Breaking Old Chip Physics with New 2D Materials
Summary
TLDRA new research from UC Santa Barbara presents a breakthrough in transistor technology, potentially reducing power consumption by 70%. The study explores the use of 2D materials in tunneling Field Effect transistors (TFETs), which require less voltage to operate, significantly lowering the energy barrier for electron flow. This innovation could revolutionize power efficiency in devices from smartphones to AI chips, although challenges in manufacturing and cost remain before widespread adoption.
Takeaways
- π The script discusses the importance of performance, power consumption, cost, and availability in modern device usage, with a focus on reducing power consumption through transistor innovation.
- π§ It highlights the fundamental limits in the physics of transistors, specifically the 60 mV per decade limit that has been a constraint for over 30 years in modern chip designs.
- π A new research paper from UC Santa Barbara presents breakthrough findings that could significantly lower power consumption in transistor-based devices.
- π The script explains the binary nature of transistor switches and their power cost in turning on and off, with the reality being more analog than digital.
- π¬ The research introduces a t-FET (tunneling Field Effect transistor) and its use of 2D materials to lower the energy barrier for electron flow, resulting in reduced power requirements.
- π The energy diagram comparison between standard MOSFETs and t-FETs illustrates the reduced energy requirement for electron tunneling in the latter.
- π The research achieved an 18 mV per decade performance, marking a 70% decrease in the active voltage needed for modern processors, which could benefit a wide range of devices.
- π οΈ The script mentions that while the technology is promising, it is still in the research phase and not yet ready for mass production or widespread adoption.
- π One of the limitations of the new t-FETs is their significantly slower operation speed compared to finFETs at equivalent voltages.
- β‘οΈ However, the t-FETs show extremely low static power consumption, with a billion transistors consuming only 0.22 microwatts, compared to 1.54 watts for finFETs.
- π‘ The potential application of these new transistors in neuromorphic chips is discussed, with the technology showing thousands of times lower power per neuron fired at low activity levels.
Q & A
What are the two main aspects of power consumption in electronic devices?
-The two main aspects of power consumption are peak power, which is the power consumed during operation, and idle power, which is the power consumed when the device is not in use.
What is the fundamental limit in the physics of transistors that has been a challenge for over 30 years?
-The fundamental limit is the 60 mV per decade voltage requirement for transitioning the electron flow in a standard MOSFET, which contributes significantly to power consumption in modern chips.
What is the significance of the research paper from UC Santa Barbara in the context of transistor design?
-The research paper showcases a breakthrough in reducing power consumption by introducing a new design for transistors that lowers the power requirements for turning them on and off.
How do modern computer chips, GPUs, and wafer-scale devices differ in the number of transistors they contain?
-Modern computer chips contain millions of transistors, GPUs contain billions, and wafer-scale devices, like the Broadcom Tomahawk 4, can contain trillions of transistors.
What is the role of doping in the creation of a MOSFET?
-Doping is a process where silicon atoms are removed or replaced with elements like boron or phosphorus to adjust the structure of the silicon, making it electron-rich or electron-deficient, which is essential for the functioning of a MOSFET.
What is the significance of the energy diagram in understanding electron transport in a transistor?
-The energy diagram represents the energy levels of electrons, showing how the energy barrier is reduced by applying voltage to allow electrons to flow between the source and drain in a transistor.
What is a Tunneling Field Effect Transistor (TFET) and how does it differ from a standard MOSFET?
-A TFET is a type of transistor that uses quantum tunneling to allow electrons to move from one end of the transistor to the other without a physical path. It differs from a standard MOSFET by doping the source and drain with opposite polarity, which predisposes the flow of electrons but requires a lower voltage to enable tunneling.
What is the key advantage of using 2D materials in the TFET design presented in the research paper?
-The key advantage is that the 2D materials used in the TFET design allow for a significant reduction in the voltage required for electron tunneling, achieving an 18 mV per decade performance, which is a 70% decrease compared to the standard 60 mV per decade in MOSFETs.
How does the new TFET design impact the power consumption of neuromorphic chips?
-The new TFET design, when modeled as part of a digital neuromorphic chip, showed a power consumption that is several thousand times lower per neuron fired at low activity, making it highly efficient for low-power applications.
What are some of the barriers to high-volume adoption of the new TFET technology presented in the research paper?
-Some barriers include the specialized manufacturing techniques required for 2D materials, which are not yet standardized for mass production, and the higher cost associated with low-volume production compared to the optimized processes of current technologies.
Outlines
π Transistor Power Consumption and Design
This paragraph discusses the importance of performance and power consumption in modern electronic devices, emphasizing the cost and availability of these technologies. It explains the concept of peak and idle power consumption, which are dependent on the voltage required to switch transistors between states. The script introduces a research paper from UC Santa Barbara that aims to reduce power consumption in transistors. It also touches on the limitations of current transistor design, known as MOSFETs, and the fundamental physics involved in their operation. The paragraph concludes with an introduction to the concept of energy diagrams and the potential for new research to overcome the 60 mV per decade limit that has been a constant in chip design for over 30 years.
π Tunneling FETs and 2D Materials for Power Efficiency
The second paragraph delves into the specifics of tunneling Field Effect transistors (TFETs), which are a type of transistor that allows for electrons to move between states through quantum tunneling, rather than thermal diffusion. It explains how TFETs work with a different doping process that creates an electron-rich and electron-deficient environment within the transistor, facilitating the tunneling effect. The paragraph highlights the research's use of a 2D channel material, which has enabled a significant reduction in the voltage required for electron transition, from 60 mV per decade to 18 mV per decade, a 70% decrease. This advancement could lead to substantial improvements in power efficiency across various devices, from AI chips to smartphones. The script also discusses the potential and current limitations of 2D materials in manufacturing, including the challenges of scaling and cost.
π The Future of Transistors and Neuromorphic Chips
The final paragraph reflects on the potential impact of the new transistor technology on the future of computing, particularly in the area of neuromorphic chips, which are designed to mimic the neural structure of the human brain. It mentions a comparison between the new 2D TFET technology and current finFET devices, showing a significant reduction in power consumption, albeit with a trade-off in speed. The script also discusses the barriers to widespread adoption of this technology, including manufacturing challenges and cost. It concludes with a broader perspective on the evolution of transistor technology and the importance of revisiting and rebuilding the foundations of chip architecture to continue advancing the field.
Mindmap
Keywords
π‘Transistor
π‘Power Consumption
π‘MOSFET
π‘Doping
π‘Voltage
π‘Tunneling FET
π‘2D Material
π‘Quantum Tunneling
π‘Neuromorphic Chip
π‘Manufacturing Methods
π‘Cost
Highlights
New research from UC Santa Barbara aims to reduce power consumption in transistor-based devices.
The power consumption of transistors is tied to the voltage needed to flip the state of the transistors from 0 to 1.
A fundamental limit in physics has restricted the power efficiency of transistors for over 30 years, known as the 60 mV per decade limit.
Modern computer chips, GPUs, and other devices use billions to trillions of transistors, each with a power cost when switched.
Transistors work by applying an electric field to allow electron flow, reducing energy barriers through applied voltage.
MOSFETs (Metal Oxide Semiconductor Field Effect transistors) are the current standard for transistors, with a design limited by the 60 mV per decade rule.
UC Santa Barbara researchers have developed a new approach using a tunneling Field Effect transistor (TFET) for lower power consumption.
TFETs work on the principle of quantum tunneling, allowing electrons to move without a physical path by applying a voltage.
The energy required for electron tunneling in TFETs is significantly lower than in standard transistors, offering a 70% decrease in active voltage.
The research introduces a 2D channel material that enables the 18 mV per decade performance, a substantial improvement over the traditional limit.
2D materials, such as transition metal dichalcogenides (TMDs), offer unique properties for transistor design due to their limited Z-direction scaling.
The new TFET design was compared to 7nm FinFET devices, showing a significant reduction in static power consumption.
While the TFET operates at a slower speed, its ultra-low power consumption is ideal for neuromorphic computing applications.
The research paper models the TFET as part of a digital neuromorphic chip, demonstrating its potential for future low-power devices.
There are challenges in manufacturing these new transistors at scale, including specialized techniques for 2D materials.
Cost is a barrier to adoption, as low-volume production of new technology is typically more expensive.
The potential for fundamentally changing the physics of transistors could pave the way for more efficient future hardware.
Transcripts
for most of us who use devices from
day-to-day there are two things that
matter most performance and power beyond
that there's the cost and when exactly
can I get my hands on it the topic of
power often has two main angles the peak
power consumed and the idle power
consumed both of those rely on how much
voltage is needed to convert the
transistors the switches in Silicon
chips inside from a zero to a one and
vice versa despite advances in
manufacturing and packaging there has
been a fundamental limit in the physics
of that 0 to one to zero flip a new
research paper from UC Santa Barbara is
showcasing breakthrough numbers to help
lower the power consumption of anything
with a
transistor what's your minimum
specification so modern computer chip
has millions transistors or in the case
of gpus billions or in the case of wave
scale dinner plates trillions this is
the broad broadcom Tomahawk 4 and this
has billions of transistors each
transistor is a switch and there's a
power cost turning it on and off we
often like to think that a switch is a
binary one or zero in a very digitall
likee context and for the most part it
makes everything we talk about a lot
simpler however the reality is
definitely a bit more analog than that
for this video we're going to talk about
transistor design the physics of of
electrons and there'll be some graphs as
well this is still research but I'll
take you through let's start with what
we need in a switch in a standard
computer chip a switch is enabled by
allowing electrons to flow between two
locations in order to get electrons to
flow we apply an electric field
attracting electrons to flow through a
material it lowers the energy barrier
for those electrons to flow the barrier
is reduced by orders of magnitude due to
this applied voltage and what might be
one in a million chance of an electron
flowing becomes one in one six orders of
magnitude difference the voltage to
create that electric field that causes
the change is where a lot of the power
in modern chips comes from and the key
limit to Modern chip designs which
hasn't changed in over 30 plus years
that limit is given at 60 molts per
order of magnitude also known as 60
molts per decade in order to transition
those six orders of magn itude it
requires around 360 MTS and this is
above and beyond the minimum voltage
required to get the chip to even
function the reason this exists is due
to the fundamental premise of the modern
transistor modern transistors are also
known as mosfets metal oxide
semiconductor Field Effect transistors I
mentioned that we tried to get electrons
to flow between two locations known as a
source and a drain the voltage is
applied through an oxide layer known as
a gate in order to build the transistor
in the device the source and the gate
have silicon atoms removed or replaced
in a process called doping you can dope
with elements like Boron or phosphorus
to adjust the structure of the Silicon
making its electron rich or electron
deficient in a mosfet both source and
drain are doped the same way and then by
applying a voltage to this gate
separated by this oxide it creates a
field that attracts electrons from
source to drain this is a design that
has a 60 molt per decade
limit one way to represent this electron
transport is an energy diagram in this
diagram we have the standard transistor
and the lines indicate the energy level
of the electrons the energy level is
more of a spectrum than a line and in
order to move from source to gate you
have to give the electrons more energy
or reduce the energy requirement to move
between them through what we call the
channel in the case of a mosfet we
reduce the energy barrier of the channel
the new research published in nature by
the research group at UC Santa Barbara
has found a way to make the transistor
easier to turn on and off lowering the
power requirements it involves a t fet
or tunneling Field Effect transistor
which isn't a new technology we'll get
to that in a second but the materials
used are new in order to help drive down
the power in a tunneling fet we build a
transistor in a similar way a source a
channel a drain and then a control gate
with an oxide except this time instead
of doping the source and drain the same
way they are doped with opposite
polarity this means one side of the
transistor is electron rich and the
other side is electron deficient it
means that the transistor is predisposed
to wanting these electrons to flow
however the barrier between them is
either physical with no path or too far
for Quantum tunneling to actually take
place as perhaps name implies tunneling
fets work by applying a vol to the gate
that causes electrons to tunnel from one
end of the transistor to the other this
is a Quantum effect there's no physical
path but we have electrons ready to move
from one side to the
other in order for the electrons to move
we have to talk about the electron
energies again this is the diagram
before where we showed with standard
transistors with that the energy hump to
get over in order for the electrons to
flow due to the way tunneling fets are
manufactured the energy diagram actually
looks like this which might be a little
confusing at first but for those
familiar with the technology terminology
this is a representation of electron
bonding and antibonding orbital energies
also known as the electron bands in
order for tunneling to occur the
electron energies need to overlap such
that the electron can transfer from
source to drain while staying at the
same energy level and conserving energy
when the voltage is applied to the
tunneling fet the energy levels for the
electrons in the source and drain move
from not overlapping to overlapping
enabling this Quantum effect the energy
required to do this is much less than a
standard transistor again we previously
mentioned this 60 molts per decade
number yet in this research paper with
the 2D Channel material used the group
were able to represent it at 18 molts
per decade a 70% decrease reducing the
active voltage for modern processors by
70% would be a sizable Improvement it
anything from Big AI chips down to
smartphones and smart devices let's
cycle back and talk about that channel
material I mentioned it's a 2d material
but what is a 2d material if you take
standard table salt known as sodium
chloride it naturally forms a large
cubic array of sodium ions and chloride
ions the elements used naturally form a
large array almost every combination of
elements outside of organic materials
form large arrays however there are a
few that end up limited with which
directions they can spread 2D materials
fall into this Gap they can scale in an
X and a y direction but due to the
nature of the elements used do not scale
in The Zed Direction one group of two
dimensional materials are called tmds
which is an acronym for transition metal
dialogen the name isn't important but
these are materials you may have heard
me mentioned before in other videos
malum disulfide or mos2 is one and
tungsten diell oride wse2 is another
these both form 2D materials that kind
of look like this it's a sheet and yes
if you know anything about Nano sheets
this is where Nano sheets is going to
end up as well however in this research
paper 2D materials were used for the
channel as well as the source to enable
this 80 Ms per decade performance it was
compared to equivalent 7 nanometer
finfet devices and provided some
surprising results
firstly any device this much in research
isn't going to be ready for prime time
anytime soon regular transistors have
had 50 years of development these new
transistors have not so at an equivalent
voltage where a finfet could run at 1
GHz this tunnel fet would run at 0.7
mahz that's over a thousand times slower
however at that voltage the static power
of a billion transistors is only 0.22
microwatt compared to 1.54 watts of the
finfets that's a factor difference of
over 7 million due to these features the
research paper modeled the transistor as
part of a digital neuromorphic chip and
compared it against known models of
modern neuromorphic chips the research
groups behind this design are actually
involved in neuromorphic research hence
the focus on something super low power
and researchers from Intel's
neuromorphic team were also involved in
this research neuromorphic chip power
consumption is usually measured by an
activity Factor as in how regularly a
neuron is used or fired at low activity
the new 2D tunnel fet showcased several
thousand times lower power per neuron
fired now there are a couple of barriers
to high volume adoption here as you
might expect with any early research
technology even though the paper states
that standard manufacturing methods have
their limitations standard manufacturing
methods are used more often not because
they can scale and create millions of
chips per day the techniques to create
these type of transistors are
specialized especially when using 2D
materials such as the malum disulfide or
tungsten D teleride lots of research is
going on today to even manufacture those
on standard 300 mm Wafers for use with
standard cosos manufacturing methods
another limitation is cost low volume
parts will be higher uh higher cost to
manufacturer and one of the benefits of
the current generation of mass
production technology is that it has had
50 to 70 years of optimization put into
it one of the things I love about what I
do is the ability to look at what's new
what's the latest and greatest it's why
I spent a lot of time talking about the
latest generation of chips for CPUs for
AI or specialist compute it's great that
we can build on the foundational
technologies that we have got to where
we are today however if we can find New
Foundations with less restrictive limits
to help the future evolve faster and the
better and good for all then it's worth
keeping an eye out for potential roots
for better Hardware in my discussions
with legendary chip architect Jim Keller
he is always an advocate for rebuilding
the foundations of an architecture as
often as possible design something good
then get the low hanging fruit in the
Next Generation then start again with a
fresh sheet of paper it allows you to
reset your Baseline time and time again
in land of transistors we're doing that
with PL of transistors to finfets
finfets to Nano ribbons or gate all
around and then in the future we have
Fork sheets and stacked transistors on
that road map as well but fundamentally
changing our physics perhaps we need to
do that sometime soon
[Music]
[Laughter]
[Music]
Browse More Related Video
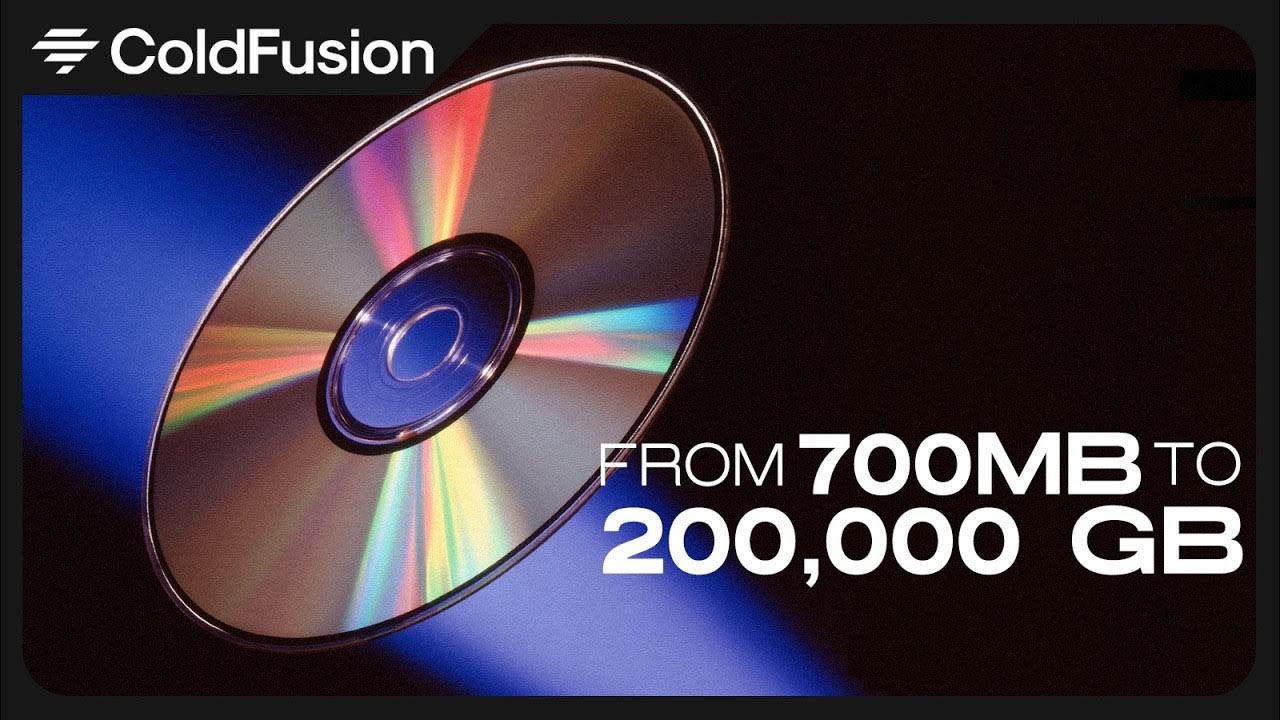
Why This New CD Could Change Storage

Is This Accidental Discovery The Future Of Energy?
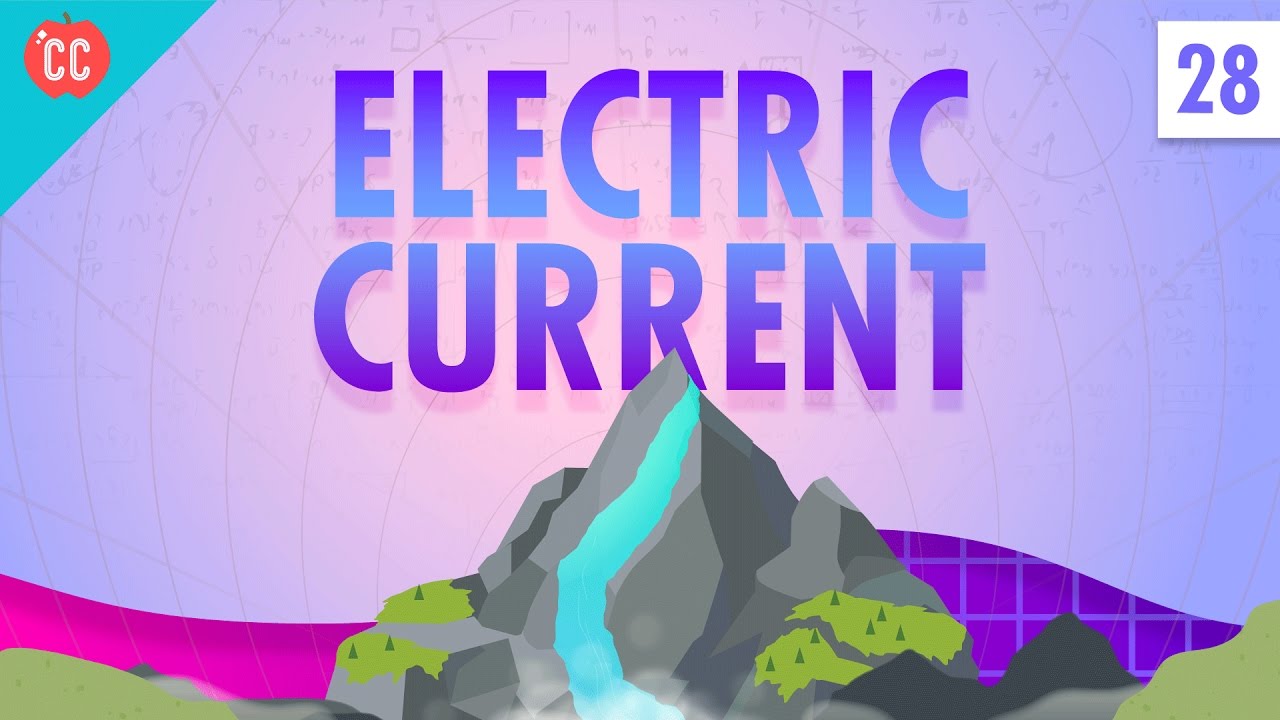
Electric Current: Crash Course Physics #28
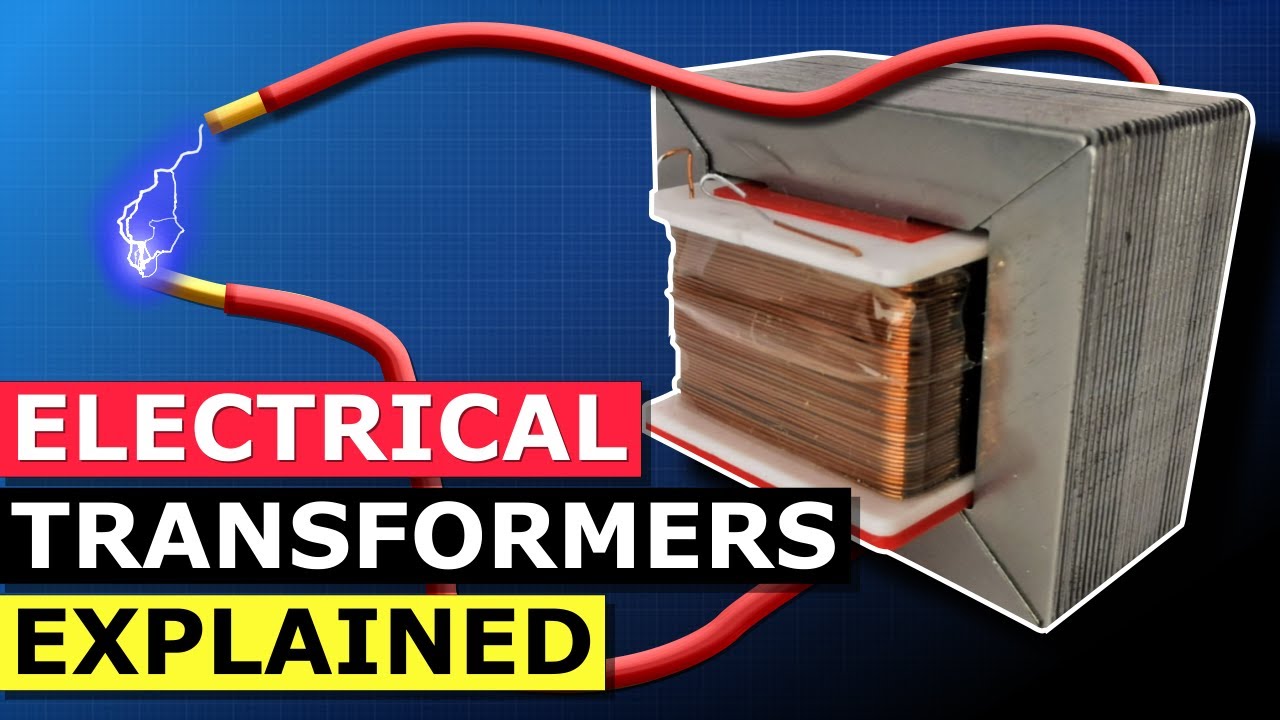
Transformers Explained - How transformers work
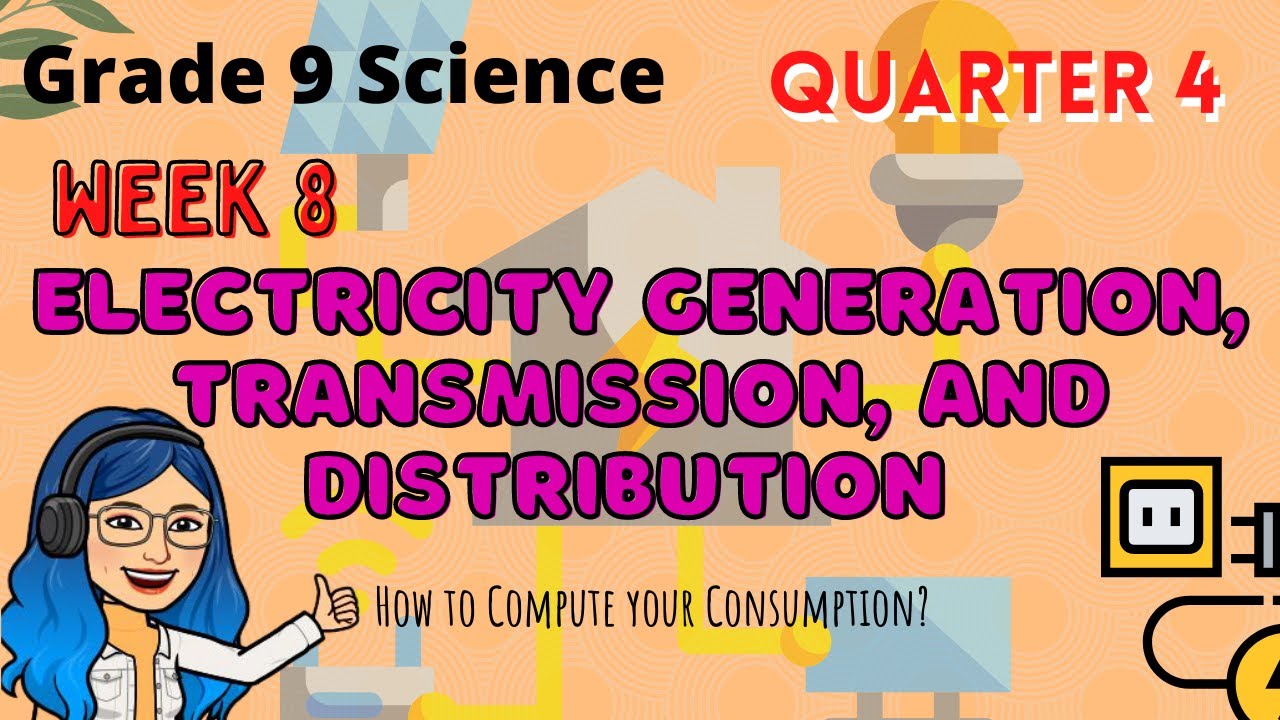
Electricity Generation, Transmission, and Distribution | Grade 9 Science Quarter 4 Week 8
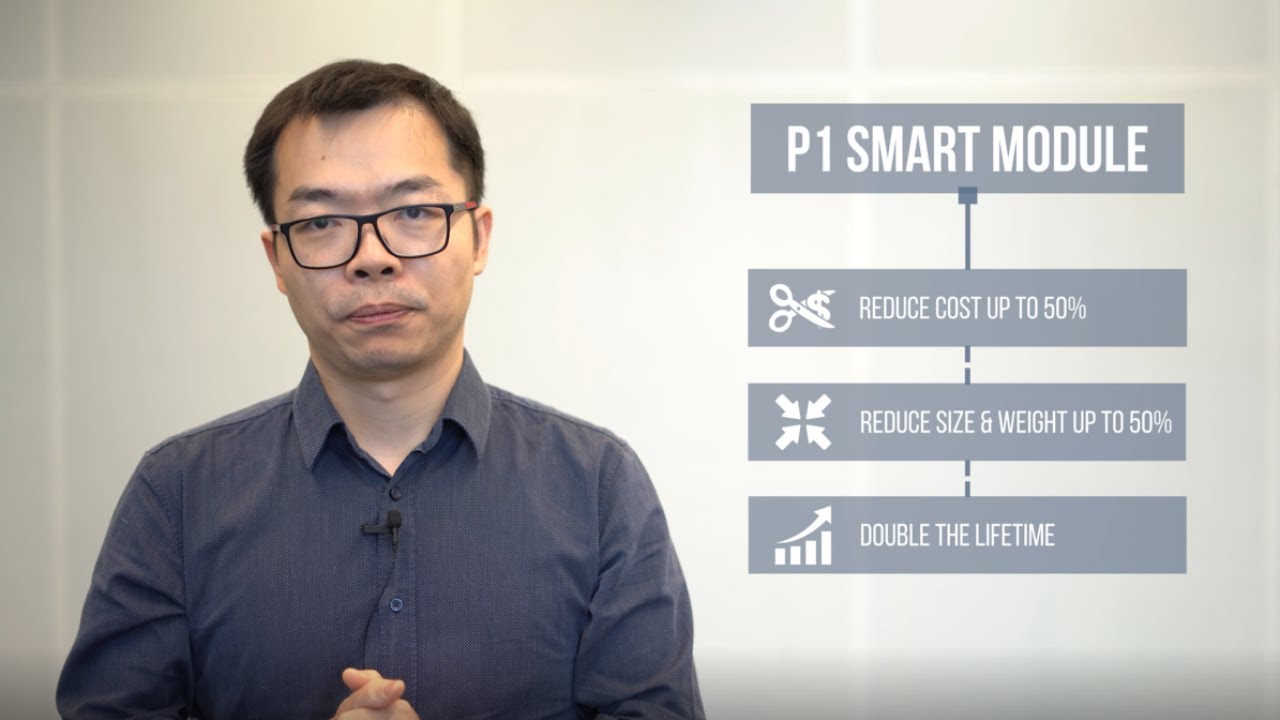
Nordic Passive: Two-Terminal Active Capacitor
5.0 / 5 (0 votes)