Waves, Light and Sound - Physics 101 / AP Physics 1 Review with Dianna Cowern
Summary
TLDRIn this physics lesson, Dianna Cowern explores the nature of waves, demonstrating how they carry energy and information through materials like a Slinky. She clarifies misconceptions about waves, distinguishing between transverse and longitudinal types, and explains how waves like light and sound interact with matter, affecting phenomena such as refraction, the Doppler effect, and the universe's expansion. The lesson includes practical examples and equations, illustrating the fundamental wave equation v = fΞ», and emphasizes the importance of understanding waves in various contexts, from everyday experiences to cosmological studies.
Takeaways
- π Waves are disturbances or displacements in a medium that carry energy without the medium itself moving in the direction of the wave's energy transfer.
- π Longitudinal waves involve the medium moving parallel to the direction of energy transfer, like sound waves in air.
- π Transverse waves occur when the medium moves perpendicular to the direction of energy transfer, such as light waves through the electromagnetic field.
- π The speed of a wave is determined by the properties of the medium and is independent of the wave's amplitude or how hard the wave is pushed.
- π’ The wave equation ( v = Ξ» Γ f ) relates the velocity (speed) of a wave to its wavelength ( Ξ» ) and frequency ( f ) in a given medium.
- πΆ The frequency of a wave is linked to the pitch of a sound, with higher frequencies corresponding to higher pitches.
- π Wavelength is a key factor in determining the color of light, with shorter wavelengths corresponding to bluer colors and longer wavelengths to redder colors.
- π The speed of light in a vacuum is constant ( c = 299,792,458 m/s ), but it slows down when it enters a medium like water or glass, causing refraction.
- π¨ The Doppler effect causes a change in the frequency (and perceived pitch) of waves when the source is moving relative to the observer, resulting in a higher pitch as it approaches and a lower pitch as it recedes.
- π The redshift observed in galaxies indicates that they are moving away from us, a phenomenon that led to the understanding of the universe's expansion.
- π Studying physics involves peeling back layers of understanding to reveal deeper insights into the world, with each discovery leading to more questions and a greater appreciation for the complexity of the universe.
Q & A
What is the primary way the wave moves down the Slinky in the video?
-The primary way the wave moves down the Slinky is through a longitudinal wave, where the individual bits of the medium move back and forth along the direction that the energy travels.
Why does pushing harder on the Slinky not change the speed of the wave?
-Pushing harder on the Slinky does not change the speed of the wave because the speed of the wave is determined by the properties of the medium and its tension, not the force applied to create the wave.
How does stretching the Slinky affect the speed of the wave?
-Stretching the Slinky puts it under more tension, effectively changing the medium and allowing the wave to travel faster due to the increased tension in the medium.
What is the difference between longitudinal and transverse waves?
-In longitudinal waves, the particles of the medium move back and forth in the same direction as the wave's energy travels, like sound waves in air. In transverse waves, the particles move perpendicular to the direction of energy travel, like light waves disturbing the electromagnetic field.
How does the Slinky demonstration relate to the concept of waves in the ocean?
-The Slinky demonstration relates to ocean waves by illustrating that it's not the medium (water in the ocean, Slinky material) that travels but rather the disturbance or energy transfer that moves through the medium.
What is the definition of a wave given in the script?
-A wave is defined as a disturbance or displacement of some medium that carries energy.
How does the amplitude of a wave relate to the energy it carries?
-The amplitude of a wave is related to the energy it carries; the greater the amplitude, the more energy the wave carries. However, the script focuses more on frequency and wavelength as indicators of energy in the context of light and sound.
What is the relationship between wavelength, frequency, and the speed of a wave?
-The relationship between wavelength, frequency, and the speed of a wave is given by the wave equation: velocity (v) equals wavelength (Lambda) times frequency (f), or v = Lambda * f.
Why do some waves, like water waves, exhibit both longitudinal and transverse characteristics?
-Some waves, like water waves, exhibit both longitudinal and transverse characteristics because the particles of the medium move in a combination of back-and-forth and up-and-down motions, resulting in a circular motion that incorporates elements of both wave types.
How does the speed of light change when it travels through different media?
-The speed of light remains constant in a vacuum but slows down when it travels through any material medium, such as glass or water, due to the interaction with the particles in the medium.
What is the Doppler effect, and how does it relate to the pitch of sound?
-The Doppler effect is the change in frequency and wavelength of a wave in relation to an observer who is moving relative to the wave source. When the source moves toward the observer, the waves are compressed, leading to a higher pitch. Conversely, when the source moves away, the waves are stretched, resulting in a lower pitch.
How does the stretching of the Slinky loops in the demonstration relate to the concept of the universe's expansion?
-Stretching the Slinky loops changes the medium and affects the speed of the wave traveling through it, similar to how the expansion of the universe affects the speed and properties of light waves from distant galaxies, causing a redshift or blueshift based on their movement toward or away from us.
Outlines
π Understanding Waves with a Slinky
The video begins with an exploration of waves using a Slinky as a visual aid. The presenter, Dianna, demonstrates how pushing the Slinky creates a wave and discusses the misconception that pushing harder makes the wave move faster. Instead, she shows that stretching the Slinky changes the medium and affects the wave's speed. Dianna introduces the concept of waves as disturbances that carry energy without the medium itself moving, using the Slinky, ocean waves, and ripples in water as examples. She emphasizes that waves are about energy transfer and not the physical movement of the medium.
π Waves, Light, and Sound: The Basics
This paragraph delves into the relationship between waves, light, and sound. Dianna explains the nature of waves, distinguishing between transverse and longitudinal waves. She uses the Slinky to illustrate both types, with longitudinal waves being similar to sound waves traveling through air. The paragraph also touches on the mathematical representation of light waves and their role in the electromagnetic field. Dianna highlights the importance of wave properties such as period, frequency, wavelength, and amplitude, which are essential for understanding how waves carry energy and information.
π The Spectrum of Light and Color
The focus shifts to light waves, discussing the visible spectrum and how different colors correspond to specific wavelengths. Dianna explains that shorter wavelengths, such as those of blue light, carry more energy than longer wavelengths, like red light. She also mentions ultraviolet light, which carries even more energy and can be seen by some animals but not by humans. The paragraph explores how the wavelength of light is connected to its color and energy, and how different wavelengths can be detected with specialized equipment to study celestial objects.
π The Speed of Waves and the Doppler Effect
This section examines the speed at which waves travel and how it is influenced by the medium. Dianna uses the example of a stretched Slinky to show that altering the medium can change wave speed. She then discusses the constancy of the speed of light in a vacuum and how it slows down in different media, leading to phenomena like refraction. The paragraph also introduces the Doppler effect, which describes the change in frequency and pitch of waves due to the relative motion between the source and the observer, with examples from sound waves and light waves.
π The Cosmic Implications of Wave Properties
The final paragraph ties the concepts of waves to the broader context of the universe. Dianna explains how the Doppler shift in light from distant galaxies revealed that the universe is expanding. She discusses the redshift observed in galaxies moving away from us and the implications for our understanding of the cosmos. The paragraph concludes with a guest message from Destin Sandlin, encouraging viewers to delve deeper into physics to uncover more about the universe's mysteries.
Mindmap
Keywords
π‘Slinky
π‘Wave
π‘Longitudinal wave
π‘Transverse wave
π‘Frequency
π‘Wavelength
π‘Amplitude
π‘Wave speed
π‘Doppler effect
π‘Refraction
π‘Dark energy
Highlights
Demonstration of wave speed in a Slinky and the misconception that pushing harder makes the wave move faster.
Explanation of changing the medium to alter wave speed, shown by stretching the Slinky.
Introduction to Dianna Cowern's Physics by Dianna series and its relevance to AP Physics 1 curriculum.
Definition of a wave as a disturbance or displacement of a medium that carries energy.
Illustration of the difference between longitudinal and transverse waves using a Slinky.
Discussion on how sound waves travel as longitudinal waves through the air.
Clarification that light travels as a transverse wave disturbing the electromagnetic field.
Introduction of the mathematical representation of light waves.
Explanation of how water waves combine both longitudinal and transverse wave properties.
Importance of wave properties such as period, frequency, wavelength, and amplitude in physics.
Relevance of frequency to the pitch of a musical note and its measurement in Hertz.
Description of the relationship between wavelength, frequency, and the speed of light.
Practical application of wave properties to calculate the size of radio waves based on frequency.
Influence of medium properties on the speed of sound and its dependence on temperature and molecular mass.
Demonstration of the effect of different gases on the pitch of sound using helium and sulfur hexafluoride.
Explanation of how stretching the Slinky changes its medium properties and affects wave speed.
Discussion on the constancy of the speed of light in a vacuum and its variation in different media.
Introduction to the concept of refraction and its everyday examples like a spoon in water.
Explanation of the Doppler effect and its impact on the perceived pitch of sound from moving sources.
Cosmological significance of redshift and blueshift in understanding the expansion of the universe.
Encouragement from Destin Sandlin to delve deeper into physics for a richer understanding of the world.
Transcripts
I've got here a Slinky.
And I'm going to send a wave down the length of the Slinky
by pushing it inwards, like that.
And now I have a question for you.
How can I make that wave move faster down the Slinky?
I'm going to intuit that a lot of you
probably guessed, you push harder.
So let's check it out.
No.
[GRUNT]
No matter how hard I push, the wave still moves the same speed
down the length of Slinky.
So what else could I do?
Well, I could change the medium.
I could change the Slinky by stretching it out even more.
I've got more on the either end, so it's a little more stretched out.
Check it out now.
You can't even see how fast the wave moves down the end.
[GRUNT] It's like immediate.
[GRUNT]
So hopefully by the end of this lesson,
we're going to understand why that works.
Hey, I'm Dianna Cowern.
And welcome to lesson 17 of Physics by Dianna.
I usually call this Dianna's Intro Physics class
because it's useful if you're looking
for an overview of intro-level physics topics
from kinematics to energy to electricity.
But this series is also useful if you're
looking for a review of the AP Physics 1 curriculum,
which is what we follow.
OK, so today's lesson--
today is about waves and light and sound,
which are all related things.
If you're seeing or hearing this video,
it's because light and sound waves
are reaching you from your screen or from your speakers.
So waves can form in and travel through materials
like water, which is the reason that surfing exists.
So thank you, waves.
So our theme today is catching waves.
[WAVES SPLASHING]
I couldn't fit my whole surfboard
in the studio, so just dress-up.
So what is a wave?
I'm going to talk about some misconceptions about waves
and about what waves are.
So let's use this Slinky again.
So if I shoot a pulse down the length of the Slinky
like I did before, that's a type of wave.
Notice that the Slinky itself doesn't travel in the direction
that the energy does.
This...is not a wave.
For a wave to happen, the Slinky bunches up and then
that bunching moves down.
But it returns to its original state.
So even though it looks like something is moving down
the length of the slinky, no thing actually is moving.
It's just a disturbance traveling down.
Same with waves in the ocean.
They keep coming at you.
They keep crashing on shore.
But it's not the water continually coming at you
or you would get continually rising seas.
So unless a tide is happening, the water
is not moving up at you.
It's just this disturbance in the water moving forward.
If you do a cannonball into a pool
or throw a rock into a lake, then when the ripple forms,
energy is moving through the water
in the form of a disturbance.
But if you look closely at the water itself,
you see every part of the water moves
up and down or maybe with some circular motion,
but it doesn't travel outward like the ripple does.
It is energy that's moving outward
and this disturbance transmitted down the wave.
Now we can easily define what a wave is.
It's a disturbance or a displacement of some medium
that carries energy.
That's a wave.
Here's a crazy type of wave, a gravitational wave.
We've talked about them before.
But it's the same concept we just discussed.
Some disturbance happens, like two black holes collide,
and that triggers the stretching
and compressing of space-time itself.
It's like the ultimate medium for waves to go through.
Then, of course, it definitely carries a lot of energy.
If you took all of the mass of three suns
and turned it all into energy,
that's how much energy some of these collisions
have released.
So crazy.
Lucky for us though, that energy is not concentrated
right on us; it spreads out.
And typically the collisions happen very far away.
Now there's a couple of different ways
I could have sent a wave down the Slinky
even though it's just a simple piece of plastic.
The way that I just did it was by pushing along the Slinky.
But I could have also moved it side to side.
See how those are different?
So the first one is called a longitudinal wave
where the individual bits of the medium
move back and forth along the direction
that the energy travels.
So that's a longitudinal wave.
That's how sound waves travel through air.
So when I yell "Stella"--
so when I do that, I'm compressing the air.
And then parts compress and parts stretch out,
and that compression moves through the air
toward your ear.
But if you look closely at the individual particles,
they're just moving back and forth.
Now, the other way of making a wave--
that up-and-down motion--
that's called a transverse wave.
I like to remember it--
longitudinal is along the direction and transverse
is the other one.
In the transverse case, the medium
moves perpendicular to the direction that energy travels.
Interestingly, that's how light works.
Light travels as a transverse wave disturbing
the electromagnetic field.
Yeah, we're going to get to that weird concept
a little more in the next lesson.
But here's a mathematical representation
of light waves disturbing the electromagnetic field.
So the wave--that's a transverse wave.
You could make of a longitudinal wave,
but everyone would have to go like this.
Fun fact, some waves are actually a combination
of longitudinal and transverse.
The water wave animation that we saw before
is just like that--a little bit of back and forth,
a little up and down.
And the result is a circular motion.
OK, waves--but what can we, as physicists, do with them?
Well, obviously we measure things,
like measuring the energy carried by a wave.
Let me tell you, it's not easy to just look at a wave
and know how much energy it's carrying.
But there are other properties of waves
that we can more easily measure and use those
to figure out the energy.
And each of those properties is important in its own way,
too, as I'll explain.
Consider a transverse wave, like a Slinky, oscillating side
to side, like this.
I'm going to draw that.
So what I'm drawing is a little snapshot of the wave in time.
So the wave has some properties that are
familiar from previous videos.
As a part of the Slinky moves side to side,
it takes some amount of time to return to its starting point.
So that would be like the wave going from here
to here to here.
And that time it takes is the period of the wave.
Time is the period.
The period is something that surfers care about
because it tells you how often the waves are going to come.
If we measure how many oscillations happen per second,
like the frequency of the waves, that's called the frequency.
And just like when we talked about angular motion
and simple harmonic motion, the frequency
is inversely proportional to the period.
So t equals 1 over f.
And we can work out the units of this too.
So the units of period are obviously
a unit of time, seconds.
And that's 1 over units of frequency or Hertz.
Hertz is actually 1 over second.
So if you flip that, you get seconds back.
Musicians or audio people very much care about frequency,
because for sound waves, the frequency
is what determines the pitch of a note.
The higher the frequency, the higher the note.
[WHISTLE]
Check it out.
I've got this app that shows you sound waves like--
[WHISTLING]
Now actually, it may be a little easier
to see what I'm talking about if you
know the next property called wavelength.
That's the distance between two peaks of the wave
or two troughs or two midpoints--
not these two midpoints-- it's like a full wavelength,
basically the distance
between two identical points on the wave.
Lower notes have longer wavelengths.
[WHISTLING]
And higher notes have shorter wavelengths.
[WHISTLING]
Physicists use the symbol Lambda for a wavelength.
There's one more useful property waves that we're not really
going to use today, but you might as well know.
It's called the amplitude.
And it's basically the midpoint.
It's like where the Slinky would be if it were resting,
all the way up to the top, or up to the bottom--same distance.
So this distance and this distance,
those are both amplitude.
Wavelength is also super important
because it's how physicists describe colors of light.
For example, the wavelength of blue light
is around 470 nanometers, which is shorter
than red light, which ranges around 750 nanometers.
And then beyond that, on both ends of the visible spectrum--
well, we don't talk about that. Just kidding.
We're going to get to that in the next lesson.
But I'll give you a hint.
Even though we can't see some wavelengths of light
with our eyes, astrophysicists use
different kinds of telescopes and detectors
to be able to collect light of all different sorts
of wavelengths so we can learn extra things about stars
and galaxies that we otherwise wouldn't be able to see.
And on the topic of wavelengths, with our Slinky,
we can see that if we oscillate this Slinky
with quick little waves, or like this,
we're sending more energy down with the wave
than if you also with longer waves.
Obviously, more energy.
And that's true for light as well.
Light with shorter wavelengths carries more energy
than light with longer wavelengths.
That's why ultraviolet light,
which has really, really short wavelength from the sun,
can damage your skin, because it carries even more energy
than blue light or than any of the other visible colors
of light.
Fun fact, humans can't see ultraviolet light,
but some other animals can.
There are butterflies, fish, even reindeer
that can see ultraviolet light.
Flowers look different in ultraviolet light
to attract pollinating insects.
So there's a specific relationship
between the frequency and the wavelength of a wave.
And we can figure this out with our Slinky.
So I'm going to move the Slinky in two different waves,
a long wave and a short wave, and then
measure their wavelengths and frequencies.
This one's about half a meter and about half a Hertz.
And then this one's about 0.1 meters and 2.5 Hertz.
So let's write that down.
The wavelength of my longer wave was 0.5 meters and my frequency
was 0.5 Hertz, about half a wave per second.
And then my second one was 0.1 meters--
the faster one.
And the Hertz were obviously quicker, 2.5 Hertz.
This make sense?
Longer wavelengths happen less often.
Shorter wavelengths happen more often.
Now watch what happens when I multiply the wavelength
and the frequency of these two waves.
If I multiply these two, I get 0.25 meters per second.
And if I multiply these, I also get 0.25 meters per second.
Hmm, interesting.
I got the same number.
So what this number is is the speed
of the wave traveling through the Slinky, or v.
So I can now write down this relationship.
Velocity of a wave through a medium
equals the wavelength times the frequency of the wave.
We call this relationship the wave equation.
And it's true for all waves.
And we can use it to figure out all sorts of things,
like how long are the waves that carry music
from your radio station to your car?
Here, we would use--
for v we would use c, because that's the speed of the wave.
The speed of the radio wave is the speed of light,
because radio waves are light.
So we would use c for the speed of light,
which is about 3.00 times 10 to the 8 meters per second.
You already know the frequency.
Maybe you didn't know you knew, but the frequency
of your radio station is just the dial number.
So mine from my favorite radio station growing up
was 93.5 on the dial.
And what that means is 93.5 megahertz.
So 93.5 times 10 to the 6 Hertz.
And so I can use this equation, 3.00 times 10 to the 8 meters
per second equals my wavelength, which I want to find out,
times my frequency, which is 93.5 times 10 to the 6 Hertz.
So my wavelength is 3.21 meters.
3.21 meters, that's huge.
That's why antennas are big.
I love to think about these huge, long light waves
traveling through the air and then getting snagged
by my car's radio antenna all so that I can rock out.
All those radio telescopes that you've ever seen,
they're massive dishes because they
have to pick up these wavelengths that are huge.
We've used these radio telescopes
to detect light waves from an outer space
in order to study pulsars and exoplanets and even search
for extraterrestrial life.
OK, let's talk about, though, the speed of waves,
because I feel like we're almost to the point of answering
the question from the very beginning.
Let's talk about the speed of sound.
The speed of sound depends on a lot of things.
And I think you've seen this demo before.
I'm going to get to it.
Sound travels through solids faster
than it does through gases, for example,
because the molecules are more tightly bound together.
So you can put your ear down and hear an earthquake
before you heard it in the air.
Can you hear earthquakes in the air?
I don't know, maybe.
The molecules are bound together more tightly
in the ground, which affects how fast particles
can hit each other and transmit the waves.
Which is why in gases--
I'm getting to the demo--
the speed of sound depends on the mass of the molecules
and the temperature of the gas.
So let's think about two different gases--
a really light one, like helium,
and a really heavy one, like sulfur hexafluoride.
My friend Mark Rober did an awesome
live-streamed physics course,
and he did this demo during the course.
[low voice] Because this is sulfur hexafluoride.
It actually does the opposite of helium.
It makes your voice low.
So we're going to discuss that.
And here it starts to wear off.
It's like still in my lungs.
Why does sulfur hexafluoride make your voice go really low,
and yet helium makes it feel really high?
What the heck is going on there?
I would someday love to try that.
But thank you, in the meantime, Mark.
You should go check out his course.
So the little helium molecules are easier to move around
than the big, heavy sulfur hexafluoride molecules.
So therefore, sound travels faster in the helium gas.
And if you look at our wave equation,
velocity equals Lambda times frequency.
A faster velocity, traveling faster
through those light molecules, v goes up,
means my frequency goes up.
Frequency goes up, pitch goes up.
That's why, when someone talks after inhaling helium
with that faster velocity, their voice becomes higher pitched
with the higher frequency.
And if you inhale sulfur hexafluoride,
then velocity goes down, frequency goes down,
your voice would sound lower pitched.
It actually doing the opposite of helium.
So the speed of sound really depends
on the type of material.
And we can use that now to explain our slinky demo.
I know it seems like the same material, the same medium.
So we're actually changing the medium
when we stretch out the Slinky loops.
We're putting it under tension and that changes the medium.
This is how sensitive waves are to the medium
that they're traveling through.
Just by stretching it out, I can change the speed of waves
inside the Slinky, very cool.
So let's go back to light waves.
You've probably heard that the speed of light is constant.
That's not quite 100% correct.
In order to be totally accurate,
we need to say the speed of light in a vacuum is constant.
In a vacuum, the speed of light is
c equals 299,792,458 meters per second.
By the way, this is also the speed of gravity.
Think about that.
So this is about 3 times 10 to the 8 meters per second.
I'm going to take a short detour and do a quick little problem.
So sound is a lot slower than light.
And now you know it would depend on what elevation
you're at, how hot it is out.
But, typically, sound travels at about 343 meters per second.
You can see now these are a lot different in magnitude.
So sometimes we talk about how you see lightning
before you hear it.
But let's talk about a rocket launch.
If you're ever lucky enough to go see one--
someday, I hope--
then say you're standing 11 kilometers away.
So that's your distance away.
Eleven kilometers, that's 11,000 meters.
So say my distance is 1.1 times 10 to the 4 meters.
I want to know how long it'll be between
when you see the ignition and you hear the rumble.
OK, so I'm going to use the equation
from our early kinematics lessons.
Velocity equals distance per time.
My distance is 11 kilometers.
And I want to know my time.
So for sound, that's going to be bring the t up,
bring the V down.
So I'm going to divide distance by velocity.
So I'm going to have my 1.10 times 10 to the 4 meters
over my velocity.
And for sound, that's 343 meters per second.
That equals 32.1 seconds.
That's a long time.
And then for my lights, same distance,
but much bigger velocity.
I'm using three significant figures.
So 3.00 times 10 to the 8 meters per second.
And I get 0.00004 seconds.
So if we subtract these out, you just get that 32.1 seconds.
So you would see the ignition half a minute earlier
than you would hear the rumble.
That is cool.
Coming back to what we were saying about the speed of light
in a vacuum.
But light actually slows down when it travels through matter.
So light traveling through Earth's atmosphere
travels a tiny bit slower than light in outer space.
And if light enters a denser medium, like glass or water,
it slows down even more.
So what happens when light slows down?
So let's say light is traveling through air and it hits water.
The light slows down in the water and it bends,
it changes direction.
That's one of the weird results of light changing speed.
This bending of light is called refraction.
And you've probably see light refracted
if you put a spoon or a straw into a glass of water.
It looks like the spoon is bent at the surface of the water
because of refraction.
We actually did a video where I took a straw,
and I put it into the water, and then looked into the straw,
moved it up and down, and you can see the image
at the bottom getting blown up into this big image
and then shrinking away far down
as you bend the surface of the water at the top.
This is actually how lenses work.
They are designed to bend and refocus light
to different parts of your eyes as the light enters
either a glass lens, or a plastic lens,
or even a water lens so you get a sharper image.
Cool.
So all sorts of cool things happen when
waves interact with matter.
Another example is the Doppler effect.
ANNOUNCER: The three remaining cars
take the checkered flag, thus ending
what must be counted as one of the most
astounding performances in automobile history.
So say the source of your light waves
or your sound waves is moving toward or away from you.
So you know what it sounds like when a parked fire truck
has its sirens blaring.
It's emitting these sound waves with a certain wavelength.
But now imagine that the fire truck is driving toward you.
So then between each peak in the sound waves
it emits, the truck gets a little bit closer.
So that means overall the waves are
going to get squished together to a shorter wavelength.
And if the wavelength goes down,
that means the frequency goes up.
So it sounds higher pitched as it comes toward you.
When this fire truck passes you, the opposite is happening.
As the truck moves away, the wavelength
gets longer and spread out between these peaks.
The wave is emitted, truck moves away, the next peak is emitted.
And it becomes lower pitched as it's moving away.
So this is called the Doppler effect--
waves getting shorter or longer because the source
is moving toward or away from you.
And you can hear it change as it goes by, that--
[MAKES NOISE UP TO DOWN]
So maybe you can figure out why you never hear--
[MAKES NOISE DOWN TO UP]
Hmm, intriguing.
Now what if the moving object is emitting light waves,
like a star?
So if a star is moving toward us,
its light waves actually get squished
into shorter wavelengths, which means--
what does it mean for the color?
It means it gets bluer.
And if it's moving away, then the same thing.
The light wave gets stretched to longer,
redder wavelengths of light.
So it gets redder.
We call that red shift and blue shift.
You can understand the joke on the bumper sticker
my high school physics teacher had on his car that said--
it was a red sticker.
And it said, "If this sticker is blue,
you are traveling too fast."
Oh, wow.
We just did a lot.
[SIGH]
All with a few simple ideas about relating
the wavelength and frequency of waves to their speed.
And we've just barely scratched the surface.
We didn't even get to reflection of light.
Sometimes light doesn't go into the surface, it reflects off.
That's called reflection.
And we've also got transmission,
where light waves go through the medium.
There's diffraction, and dispersion, and standing waves,
which are super cool.
There's the physics of music.
We just don't have time to cover all of this.
But that's the great part about physics,
because the more you learn, the more you
realize you have yet to understand.
So that's our lesson today on waves, light, and sound.
When they ask you what you learned on YouTube today,
here are your key takeaways, or should I say take "a-waves."
Number one, waves carry energy and information.
And they're defined as transverse and longitudinal.
And number two, v equals Lambda times f.
The speed of a wave is equal to its wavelength
times its frequency.
And here are all the problems that we solved
in today's lesson so that you can go solve them
on your own, because solving problems
is a super important part of learning
and understanding physics.
And one last thing.
One really cool little bit of physics--
physics history, actually.
Stick around for this.
This is pretty mind-blowing.
In the 1910s and 1920s, astronomers
measured the Doppler shift of objects
that they called spiral nebulae,
which are these weird, fuzzy patches of light
in the sky with spiral patterns that they assumed
were inside of the Milky Way.
But it turns out that a lot of these objects
had longer, redder wavelengths than they should have had,
meaning, now you know, that they were moving away from us.
Astronomers measured the distances
of these spiral nebulae and figured out
that they were not in the Milky Way.
They were actually their own galaxies.
And the further away a galaxy was,
it turned out the faster it appeared to be moving away.
Now we know why.
We know it's because the entire universe is expanding.
And galaxies and galaxy clusters are moving apart
from one another because of that expansion.
That's why all these galaxies appear to be moving away.
We don't know why the universe is expanding.
What we do know is that there's some source of energy
we don't quite understand that's making the universe expand
faster and faster.
And we call that energy "dark energy."
Super cool stuff.
And if you continue on with physics and take a class in,
say, cosmology, you get to learn a lot more
about dark energy and this crazy type of physics.
[SIGH] That's it.
And now for a message from a special guest.
Hey, what's going on?
My name is Destin Sandlin.
I'm a mechanical and aerospace engineer,
and I love physics.
So I was super excited to learn
that you were learning physics with Dianna.
And I want to encourage you because, for me,
physics is like this storybook,
like you get more and more understanding
about the world around you as you peel back
the pages of the story.
But the cool thing is the more understanding you get,
the more there is to understand.
Like you can go deeper and deeper and deeper,
and I just love it.
So I am super excited that you're studying with Dianna.
And I would like to encourage you to just go
as far as you can with it.
Anyway, nice to meet you. My name is Destin.
And I hope you fall in love with physics. Bye.
[MUSIC PLAYING]
Browse More Related Video

Dr Quantum Double Slit Experiment

Fourier Transform, Fourier Series, and frequency spectrum
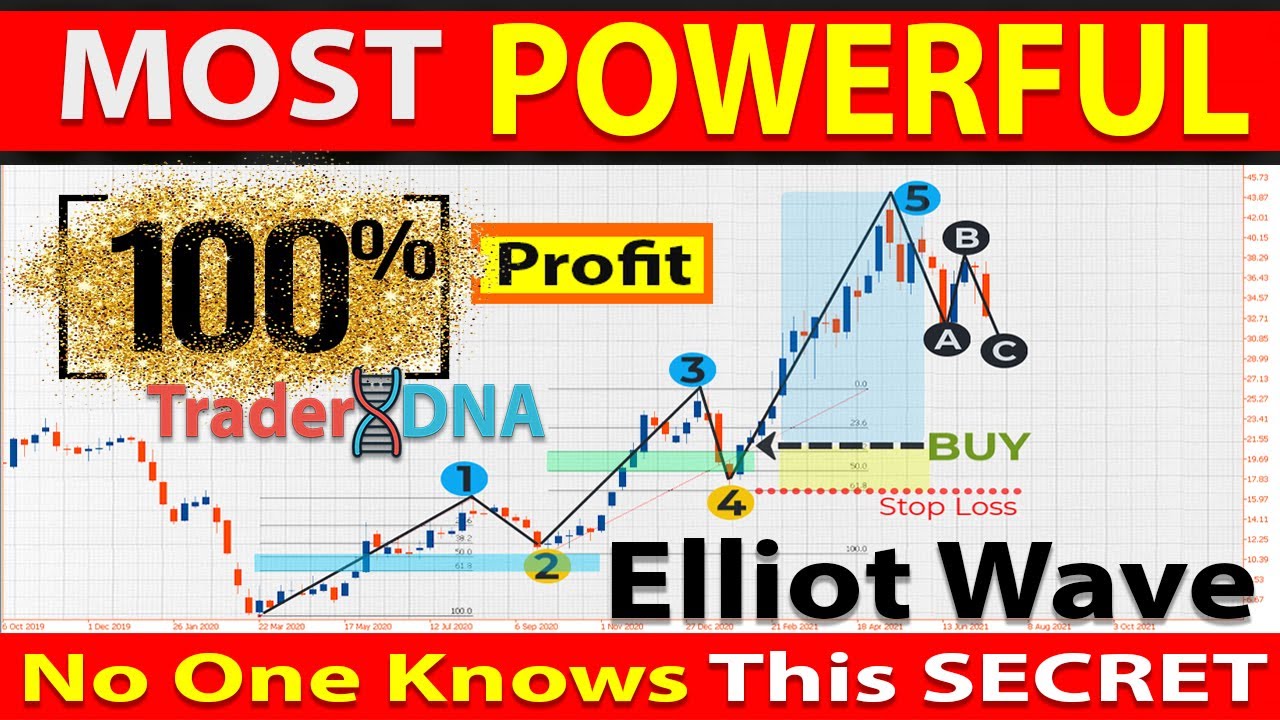
π΄ Most Effective "ELLIOT WAVE and FIBONACCI" Price Action Trading Strategy (Wave Trading Explained)
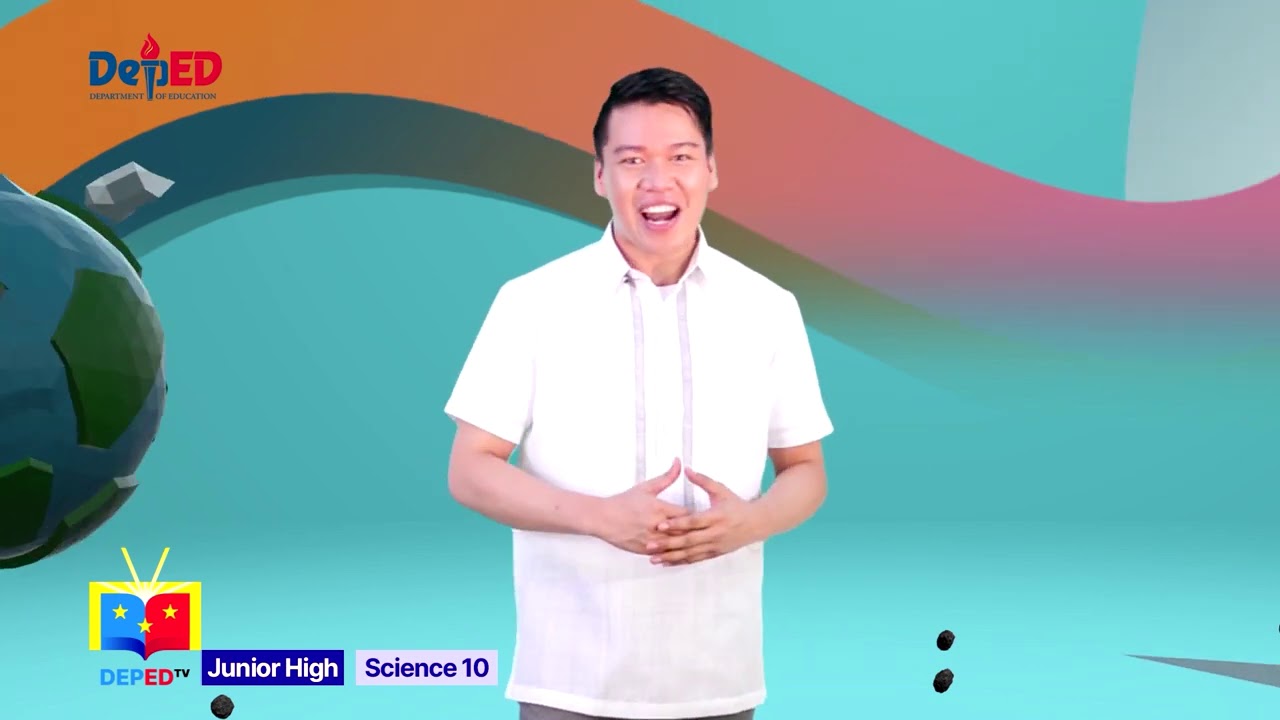
Grade 10 Science Q1 Ep 2: Earthquake Epicenter
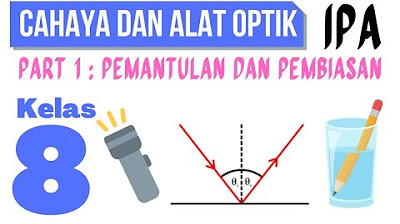
CAHAYA DAN ALAT OPTIK (PART 1) : IPA KELAS 8 SMP

Conservation of Momentum | Elastic and Inelastic Collision | Grade 9 Science Quarter 4 Week 4
5.0 / 5 (0 votes)