How to use MOSFET as a Switch ? MOSFET as a Switch Explained
Summary
TLDRThis video from the ALL ABOUT ELECTRONICS YouTube channel explains the use of MOSFETs as switches, highlighting their advantages over BJTs, particularly in high-frequency and power-efficient applications. It covers the basic operation of MOSFETs, their types, characteristics, and how to select them based on datasheet parameters. The script also discusses gate driving considerations, thermal stability, and the importance of total gate charge for fast switching applications, providing a comprehensive guide for electronics enthusiasts.
Takeaways
- 😀 The MOSFET is a voltage-controlled device that can be turned on or off by controlling the gate-to-source voltage, making it suitable for use as a switch.
- 🔌 In contrast to the MOSFET, the BJT is a current-controlled device that requires base current to be driven into saturation or cutoff for use as a switch.
- 💡 MOSFETs are easier to drive compared to BJTs, especially beneficial for applications requiring high drive currents or control by microcontrollers.
- 🚀 MOSFETs are more power efficient than BJTs in continuous switching applications due to their lower on-state drain-to-source resistance.
- 🔥 The switching loss of a MOSFET is lower than that of a BJT, making it preferable for high-frequency continuous switching applications.
- 🌡 MOSFETs exhibit positive temperature coefficient, which contributes to their thermal stability, unlike BJTs that have a negative temperature coefficient and risk of thermal runaway.
- 🛠 Enhancement type MOSFETs are typically used as switches due to their normally off state, as opposed to depletion type MOSFETs which are normally on.
- 📉 The RDS(ON) parameter is crucial for MOSFETs when used as a switch, as it represents the on-state resistance and affects conduction loss.
- 🔄 When using a MOSFET as a switch, it's important to consider the load line and operating point to ensure the MOSFET operates in the linear region for efficient switching.
- 🔌 A series resistor is recommended between the gate and control input to limit transient current and protect the control circuit when switching a MOSFET.
- ⏱ The total gate charge of a MOSFET is an important parameter for fast switching applications, as it affects the speed at which the MOSFET can be turned on or off.
Q & A
What is the primary function of a MOSFET in this video?
-The primary function of a MOSFET in this video is to be used as a switch, controlled by the gate to source voltage.
How does a MOSFET differ from a BJT in terms of control mechanism?
-A MOSFET is a voltage-controlled device, whereas a BJT is a current-controlled device. This means a MOSFET can be turned on or off by controlling the gate to source voltage, while a BJT requires base current to be driven into saturation or cutoff.
What are the advantages of using a MOSFET over a BJT for high current applications?
-For high current applications, a MOSFET is easier to drive and requires less base current compared to a BJT. Additionally, MOSFETs are more power efficient and thermally stable, making them preferable for high frequency switching.
Why is a MOSFET considered more thermally stable than a BJT?
-MOSFETs have a positive temperature coefficient, which means as the temperature increases, the on resistance increases, reducing the drain current and thus the temperature. In contrast, BJTs have a negative temperature coefficient, which can lead to thermal runaway if not properly managed.
What is the significance of the RDS(ON) parameter in MOSFETs?
-RDS(ON) represents the drain to source resistance when the MOSFET is in the on condition. A lower RDS(ON) value is desirable as it reduces the conduction loss in the MOSFET.
How does the threshold voltage of a MOSFET affect its operation as a switch?
-The threshold voltage determines the minimum gate to source voltage required to turn on the MOSFET. If it is low, the MOSFET can be easily operated using a microcontroller, making it suitable for switch applications.
What is the purpose of a series resistor when connecting a control signal to the gate of a MOSFET?
-A series resistor is used to limit the transient current that can be drawn by the gate during the charging of the gate-source capacitor, preventing damage to the control circuit.
Why is a pull-down resistor necessary at the gate terminal of a MOSFET?
-A pull-down resistor ensures that the MOSFET remains in the off condition during power-up of the control circuit, maintaining the default state until the control signal is applied.
How does the total gate charge of a MOSFET affect its switching speed?
-The total gate charge is the amount of charge needed to fully turn on the MOSFET. A lower total gate charge allows for faster switching, which is beneficial for high-frequency applications.
What is the relationship between the switching speed of a MOSFET and its switching loss?
-Faster switching speeds in a MOSFET reduce the time during which both drain current and voltage are present, thus reducing the switching loss and improving efficiency.
How can the load line be determined when using a MOSFET as a switch?
-The load line can be determined by considering the maximum current through the load when the drain-source voltage is zero and the drain-source voltage when the current is zero, connecting these two points to form the line.
Outlines
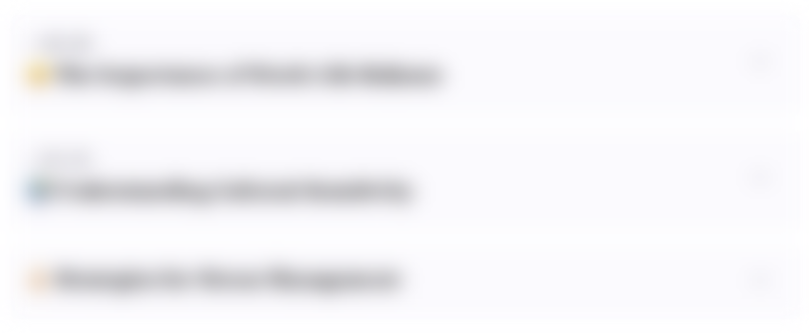
This section is available to paid users only. Please upgrade to access this part.
Upgrade NowMindmap
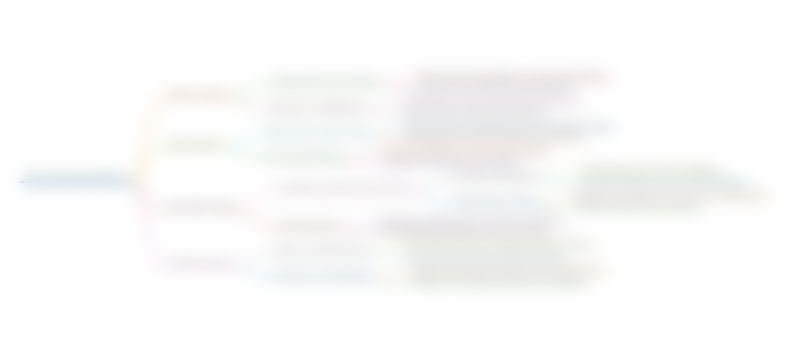
This section is available to paid users only. Please upgrade to access this part.
Upgrade NowKeywords
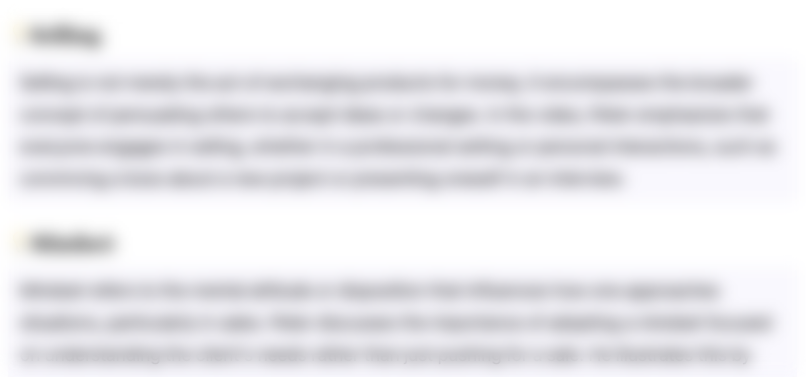
This section is available to paid users only. Please upgrade to access this part.
Upgrade NowHighlights
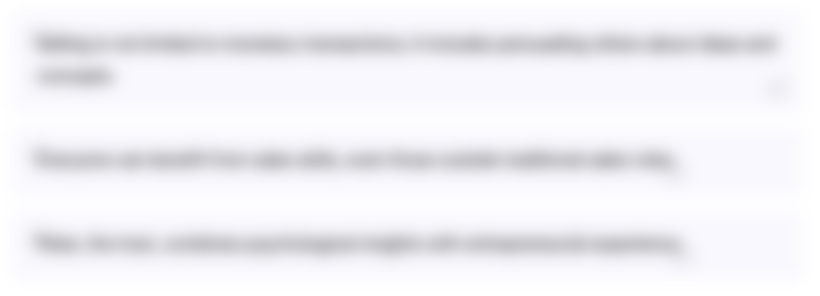
This section is available to paid users only. Please upgrade to access this part.
Upgrade NowTranscripts
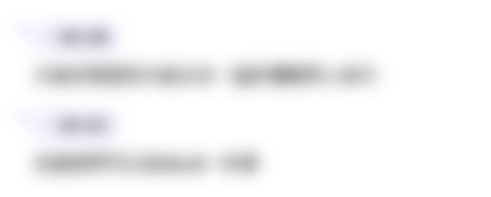
This section is available to paid users only. Please upgrade to access this part.
Upgrade NowBrowse More Related Video
5.0 / 5 (0 votes)