An Overview of Earth’s Layers
Summary
TLDRThis script delves into Earth's layered structure, contrasting the continental and oceanic crusts and their roles in the subduction process. It explains the mantle's convection and its influence on plate tectonics, driven by slab-pull forces. The mysterious D'' layer's potential as a 'slab graveyard' and source of mantle plumes is highlighted. The geodynamo's role in maintaining Earth's magnetic field through the liquid outer core is unveiled, along with the inner core's super-rotation and implications for magnetic field reversals. The script concludes with Earth's formation, differentiation, and the gradual solidification of its inner core, hinting at a future without Earth's magnetic field.
Takeaways
- 🌏 The Earth's crust is divided into continental and oceanic types, with continental crust being felsic and thicker, while oceanic crust is mafic and thinner.
- 🔍 Oceanic crust is denser than continental crust, leading to subduction where oceanic plates slide beneath continental plates.
- 🏔 Cratons are ancient continental crust areas that are 4 billion years old, whereas the oldest oceanic crust is only 340 million years old due to constant subduction and destruction.
- 🔥 The mantle lies beneath the crust, with temperatures reaching 3500 degrees Celsius and pressures a million times greater than the atmosphere, and it convects slowly over millions of years.
- 🌋 Mid-ocean ridges and rift zones are formed by upwelling mantle, while subduction zones are associated with sinking cold mantle.
- 🌀 Slab-pull force, driven by buoyancy, is the primary force behind plate tectonics, initiated when oceanic crust becomes denser than the underlying mantle.
- 💎 The mantle is primarily composed of peridotite, an ultramafic rock, and diamonds form at depths of about 150 kilometers or more within it.
- 🌌 The D'' layer at the bottom of the mantle is a mysterious region with a large thermal gradient and increased heterogeneity, possibly acting as a 'slab graveyard' or the origin of mantle plumes.
- 🌐 The Earth's magnetic field is generated by the geodynamo effect in the liquid outer core, which is mainly composed of iron and nickel, and is influenced by the Earth's rotation.
- 🌀 The inner core, about the size of the moon, is solid and helps stabilize the magnetic field, although it does not generate it itself and rotates faster than the Earth as a whole.
- 🌐 Earth's magnetic field undergoes periodic reversals, the triggers of which are still unknown, but may involve the collapse of subducted slabs into the D'' layer.
Q & A
What are the two types of Earth's crust and how do they differ in composition and thickness?
-The two types of Earth's crust are continental and oceanic. Continental crust is felsic, rich in silica, and is approximately 20 to 70 kilometers thick. Oceanic crust, on the other hand, is mafic, rich in iron and magnesium, and is about 5 to 10 kilometers thick.
Why is oceanic crust denser than continental crust, and what is the geological process that occurs when they collide?
-Oceanic crust is denser due to its higher content of iron and magnesium. When oceanic crust collides with continental crust, the denser oceanic crust subducts beneath the continental crust in a process known as subduction.
What is the significance of the age difference between the oldest continental and oceanic crusts?
-The age difference signifies the recycling of oceanic crust through subduction. Continental crust can be as old as 4 billion years, while the oldest oceanic crust is only 340 million years old, indicating that oceanic crust is constantly being created at mid-ocean ridges and destroyed through subduction.
What are the primary components of Earth's mantle, and how does its behavior contribute to plate tectonics?
-The mantle is primarily composed of an ultramafic rock called peridotite, rich in the mineral olivine (MgFeSiO4). It behaves as a solid but is malleable and convects over millions of years, transferring heat to the surface. This convection, along with slab-pull forces, is the main driver of plate tectonics.
What is the role of the D'' layer in the context of Earth's mantle and geological phenomena?
-The D'' layer, located at the bottom of the mantle, is marked by a large thermal gradient and increased heterogeneity. It is hypothesized to be the 'slab graveyard' where subducted slabs settle, and possibly the source of mantle plumes, which can lead to the formation of large volcanoes and may have implications for magnetic field reversals.
How does the composition and state of Earth's outer core contribute to the creation of Earth's magnetic field?
-The outer core is a liquid composed mainly of iron and nickel. Its convection, facilitated by Earth's rotation, creates a geodynamo effect. The motion of the electrically conductive iron generates an electric field that strengthens the original magnetic field, thus maintaining Earth's magnetic field.
What is the geodynamo, and how does it relate to Earth's magnetic field?
-The geodynamo is the process by which the Earth's magnetic field is generated and sustained. It involves the convection of the liquid outer core and the motion of electrically conductive materials within Earth's magnetic field, creating an electric field that, in turn, generates an additional magnetic field.
What is the significance of the Bullen discontinuity, and how does it relate to the core's structure?
-The Bullen discontinuity marks the boundary between the liquid outer core and the solid inner core. At this depth, the pressure is so great that iron is forced into the solid phase, indicating a change in the physical state of the core materials.
How does the inner core's super-rotation affect Earth's magnetic field, and what causes it?
-The inner core's super-rotation, which is faster than Earth's overall rotation, is thought to be caused by rotating magnetic fields in the outer core exerting a magnetic torque on the inner core. This interaction helps lock-in and stabilize the magnetic field lines generated in the outer core.
What are magnetic field reversals, and what might trigger them?
-Magnetic field reversals are periodic events where Earth's magnetic field weakens to near zero and the poles switch. Failed reversals, where the original field reestablishes, can also occur. One hypothesis for triggering magnetic reversals is the sudden collapse of large amounts of cold, subducted slabs into the D'' layer, which can reorganize outer core convection and alter the magnetic field.
How did Earth's layers form, and what was the initial state of the planet?
-Earth initially formed as an extremely hot, homogeneous planet. Differentiation began as the planet cooled, with heavier materials sinking toward the center and lighter materials rising toward the surface. Over time, the crust and mantle crystallized, while the core remained molten. The inner core started to crystallize between 2 billion to 570 million years ago.
What is the current rate of growth of Earth's inner core, and what would be the long-term consequence if Earth were to exist for an extended period?
-The inner core is currently growing at a rate of a millimeter per year as the liquid iron in the outer core solidifies. If Earth were to exist for 91 billion years, it would eventually have an entirely solid iron core, and Earth's magnetic field would disappear. However, the Sun is expected to engulf Earth in its red giant phase before this scenario occurs.
Outlines
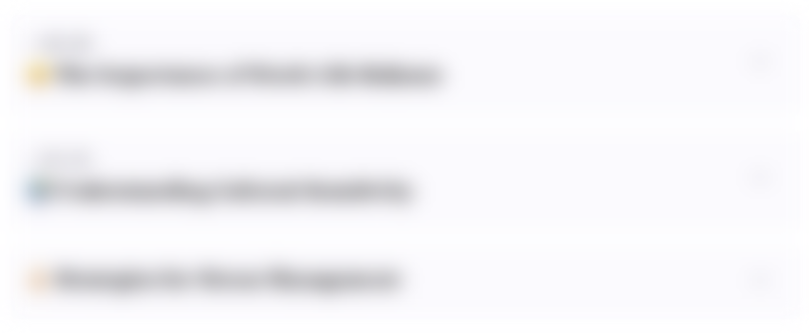
This section is available to paid users only. Please upgrade to access this part.
Upgrade NowMindmap
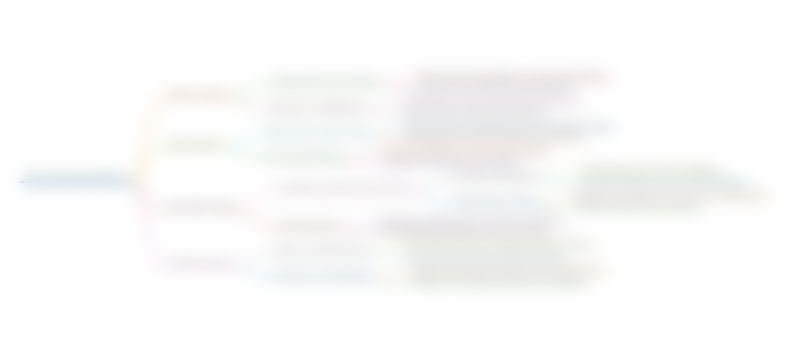
This section is available to paid users only. Please upgrade to access this part.
Upgrade NowKeywords
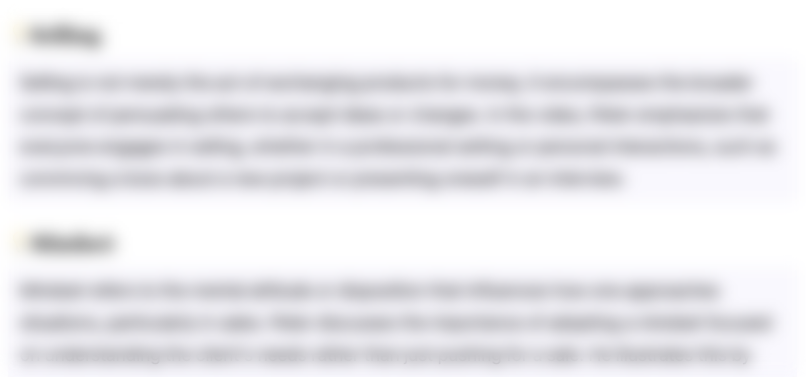
This section is available to paid users only. Please upgrade to access this part.
Upgrade NowHighlights
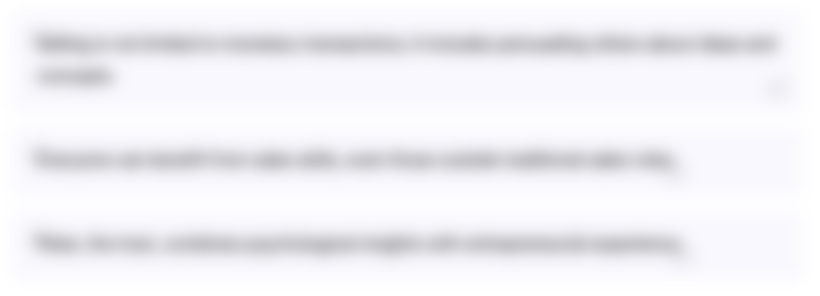
This section is available to paid users only. Please upgrade to access this part.
Upgrade NowTranscripts
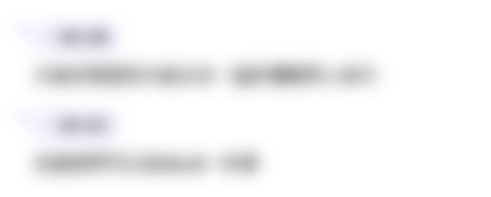
This section is available to paid users only. Please upgrade to access this part.
Upgrade NowBrowse More Related Video
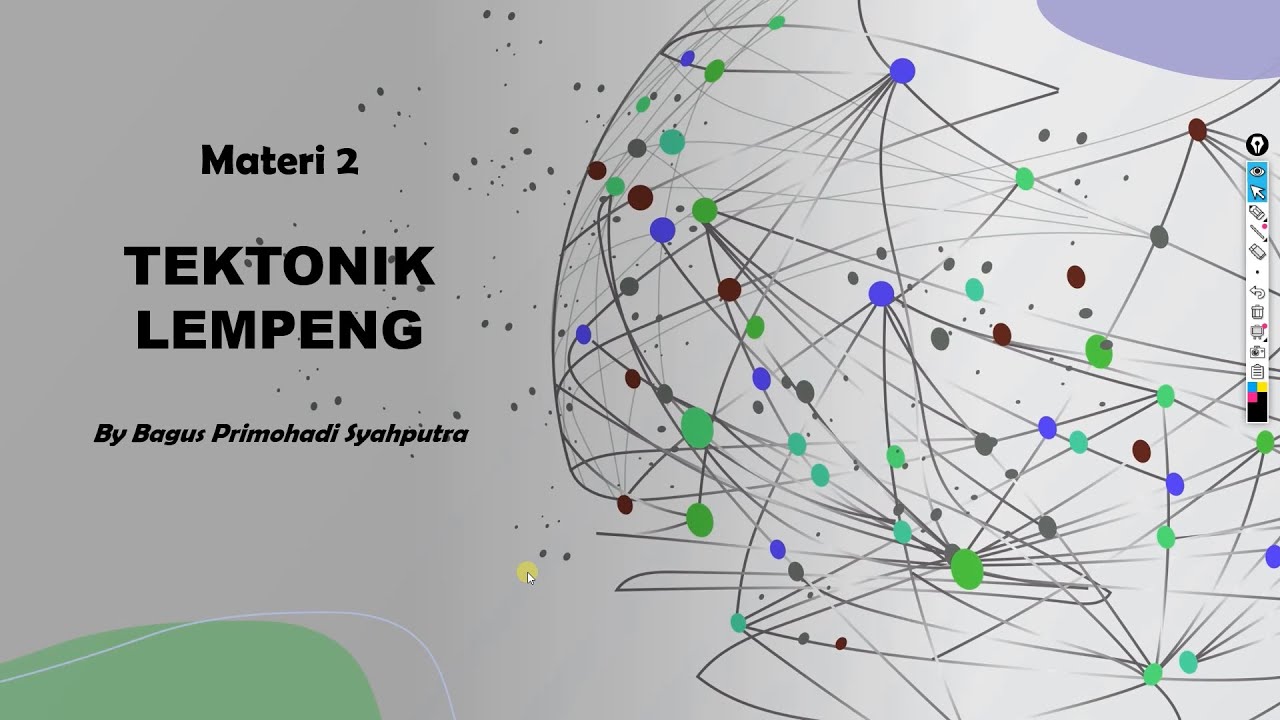
KEB02 Tektonik Lempeng | Materi OSN/KSN Kebumian SMA
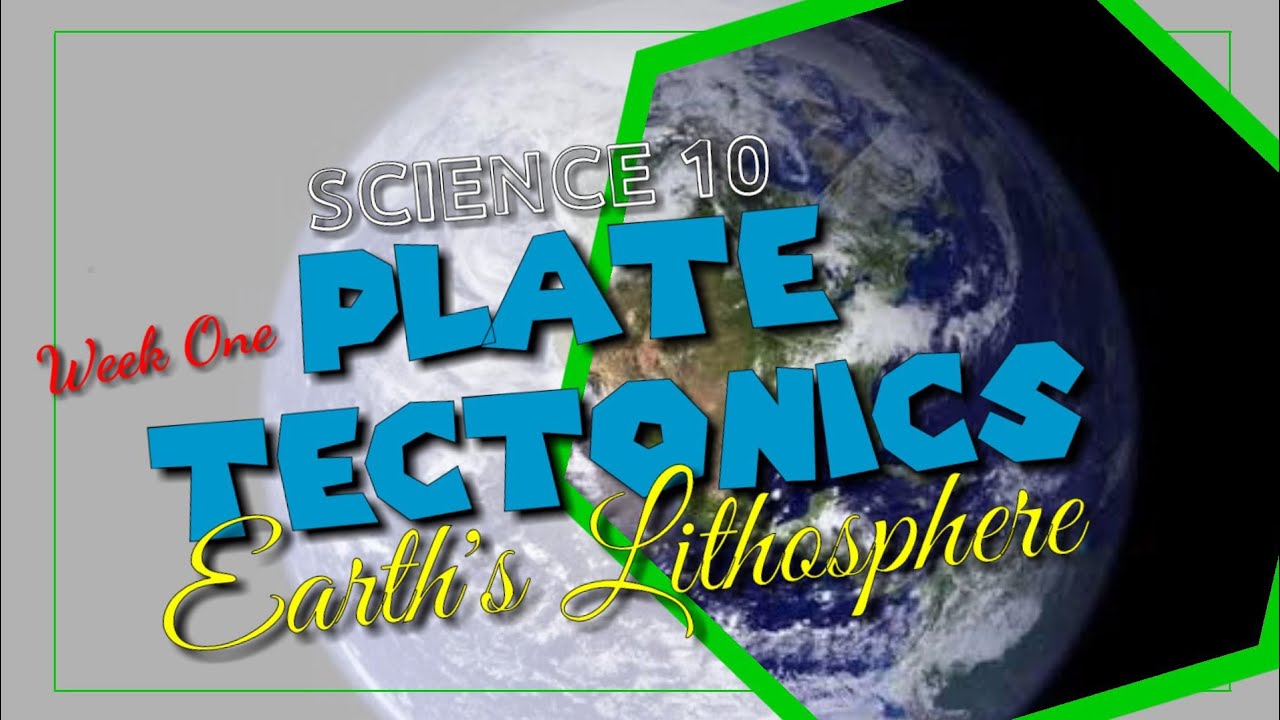
EARTH'S LITHOSPHERE | SCIENCE 10 - Week 1
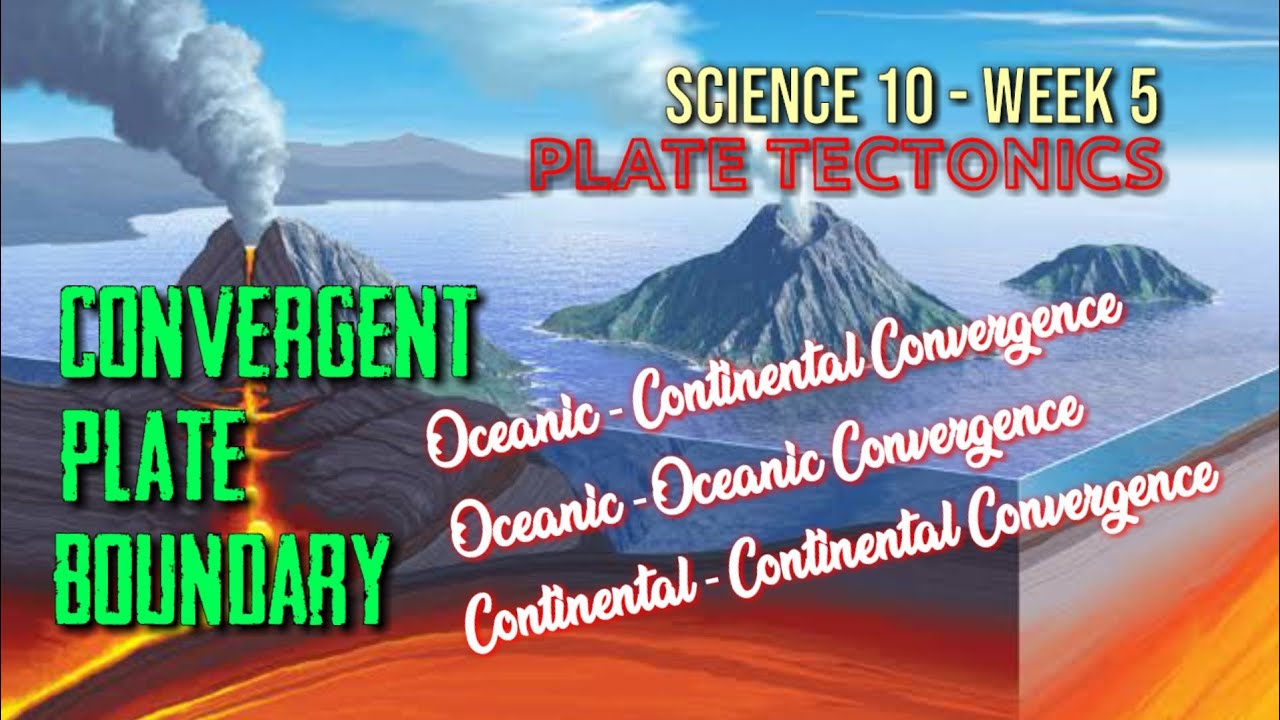
GEOLOGIC PROCESSES THAT OCCUR ALONG CONVERGENT PLATE BOUNDARIES | SCIENCE 10 - Week 5
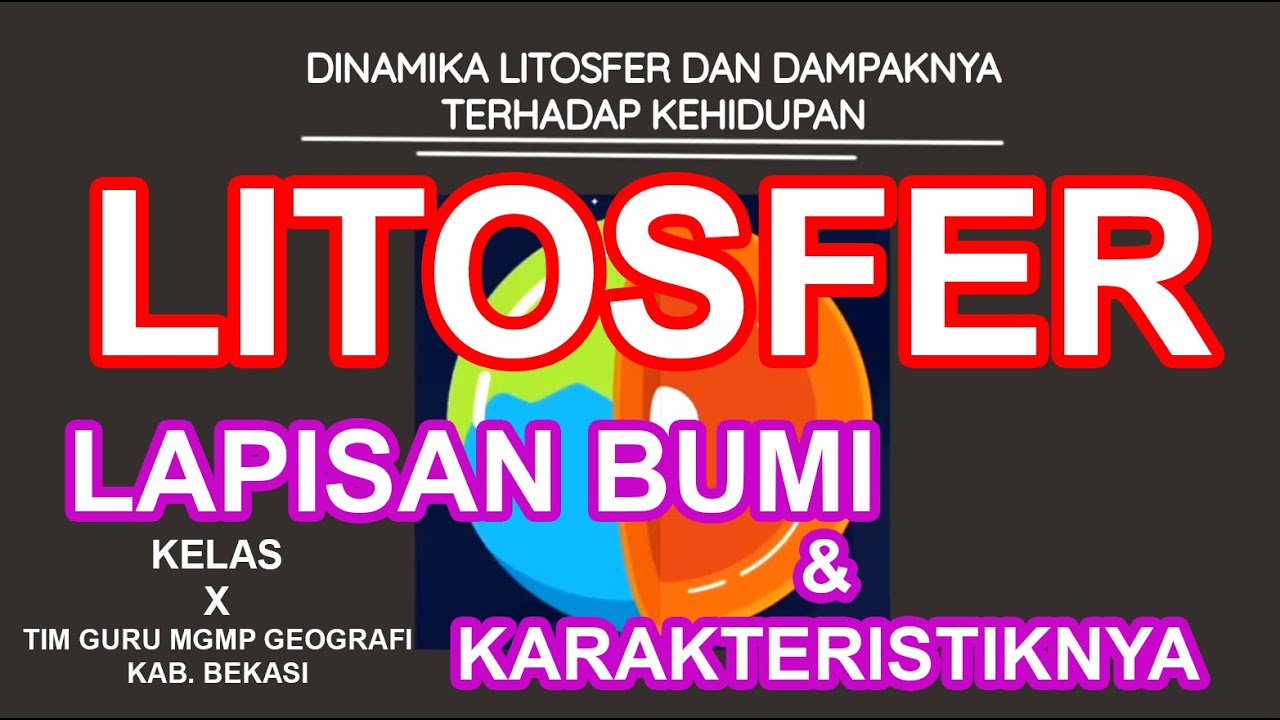
#1 #Kelas10 #Geografi #smstr2 | Lapisan Bumi dan Karakteristiknya

Grade 10 SCIENCE | Quarter 1 Module 5 (PART 1) | CONVERGENT BOUNDARY
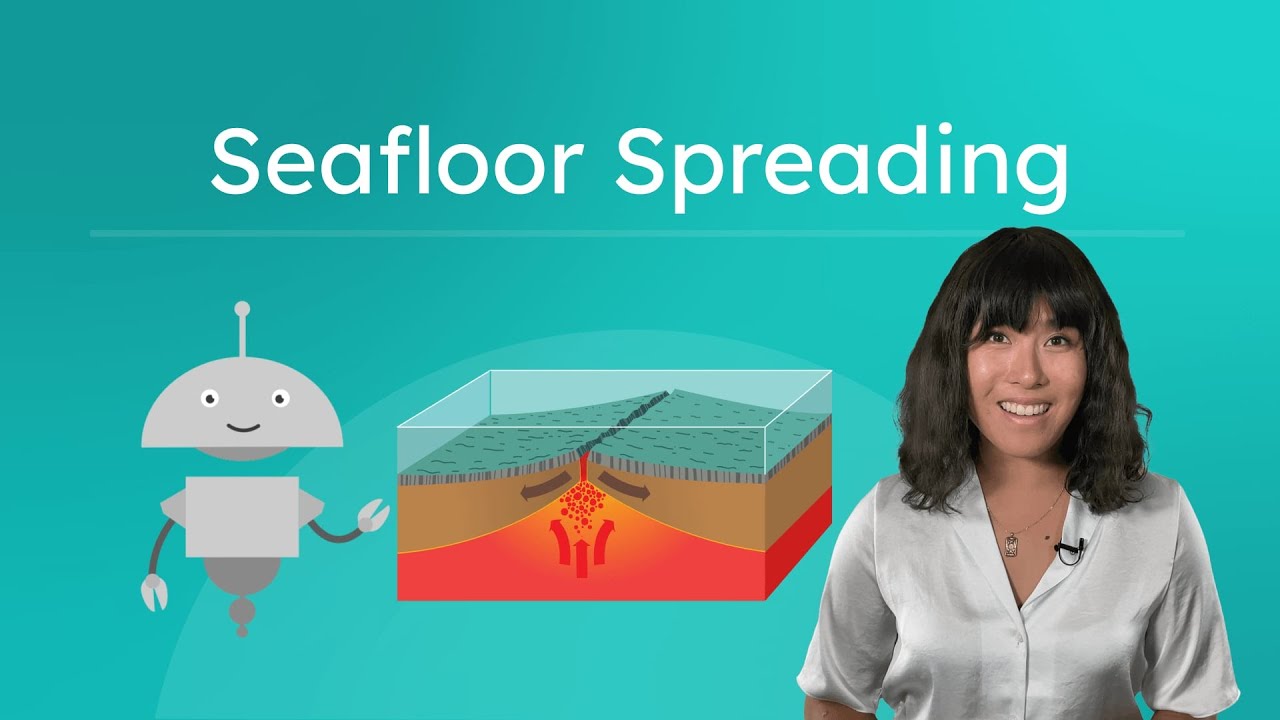
Seafloor Spreading - Earth Science for Kids!
5.0 / 5 (0 votes)