Strength of Materials - Stress
Summary
TLDRThis video introduces the fundamental concept of stress in the study of materials. The instructor begins by explaining the role of external loads in causing deformation in materials, highlighting the difference between elastic and plastic deformation. The video then defines stress as the internal resistance per unit area that a material offers to deformation. The instructor also discusses the mathematical formula for stress and its units, explaining the relationship between Newtons, Pascals, and other measurements commonly used in engineering. The video sets the stage for deeper exploration of different types of stresses in future episodes.
Takeaways
- 📚 The subject of strength of materials is introduced, focusing on understanding stresses and strains.
- 🔍 Stress is defined as the fundamental topic in materials science that needs to be understood to grasp the subject.
- 📐 The concept of 'load' is central to understanding stress; it's the external force applied to a body causing deformation.
- 🔨 Deformation is undesirable in engineering as it implies a change in the shape or size of the material, categorized into elastic and plastic deformation.
- 🧱 Stress is the resistance per unit area provided by the material to resist deformation, symbolized by the Greek letter Sigma (Σ).
- 📉 The formula for stress is mathematically expressed as the ratio of the resisting force (ΣFR) to the area (a), i.e., Σ = ΣFR / a.
- ⚖️ The body under stress is in static equilibrium, meaning the internal resistive forces are equal to the external load (P = ΣFR).
- 🔢 The SI unit for stress is Newtons per square meter (N/m²), which is equivalent to Pascal (Pa).
- 📏 In engineering, due to the smaller scales involved, the unit of stress is often given in Newtons per square millimeter (N/mm²), which is 1 Megapascal (MPa).
- 🔑 The understanding of stress involves recognizing it as an internal resistive force per unit cross-sectional area of a body under load.
- 🚀 The video promises to explore different types of stresses corresponding to different kinds of loads in the next installment of the series.
Q & A
What is the fundamental concept in the subject of Strength of Materials introduced in this video?
-The fundamental concept introduced is stress, which is crucial to understanding the entire subject of Strength of Materials.
How is stress related to the concept of load in materials?
-Stress is produced when an external load is applied to a body, causing deformation. Stress is the resistance per unit area that the material provides against this deformation.
What happens to a material when an external load is applied?
-When an external load is applied, the material undergoes deformation. This deformation is resisted by the internal forces of the material, leading to the development of stress.
How is stress mathematically defined?
-Stress is mathematically defined as the internal resistive force per unit cross-sectional area of a body. It is represented by the Greek letter Sigma (σ) and can be calculated using the formula σ = F/A, where F is the internal resistive force and A is the cross-sectional area.
What is the significance of static equilibrium in the context of stress?
-Static equilibrium is important because it indicates that the sum of the internal resistive forces in the material equals the external load applied to it, ensuring that the body remains stationary and stress is distributed evenly.
What units are used to measure stress, and how are they related?
-Stress is measured in units of Newtons per square meter (N/m²) in SI units, also known as Pascals (Pa). In engineering, stress is often measured in Newtons per square millimeter (N/mm²), which is equivalent to Megapascals (MPa). 1 N/mm² is equal to 1 MPa or 1 million Pascals.
Why is it important to understand the conversion between meters and millimeters when calculating stress?
-Understanding the conversion between meters and millimeters is important because mechanical engineering often deals with components measured in millimeters. Knowing that 1 meter equals 1000 millimeters helps in accurately converting and calculating stress in practical applications.
What are the two types of deformation mentioned, and how do they differ?
-The two types of deformation mentioned are elastic deformation and plastic deformation. Elastic deformation is temporary and reversible, meaning the material returns to its original shape after the load is removed. Plastic deformation, on the other hand, is permanent and irreversible, meaning the material remains deformed even after the load is removed.
How does the internal structure of a material resist applied loads?
-The internal structure of a material resists applied loads through the collective action of its fibers. These fibers generate internal resistive forces that counteract the external load, preventing deformation or minimizing it.
What is the practical significance of understanding stress in engineering?
-Understanding stress is crucial in engineering because it helps in designing materials and structures that can withstand external loads without undergoing undesirable deformations. It ensures the safety, durability, and reliability of engineering components and structures.
Outlines
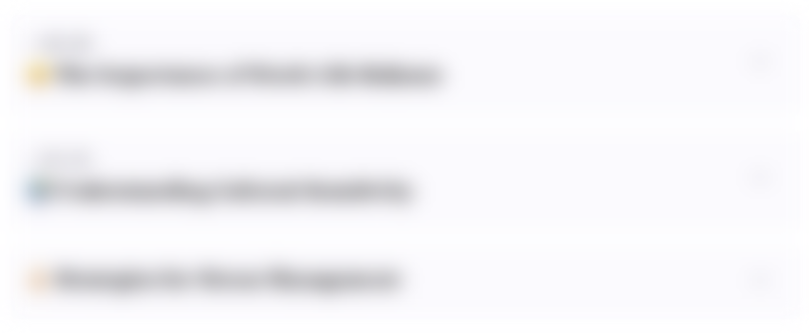
This section is available to paid users only. Please upgrade to access this part.
Upgrade NowMindmap
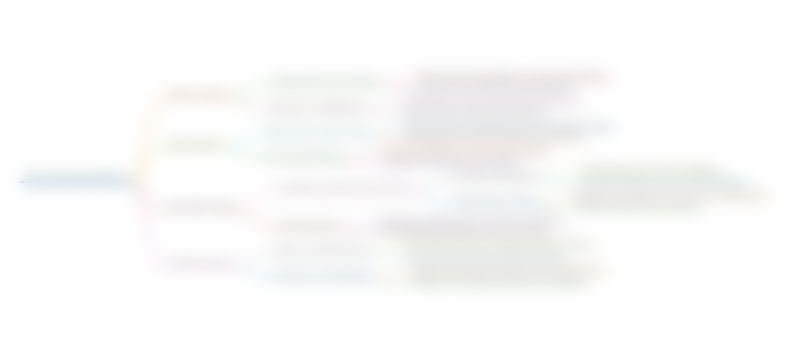
This section is available to paid users only. Please upgrade to access this part.
Upgrade NowKeywords
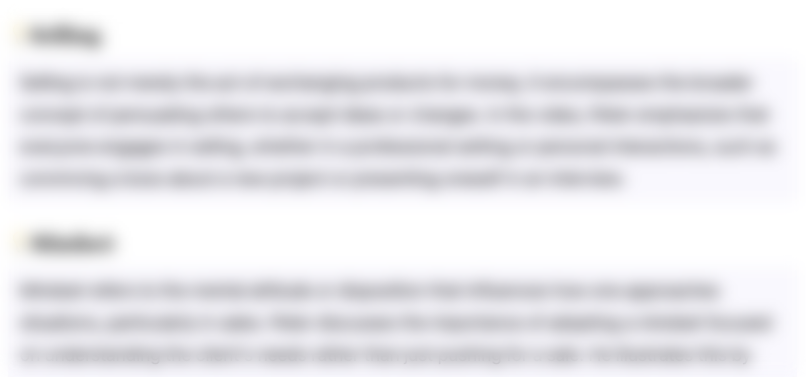
This section is available to paid users only. Please upgrade to access this part.
Upgrade NowHighlights
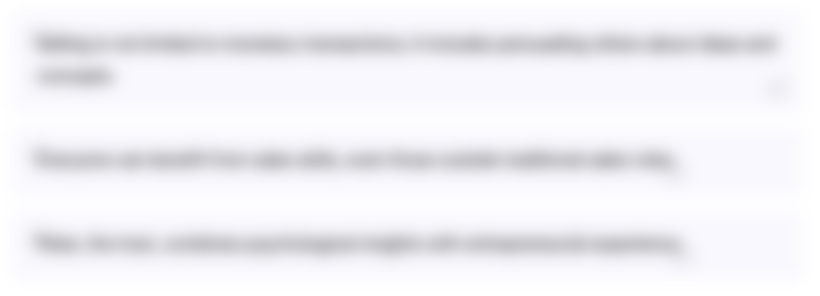
This section is available to paid users only. Please upgrade to access this part.
Upgrade NowTranscripts
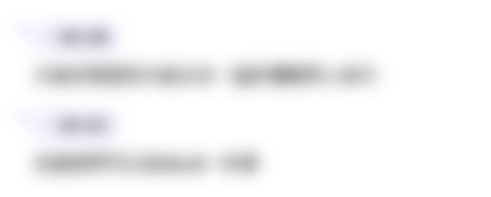
This section is available to paid users only. Please upgrade to access this part.
Upgrade NowBrowse More Related Video
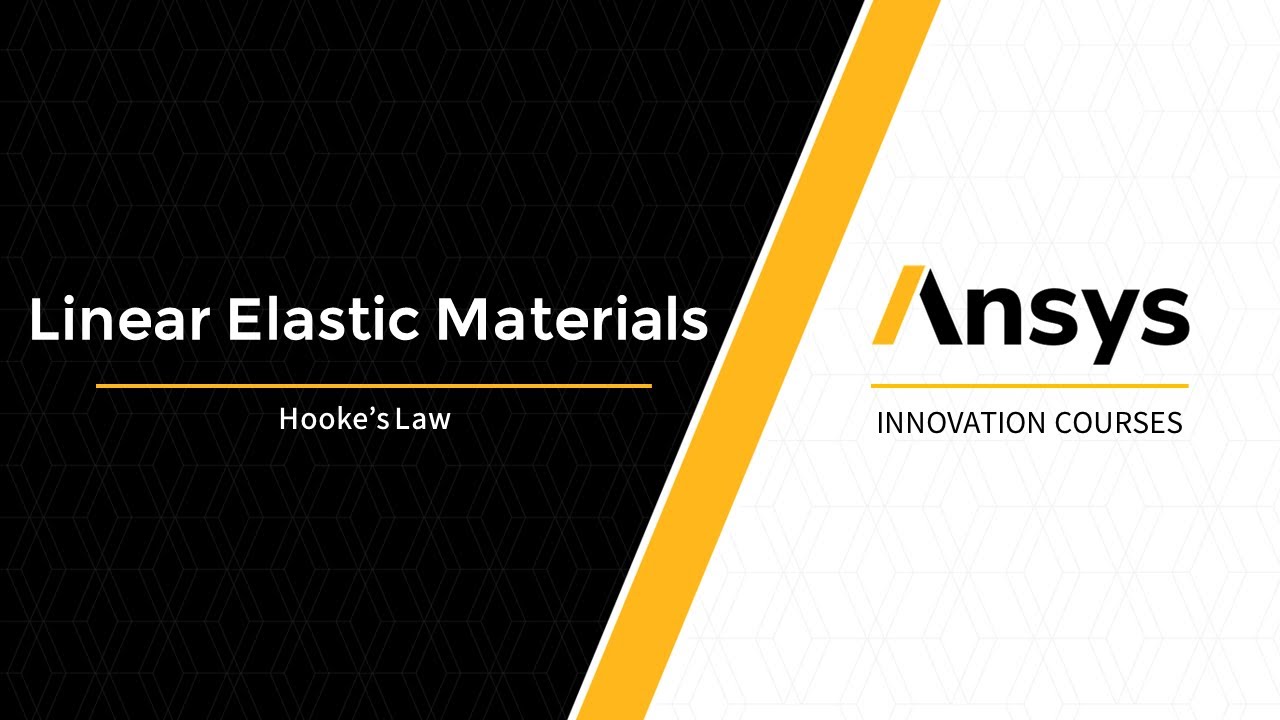
Hooke’s Law — Lesson 2

Stress and Local Equilibrium — Course Summary

Mengetahui Sistem Klasifikasi Perpustakaan | oleh : Lucky Audrylya Mahatan
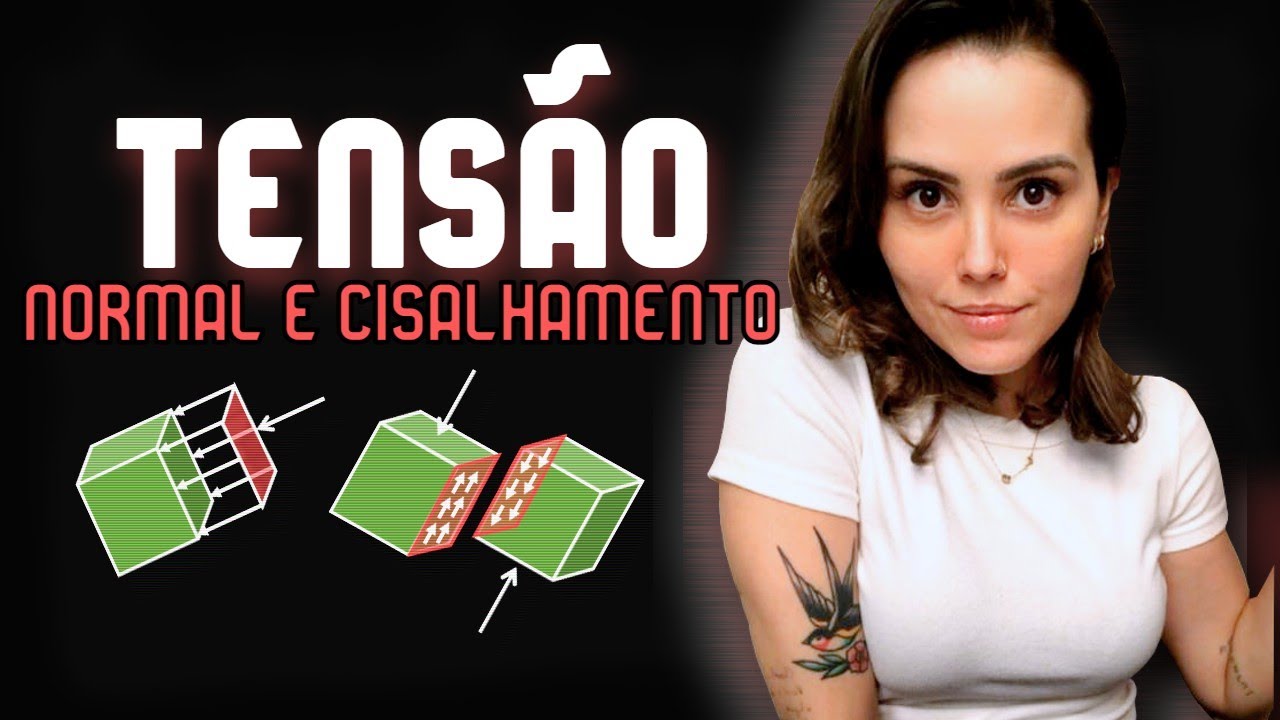
Aprenda agora o que é tensão na resistência dos materiais (ResMat)
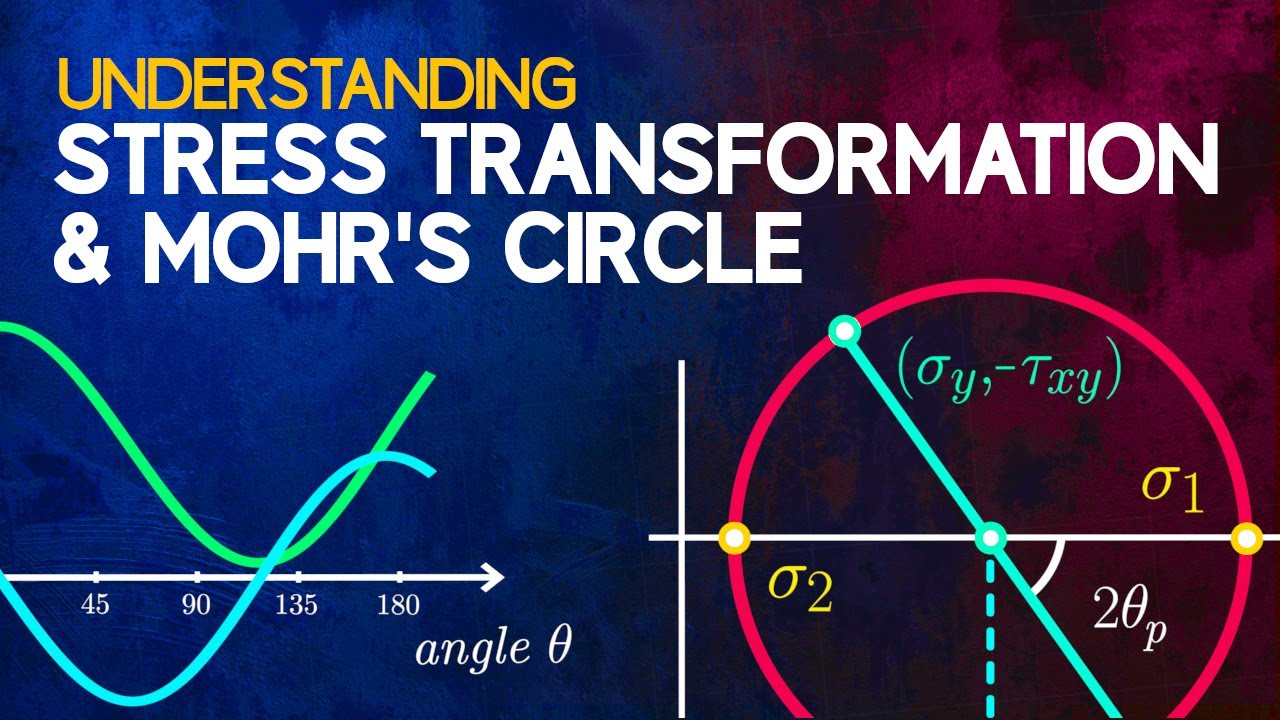
Understanding Stress Transformation and Mohr's Circle
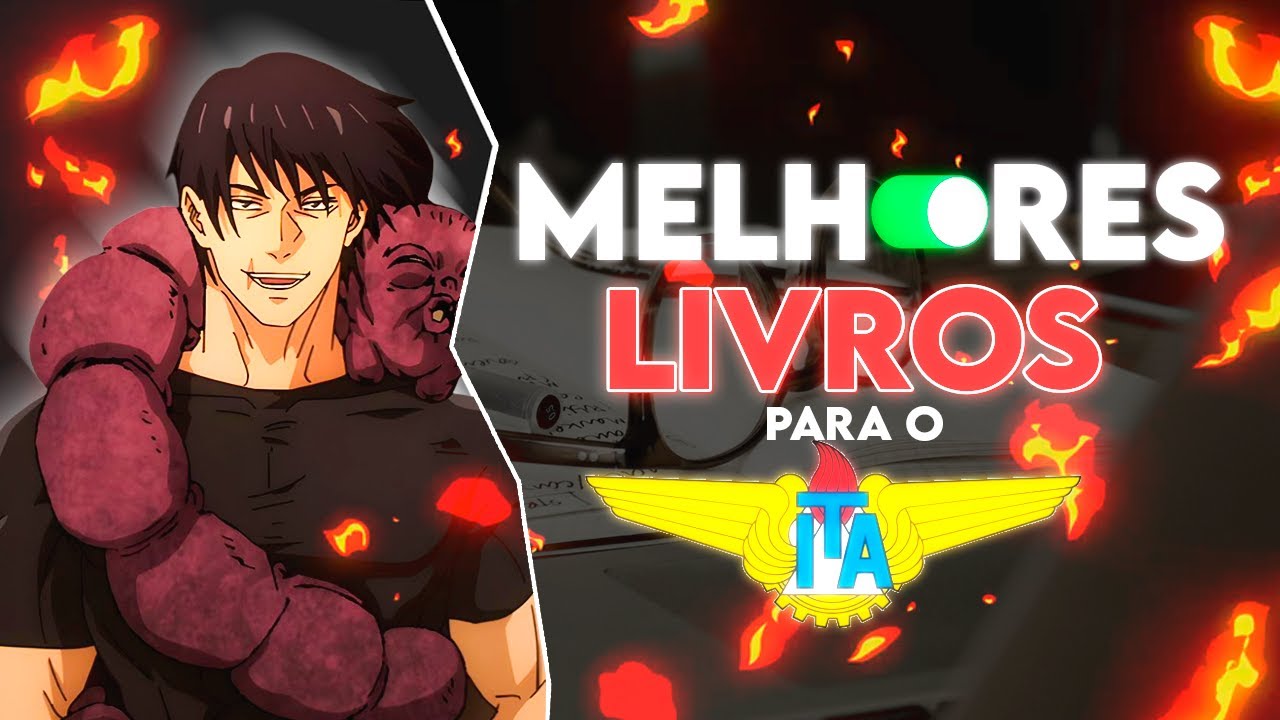
COMO SER O MELHOR NAS EXATAS E QUAIS LIVROS USAR NA PREPARAÇÃO? (livros que usei pra passar no ITA)
5.0 / 5 (0 votes)