AP Biology Practice 2 - Using Mathematics Appropriately
Summary
TLDRMr. Andersen's video emphasizes the critical role of mathematics in AP Biology, underpinning all scientific disciplines. He discusses the exponential growth in genomics and the impact of chaos theory, computing power, and in silico experiments on mathematical biology. Key equations from the AP curriculum are highlighted, including Hardy-Weinberg Equilibrium, solute potential, and Chi-Squared analysis, alongside practical examples of applying mathematical routines to understand biological phenomena. The video encourages students to develop a mathematical 'sense' for deeper insights into biology.
Takeaways
- π Mathematics is integral to AP Biology as it helps in understanding and applying biological concepts.
- π± The foundation of all sciences, including biology, is mathematics, which is evident in the progression from biology to biochemistry, chemistry, physics, and finally mathematics.
- π The field of mathematical biology is growing rapidly, driven by advancements in genomics, chaos theory, computing power, and in silico laboratory experiments.
- 𧬠Genomic sequencing is becoming cheaper and more prevalent, leading to an exponential growth in the amount of genetic data available for analysis.
- πΏ Chaos theory and mathematical patterns, such as the Rule of Thirty, can predict complex biological phenomena like the growth patterns of ferns and cone snails.
- π» Moore's Law illustrates the exponential increase in computing power, which enables more complex mathematical modeling and simulations in biology.
- π§ͺ In silico experiments allow for the simulation of biological processes in a computer, offering advantages like handling large data sets and avoiding ethical concerns associated with live subjects.
- π Four key equations in the new AP Biology curriculum are crucial for understanding different biological concepts: Hardy-Weinberg Equilibrium, solute potential, Chi-Squared analysis, and population growth models.
- π Students should be able to justify the selection of mathematical routines, calculate mean rates of population growth, and estimate quantities describing natural phenomena using mathematical formulas.
- π The ability to apply mathematical formulas and algorithms is essential for success in AP Biology, including understanding allele frequencies, calculating slopes for growth rates, and predicting changes in osmotic conditions.
- π’ Charles Darwin emphasized the importance of mathematics in scientific discovery, suggesting that it provides a new sense for understanding the natural world.
Q & A
Why is mathematics important in AP Biology according to Mr. Andersen?
-Mathematics is crucial in AP Biology because it forms the foundation of all sciences, including biology. It is essential for understanding the core concepts and for the emerging field of mathematical biology, which is driven by genomics, chaos theory, increasing computing power, and in silico laboratory experiments.
What is the significance of the Hardy-Weinberg Equilibrium in the context of the video?
-The Hardy-Weinberg Equilibrium is an important formula in the field of evolution. It allows students to determine the allele frequencies in a population based on certain measurements, which is a key concept in understanding genetic changes over time.
How does the decrease in the cost of sequencing DNA relate to the growth of mathematical biology?
-The decrease in the cost of sequencing DNA has led to an exponential growth in the amount of genetic data available. This data explosion necessitates the use of mathematical models and computational tools to analyze and interpret the genetic information, thus contributing to the growth of mathematical biology.
What is Moore's Law and how does it relate to the advancement of mathematical biology?
-Moore's Law is the observation that the number of transistors on a microprocessor doubles approximately every two years, leading to faster and cheaper computers over time. This increase in computing power enables more complex mathematical modeling and simulations in biological research.
What is the concept of 'in silico' laboratory experiments mentioned in the script?
-In silico laboratory experiments refer to the simulation of life processes on a computer. This method allows researchers to perform complex experiments and analyze large amounts of data without the ethical concerns or limitations associated with in vivo or in vitro experiments.
What are the four important equations Mr. Andersen mentions in the new AP Biology curriculum?
-The four important equations are related to the four big ideas in the AP Biology curriculum: Hardy-Weinberg Equilibrium for evolution, solute potential for free energy, Chi-Squared analysis for information, and exponential and logistic growth models for predicting population changes.
How can the Hardy-Weinberg Equilibrium be used to determine allele frequencies in a population?
-The Hardy-Weinberg Equilibrium can be used to calculate the frequency of alleles (q) in a population by using the formula q^2 + 2pq + p^2 = 1, where q and p represent the frequencies of the two alleles. By measuring the phenotypic frequencies, one can solve for the allele frequencies.
What is the Rule of Thirty mentioned in the video and how does it predict life patterns?
-The Rule of Thirty is a simple mathematical pattern created by Stephen Wolfram that can predict complex biological patterns, such as the growth patterns of ferns or the shell patterns on a cone snail. It demonstrates the power of mathematics in predicting natural phenomena.
How does Mr. Andersen suggest students prepare for the AP Biology test regarding mathematical skills?
-Mr. Andersen suggests that students should familiarize themselves with the formula sheet provided for the AP Biology test and practice using a calculator to apply mathematical routines, equations, and algorithms to biological scenarios.
What are the three mathematical understandings Mr. Andersen mentions that students should have?
-The three mathematical understandings are: 1) Justifying the selection of a mathematical routine, 2) Applying mathematical algorithms or equations to solve biological problems, and 3) Estimating quantities that describe natural phenomena using mathematical models.
How does Mr. Andersen use the example of the peppered moth to illustrate the application of mathematics in biology?
-Mr. Andersen uses the example of the peppered moth's coloration change during the Industrial Revolution to demonstrate how to calculate allele frequencies using the Hardy-Weinberg Equilibrium. By analyzing the population percentages of dark and light moths over time, students can estimate the frequency of the light allele (q).
What is the concept of osmosis as applied in the dialysis tube example in the script?
-Osmosis is the movement of water across a semipermeable membrane from a region of lower solute concentration to a region of higher solute concentration. In the dialysis tube example, the change in mass of the tube is predicted based on the difference in solute concentrations inside and outside the tube, illustrating the principles of osmosis.
How does Charles Darwin's perspective on mathematics relate to the study of biology?
-Charles Darwin believed that every new body of discovery is mathematical in form, suggesting that mathematics provides a fundamental framework for understanding natural phenomena. He also suggested that mathematics gives one a new sense, implying that it enhances one's ability to perceive and analyze biological processes.
Outlines
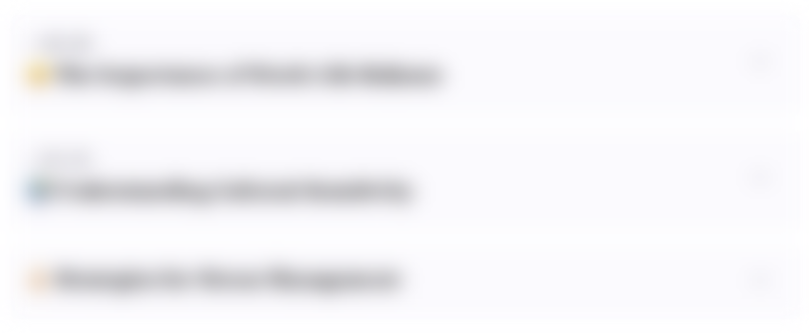
This section is available to paid users only. Please upgrade to access this part.
Upgrade NowMindmap
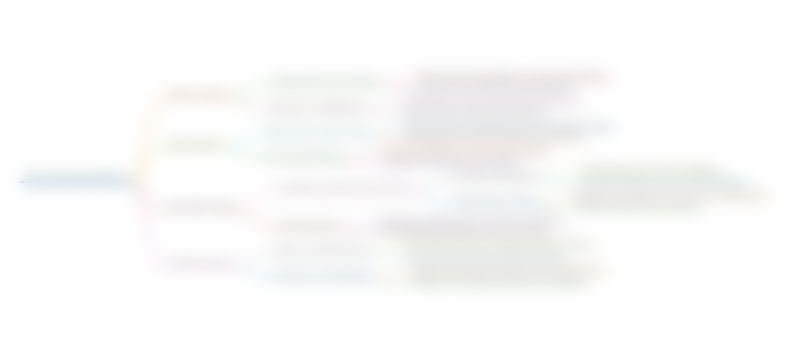
This section is available to paid users only. Please upgrade to access this part.
Upgrade NowKeywords
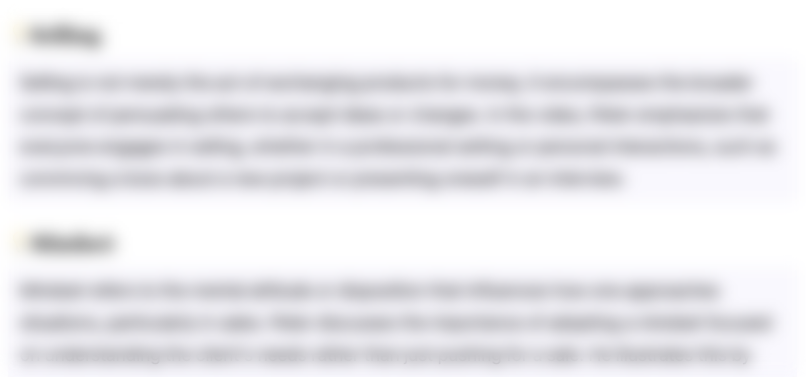
This section is available to paid users only. Please upgrade to access this part.
Upgrade NowHighlights
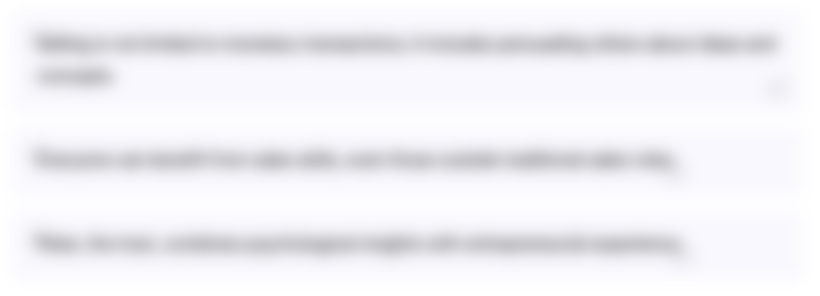
This section is available to paid users only. Please upgrade to access this part.
Upgrade NowTranscripts
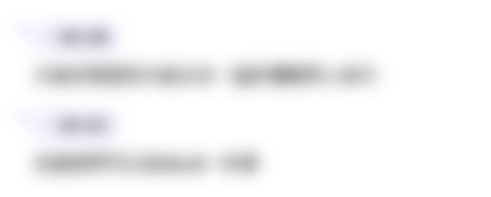
This section is available to paid users only. Please upgrade to access this part.
Upgrade Now5.0 / 5 (0 votes)