Introduction to Semiconductor Physics and Devices
Summary
TLDRThis video offers an insightful overview of semiconductor physics, focusing on the core questions for electrical engineers: the number of charge carriers in a semiconductor, their movement in response to an electric field, and how to manipulate these properties for practical applications. Starting with quantum mechanics and statistical mechanics, the video will delve into concepts such as density of states, energy-momentum relations, effective mass, and Fermi statistics to understand charge carrier behavior. It will also explore carrier movement mechanisms like drift, diffusion, and recombination, leading to the analysis of devices like MOSFETs, diodes, BJTs, and optical devices, bridging the gap between fundamental physics and real-world engineering.
Takeaways
- 🌟 Semiconductor physics is central to understanding the operation of electrical devices like MOSFETs, diodes, and BJTs.
- 🔍 The fundamental questions in semiconductor physics include understanding the number of charge carriers, their location, movement, and how to manipulate them for practical applications.
- 📚 The script emphasizes the importance of quantum mechanics and statistical mechanics as the foundational tools for analyzing semiconductors.
- 📊 The concept of density of states is introduced, which is crucial for understanding how many states are available for electrons within a semiconductor.
- 🚀 The energy-momentum relationship and band diagrams are key for visualizing and analyzing the behavior of charge carriers in semiconductors.
- 🧠 The effective mass concept is highlighted, which helps relate the motion of charges in free space to how they move within a semiconductor like silicon.
- 📉 Fermi statistics are tied to the density of states and are used to determine the number of charge carriers in a semiconductor.
- 🔄 The script discusses carrier movement mechanisms such as drift and diffusion, which are essential for understanding how carriers respond to external electric fields.
- 💡 The generation and recombination of carriers are also covered, indicating that carriers are not static but are in a constant state of creation and annihilation.
- ⚡ Ohm's law is derived from understanding carrier drift, illustrating the connection between semiconductor physics and circuit theory.
- 🛠 The continuity equation and ambipolar transport equation are introduced as powerful tools for solving various semiconductor problems, including the analysis of PN junctions and the operation of diodes, MOSFETs, and BJTs.
- 🌞 Optical devices like solar cells, photodiodes, and LEDs are also within the scope of semiconductor physics, with their operation explained through the principles discussed.
Q & A
What is the central question in semiconductor physics regarding charge carriers?
-The central question in semiconductor physics regarding charge carriers is how many charge carriers, such as electrons, are available to conduct current within a semiconductor material like silicon.
Why is the number of charge carriers in a semiconductor not equal to the number of atoms?
-In semiconductors, the number of charge carriers is not equal to the number of atoms because not all atoms contribute free electrons to conduct current, unlike in metals where each atom typically contributes one electron.
What are the two main questions in semiconductor physics that the video aims to address?
-The two main questions are: 1) How many charge carriers do I have in a semiconductor? 2) Where are they, and how are they moving in response to an external electric field?
What is the significance of understanding the movement of charge carriers in semiconductors?
-Understanding the movement of charge carriers is crucial for predicting how the charge concentration changes within the semiconductor under the influence of an external electric field, which is essential for designing and analyzing semiconductor devices.
Why are engineers interested in semiconductor physics?
-Engineers are interested in semiconductor physics because it provides the foundation for analyzing and creating practical applications of various semiconductor devices, such as MOSFETs, diodes, and BJTs.
What is the starting point for analyzing semiconductors?
-The starting point for analyzing semiconductors is quantum mechanics, along with statistical mechanics, which are fundamental tools for understanding the behavior of electrons in these materials.
What is a band diagram and why is it important in semiconductor physics?
-A band diagram is a graphical representation that shows the distribution of energy levels in a semiconductor. It is important because it helps in understanding the behavior of electrons and holes within the material, which is crucial for semiconductor device design.
What is the effective mass of a charge carrier in a semiconductor?
-The effective mass of a charge carrier in a semiconductor is a measure of how the carrier's motion is influenced by an electric field within the material, as opposed to in free space. It helps relate the motion of charges in semiconductors to their behavior in free space.
What is Fermi statistics and how does it relate to semiconductor physics?
-Fermi statistics is a set of principles that describe the distribution of particles over energy states in a system at thermal equilibrium. In semiconductor physics, it is closely tied to the density of states and is used to determine the number of available charge carriers.
What are the main mechanisms for carrier movement in semiconductors?
-The main mechanisms for carrier movement in semiconductors are drift, which is the movement of carriers due to an external electric field, and diffusion, which is the movement due to concentration gradients.
How does understanding carrier drift lead to Ohm's law?
-Understanding carrier drift in semiconductors leads to Ohm's law because it explains the relationship between current and voltage in a material, showing how the flow of charge carriers (current) is proportional to the electric field applied (voltage).
What are the continuity equation and ambipolar transport equation used for in semiconductor physics?
-The continuity equation and ambipolar transport equation are used to describe the conservation of charge and the movement of both electrons and holes in semiconductors. They are essential tools for analyzing various semiconductor problems, including the behavior of PN junctions and transistors.
Outlines
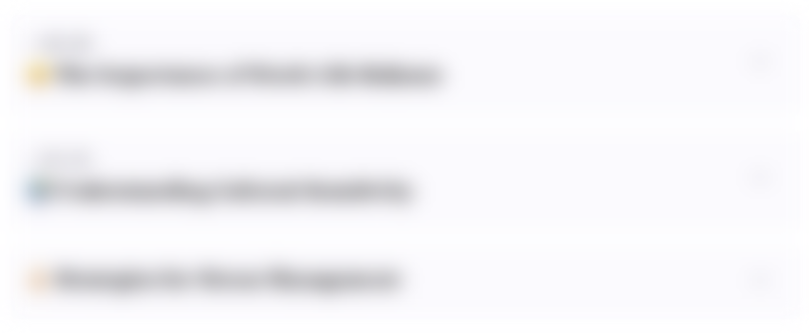
This section is available to paid users only. Please upgrade to access this part.
Upgrade NowMindmap
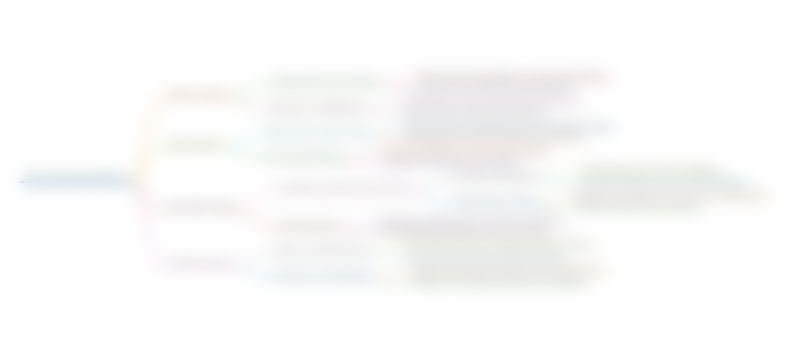
This section is available to paid users only. Please upgrade to access this part.
Upgrade NowKeywords
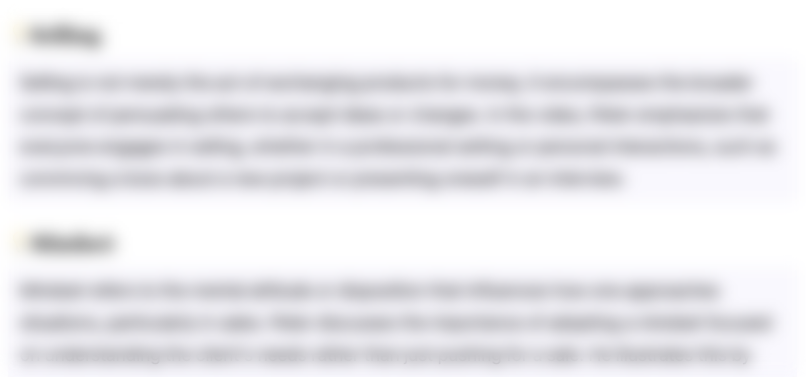
This section is available to paid users only. Please upgrade to access this part.
Upgrade NowHighlights
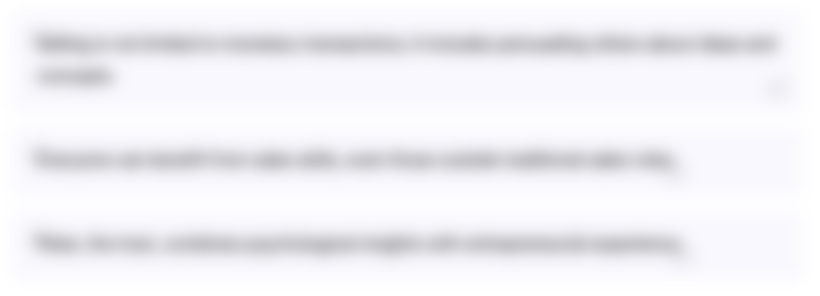
This section is available to paid users only. Please upgrade to access this part.
Upgrade NowTranscripts
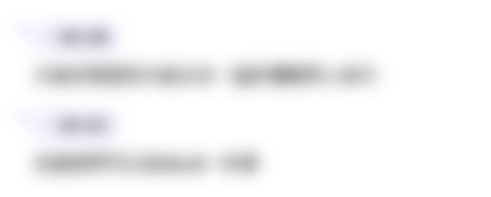
This section is available to paid users only. Please upgrade to access this part.
Upgrade NowBrowse More Related Video
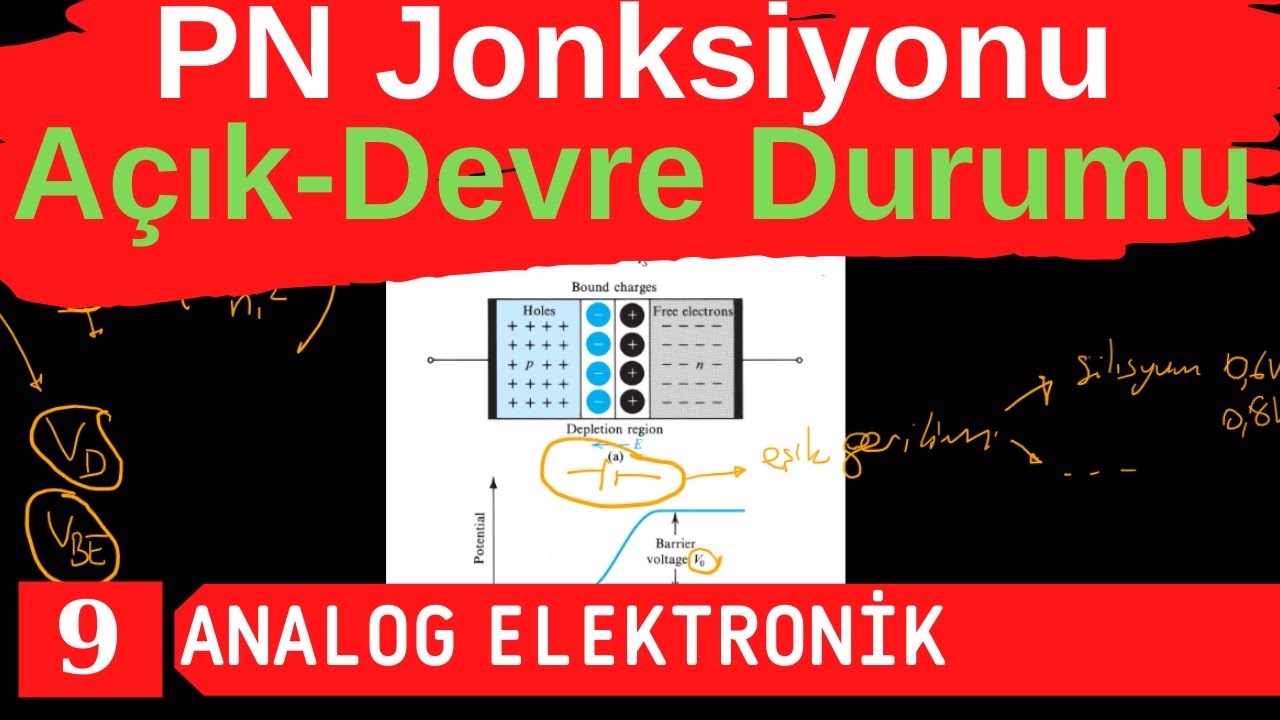
Elektronik-1 : Yarıiletken | PN Jonksiyonu-Açık Devre Durumu
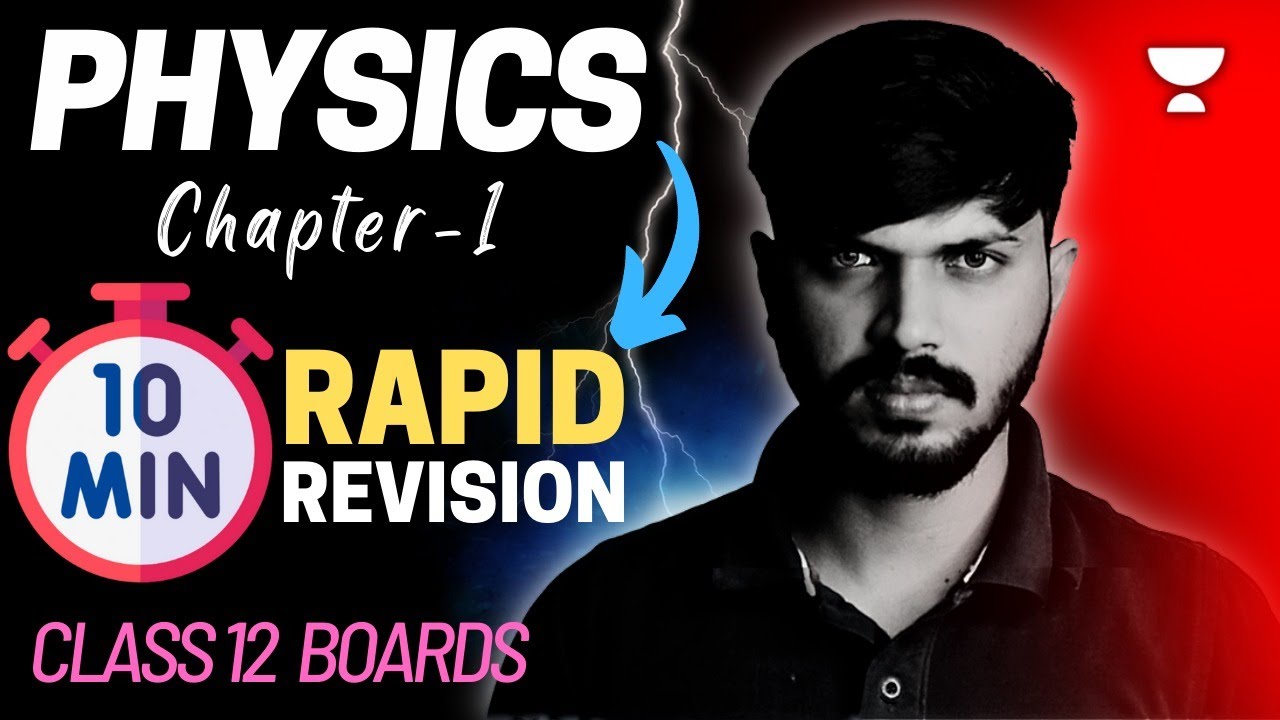
Electric Charges & Fields in 10 mins 😱🔥 Ch 1 Physics Class 12 Boards 2024 Score 95+ Zaki Bhaiya
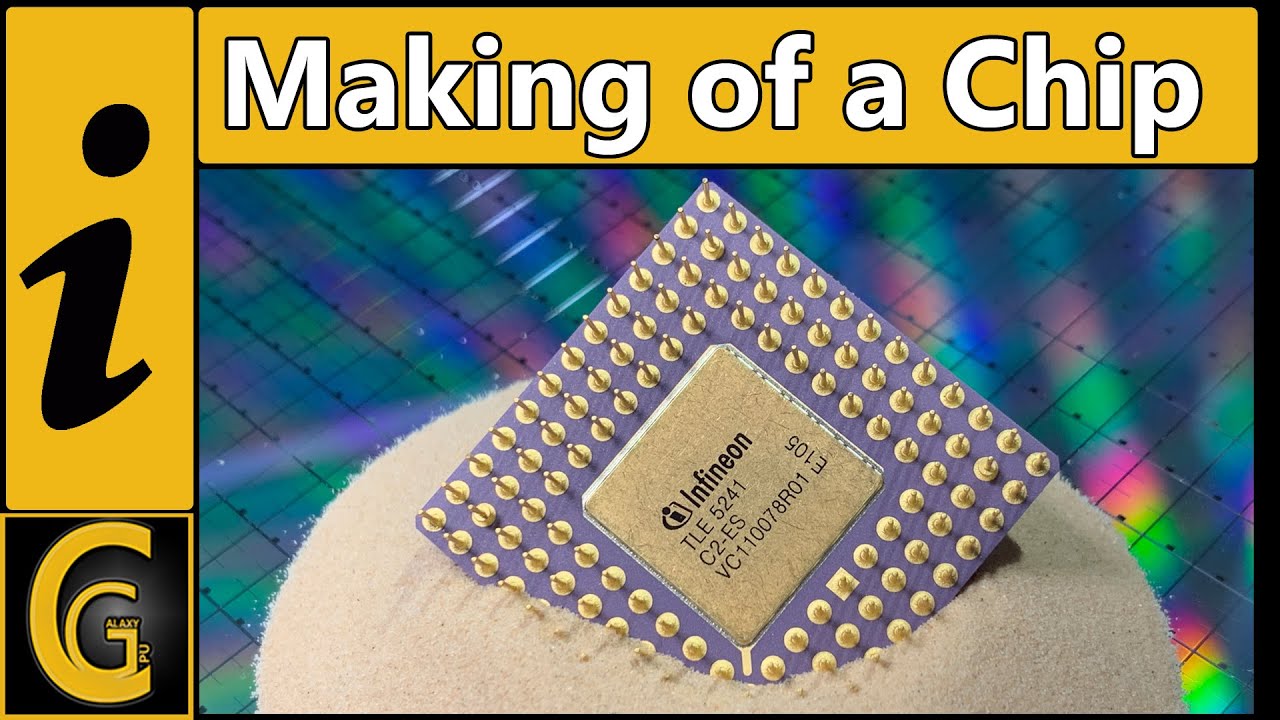
How Microchips Are Made - Manufacturing of a Semiconductor
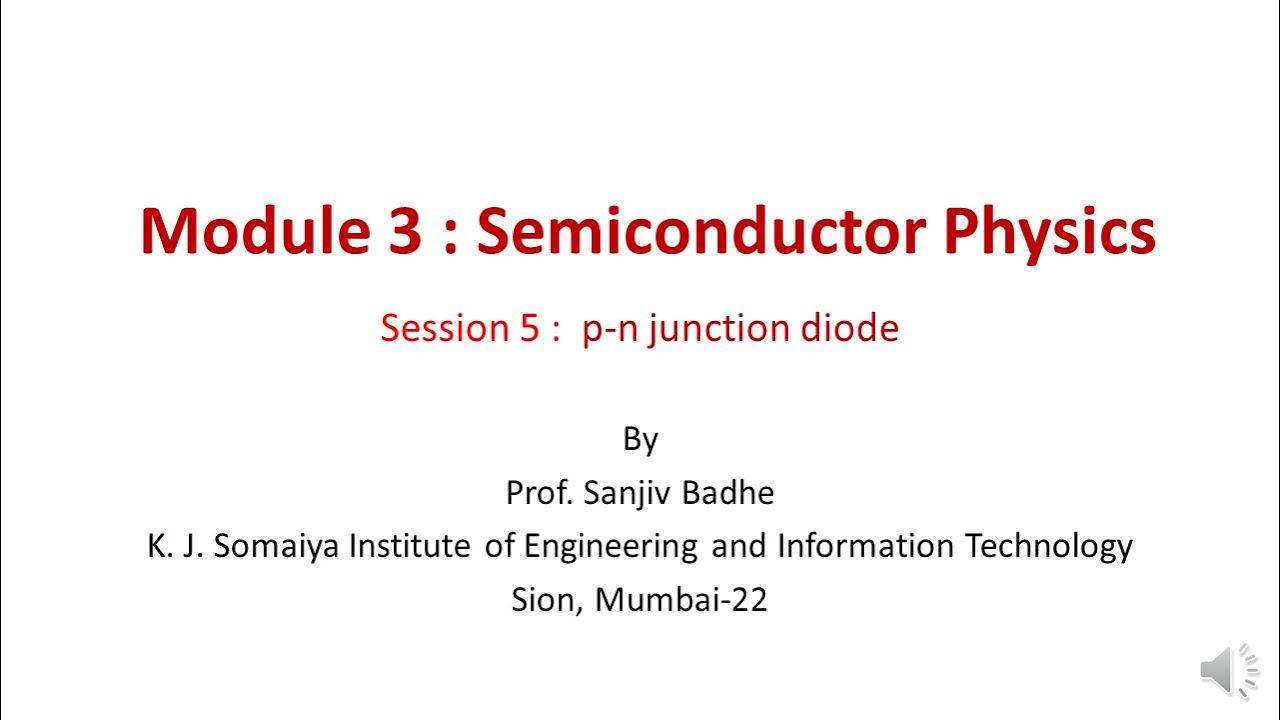
Semiconductor Physics Session 5 (p-n junction diode)
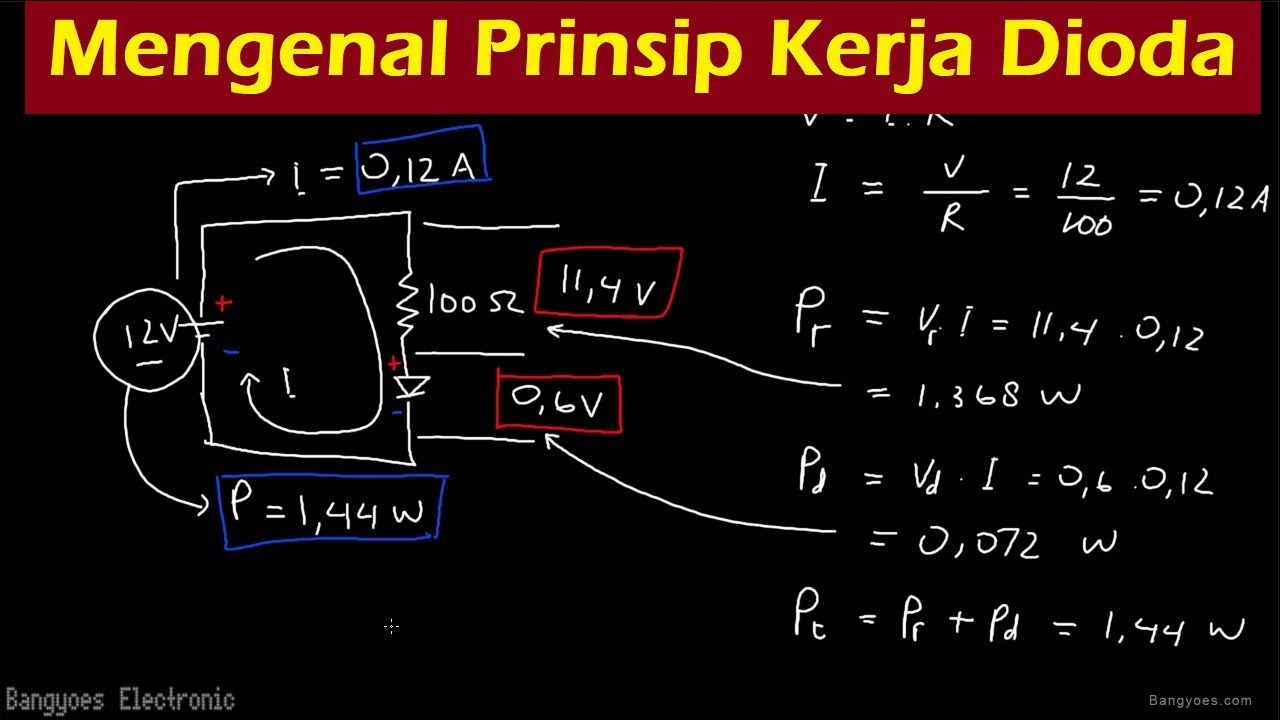
Elektronika Dasar - Mengenal Prinsip Kerja Dioda dalam Rangkaian Listrik

MODERN PHYSICS MIMP QUESTIONS FOR GTU EXAM || SEM 1 PHYSICS || UNIT 5 | SEMICONDUCTOR DEVICE #gtu
5.0 / 5 (0 votes)