding spontaneous processes?
-Gibbs free energy (G) is a thermodynamic potential that indicates whether a process will occur spontaneously. A negative change in Gibbs free energy (ΔG) means the process is spontaneous, while a positive change indicates a non-spontaneous process. It is significant because it combines the effects of enthalpy and entropy to predict the spontaneity of processes.
Can a process be spontaneous if it is both enthalpically and entropically unfavorable?
-No, a process that is both enthalpically and entropically unfavorable will have a positive change in Gibbs free energy (ΔG), indicating that it is non-spontaneous. The process will not occur spontaneously under normal conditions.
How does the script explain the formation of micelles with soap as an example of spontaneous order?
-The script explains that soap molecules, which have polar heads and nonpolar tails, spontaneously form micelles to trap dirt. The polar heads face outward to interact with water, making the micelle water-soluble, while the nonpolar tails face inward, trapping dirt. This is an example of how enthalpically favorable processes can lead to spontaneous order, despite an increase in entropy.
Outlines
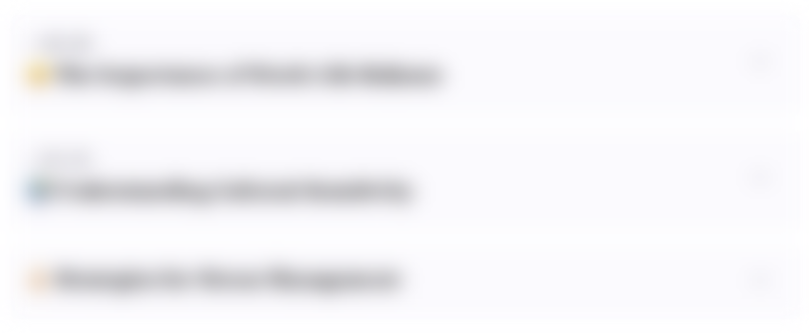
This section is available to paid users only. Please upgrade to access this part.
Upgrade NowMindmap
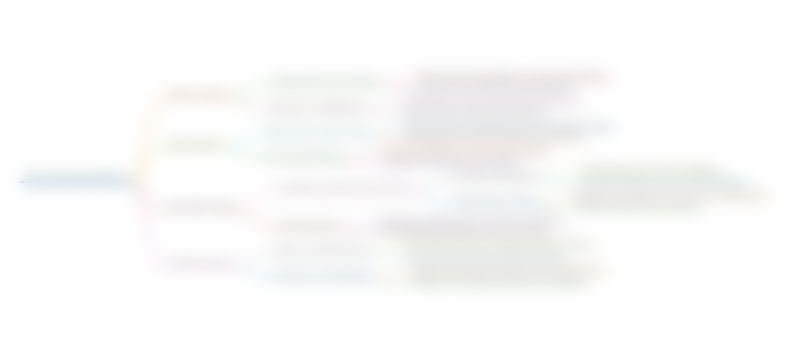
This section is available to paid users only. Please upgrade to access this part.
Upgrade NowKeywords
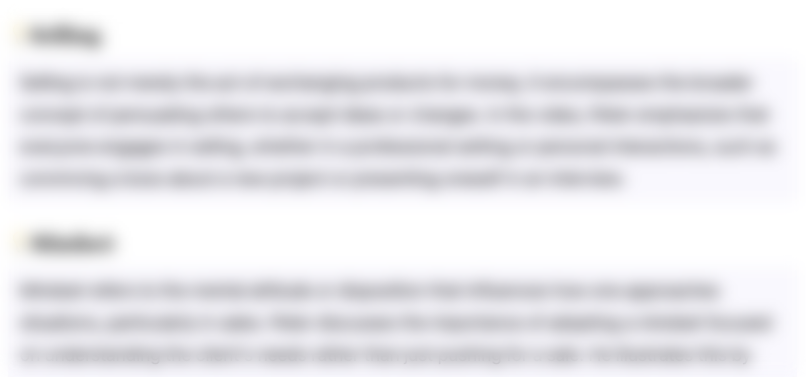
This section is available to paid users only. Please upgrade to access this part.
Upgrade NowHighlights
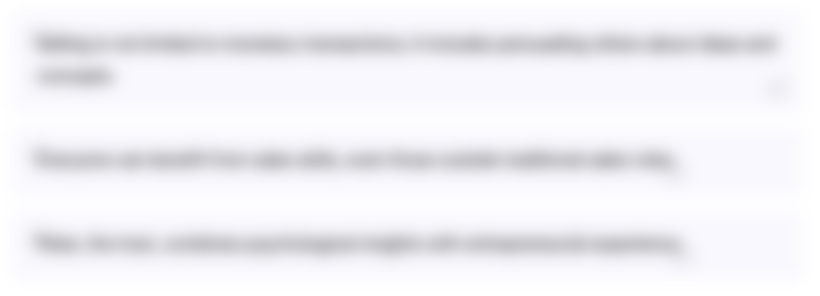
This section is available to paid users only. Please upgrade to access this part.
Upgrade NowTranscripts
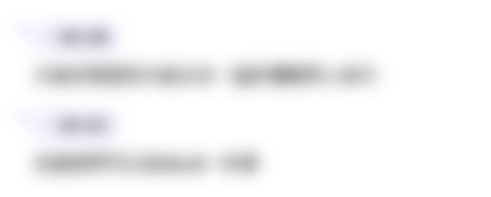
This section is available to paid users only. Please upgrade to access this part.
Upgrade NowBrowse More Related Video

THE LAWS OF THERMODYNAMICS (Zeroth law, 1st, 2nd and 3rds law) | ENGINEERING THERMODYNAMICS |
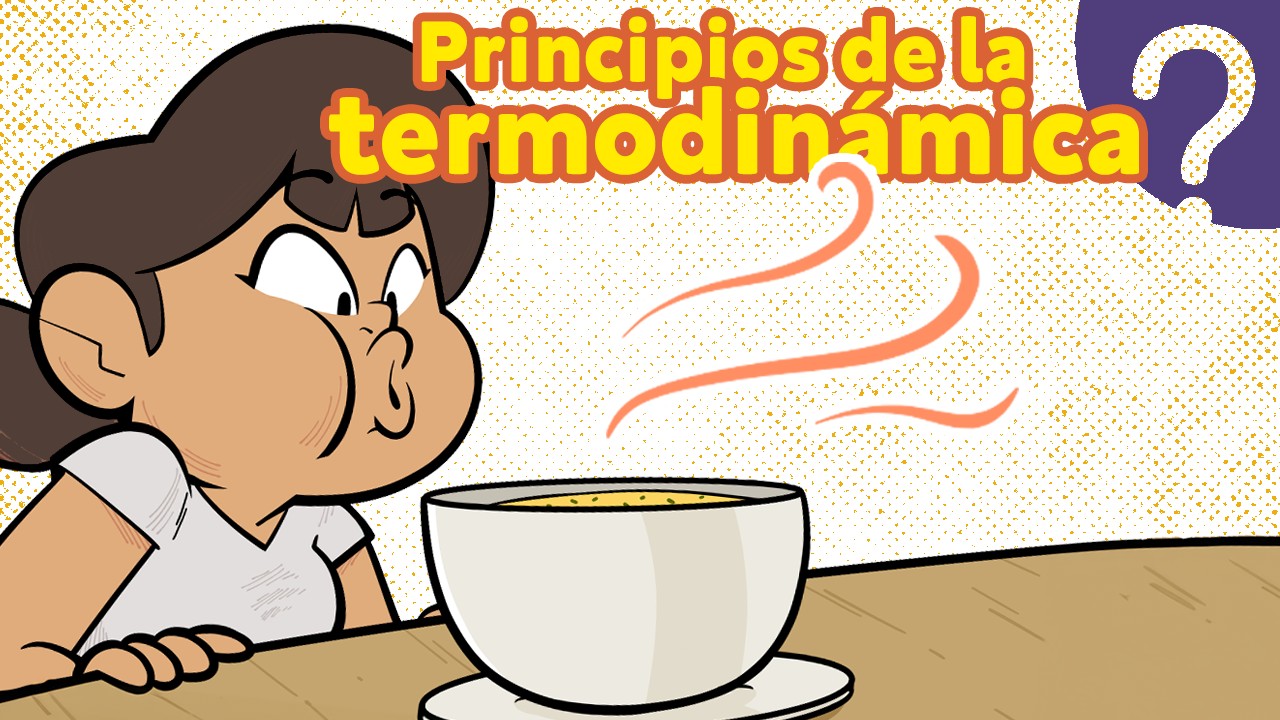
🔥 Las Leyes de la Termodinámica: una explicación sencilla
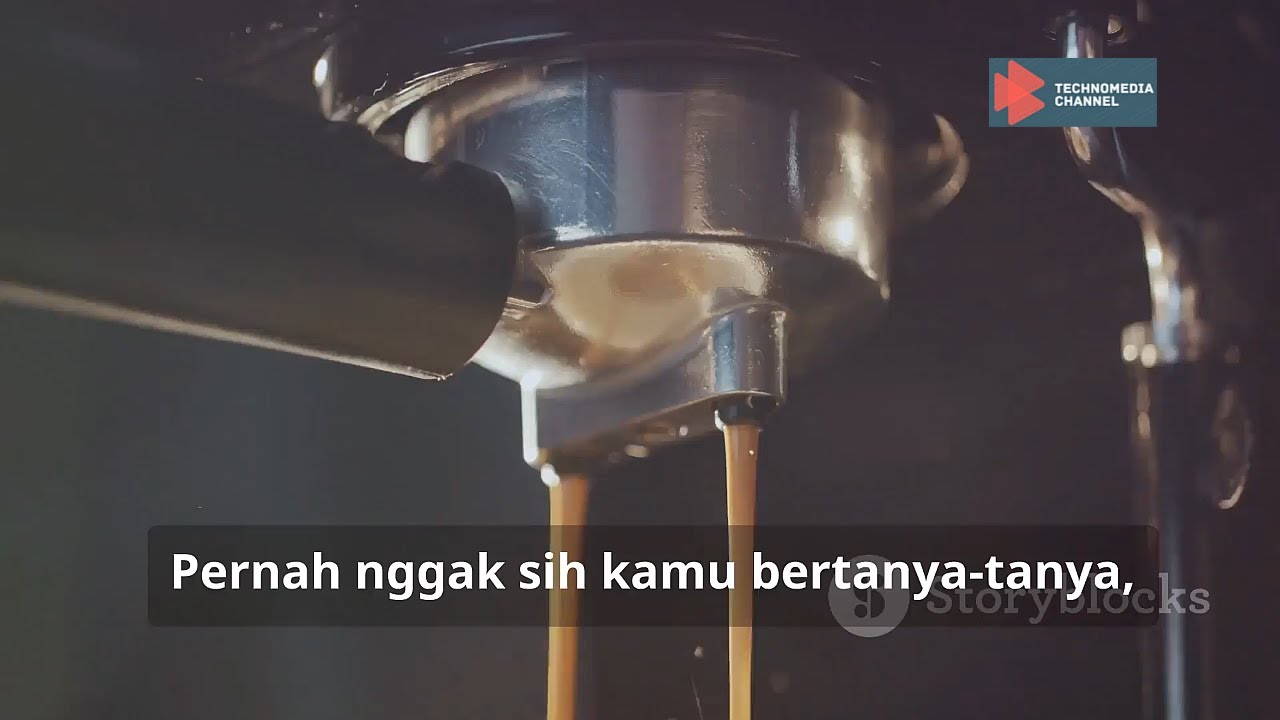
Termodinamika: Cabang Fisika yang Luar Biasa
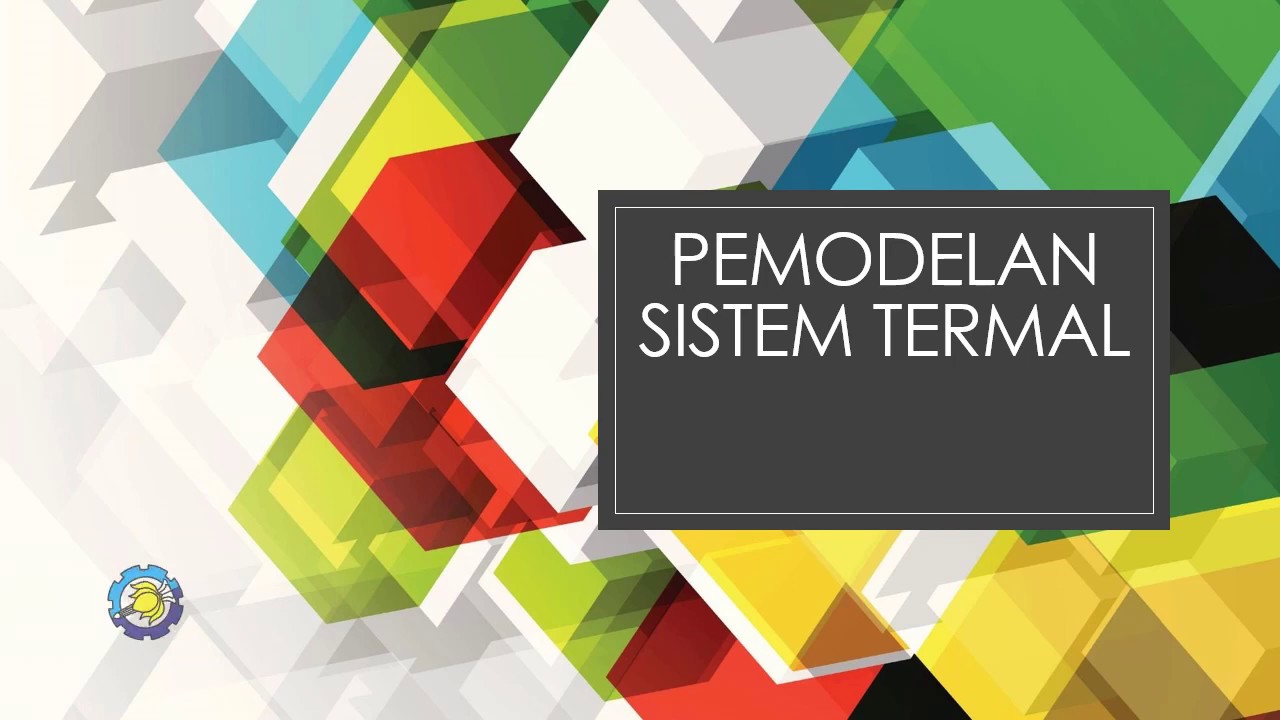
Pemodelan Sistem Termal (lumped parameter)
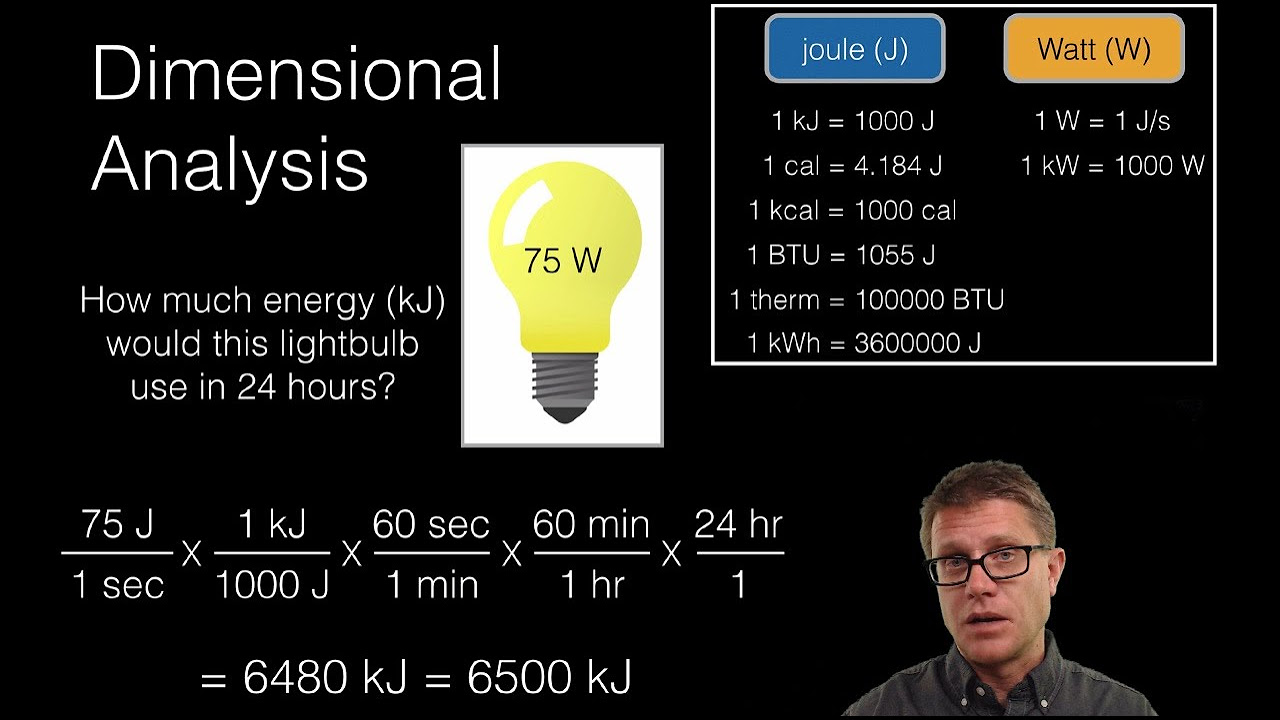
Energy Concepts
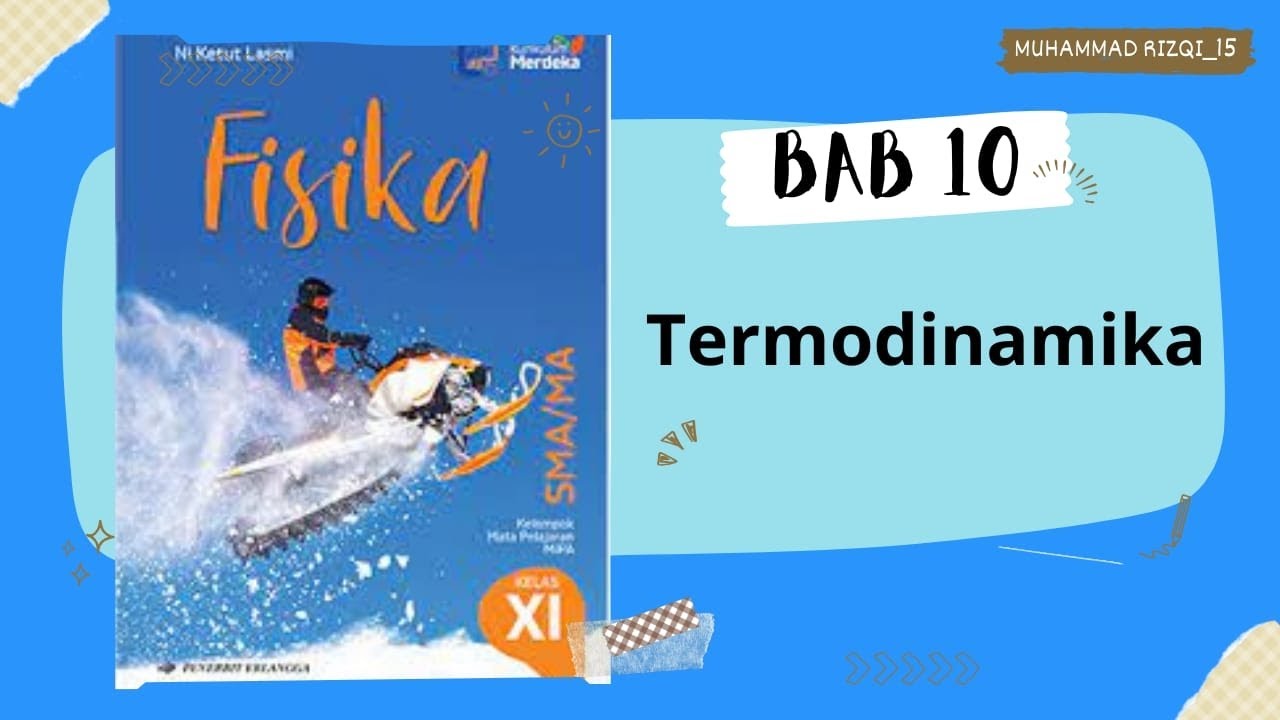
BAB 10 TERMODINAMIKA_MAPEL FISIKA_SMA KELAS XI #fyp #fypage #fypyoutube #termodinamika #fisika
5.0 / 5 (0 votes)