Synthetic Biology: Principles and Applications - Jan Roelof van der Meer
Summary
TLDR简述了合成生物学的基本原理及其应用。合成生物学通过理解生物过程的重建而非解构,以及构建具有新功能的复杂生物过程,来实现对生物体的深入理解。通过标准化生物部件、DNA合成、设计基因组等手段,合成生物学正推动着医药、农业、工业和环境等多个领域的创新。特别介绍了生物报告器的应用,这是一种工程化细菌,能够检测环境中的有毒化学物质,如砷,并在实验室外进行实地测试,展示了合成生物学在环境监测中的潜力和实际应用。
Takeaways
- 🧬 合成生物学是将生物学原理应用于设计和构建新生物部件、设备和系统,与传统生物学的观察、突变分析和解剖方法形成对比。
- 🔬 合成生物学家通过将DNA序列分解为可研究的生物部件,再将这些部件重新组装来构建新的生物功能。
- 📚 合成生物学中的规则和模型类似于电子工程中的逻辑规则,用于预测和控制基因表达和蛋白质行为。
- 🏭 合成生物学的一个核心概念是标准化,类似于电子工程中的插头标准,使得不同实验室和行业能够共同协作。
- 🛠️ 合成生物学的目标是通过重建复杂生物过程来理解它们,以及设计具有新功能的复杂生物过程。
- 🌱 合成生物学的研究活动包括标准化部件制造、DNA合成、基因组设计、最小细胞生产平台、原细胞和人工生命研究,以及Xeno-DNA研究。
- 💊 合成生物学在医疗健康领域具有广泛的应用潜力,包括制药、疫苗、基因疗法、组织工程、益生菌、诊断等。
- 🌾 在农业领域,合成生物学可以帮助改良植物,使其抵抗疾病和干旱,提供更好的动物饲料,并有助于CO2固定。
- ♻️ 合成生物学在环境领域也有应用,如通过工程细菌监测环境污染,进行生物修复和废物处理。
- 🔬 生物报告器是合成生物学的一个具体应用,它们是经过工程改造的细菌,能够感应环境中的有毒化学物质,并通过发光或荧光来报告。
- 🌐 合成生物学的市场价值预计在2016年达到100亿美元,涉及医药、化学品、农业和能源等多个领域。
Q & A
合成生物学的基本原理是什么?
-合成生物学的基本原理包括将DNA分解为生物部件,理解这些部件如何协同工作(规则和模型),以及建立标准以促进不同实验室和行业之间的合作。合成生物学家将这些部件重新组合,构建新的或修改过的生物系统。
合成生物学与传统生物学在研究方法上有何不同?
-传统生物学通常通过观察、突变创造和解剖来理解生物体,而合成生物学则通过将DNA分解为部件,利用规则和模型来理解这些部件如何协同工作,并建立标准来促进不同实验室和行业之间的合作。
合成生物学中的“生物报告器”是什么?
-生物报告器是合成生物学中一种经过工程改造的细菌细胞,它们可以被赋予不同的颜色,如生物发光或荧光,用于检测环境中的有毒化学物质。当这些细胞检测到特定化合物时,会触发产生光或荧光的反应。
合成生物学在环境监测方面有哪些潜在应用?
-合成生物学在环境监测方面的潜在应用包括使用生物报告器来检测水体中的有毒化学物质,如砷,以及测量海洋中的石油污染。这些生物报告器可以快速、低成本地提供环境质量的直接测量。
合成生物学在农业方面的应用有哪些?
-合成生物学在农业方面的应用包括改进植物以抵抗疾病、耐旱,并提供更好的动物饲料,以及可能帮助固定二氧化碳。这些应用有助于提高作物产量和质量,同时减少对环境的影响。
合成生物学如何帮助生产新的化学物质和材料?
-合成生物学可以通过设计和构建具有新功能的复杂生物途径来帮助生产新的化学物质和材料。例如,合成生物学可以用于生产生物能源、生物燃料、大宗化学品、特殊化学品以及创造新型材料。
合成生物学在医疗健康领域的潜在应用是什么?
-合成生物学在医疗健康领域的潜在应用包括生产新的药物、疫苗、基因疗法、组织工程、益生菌和诊断工具。这些应用有助于改善疾病治疗、预防和健康监测。
合成生物学中的“底盘”概念是什么?
-在合成生物学中,“底盘”是指一个基本的生物体,它可以被用作平台来插入或修改特定的生物学功能。就像汽车制造中的底盘一样,底盘提供了一个基础,可以在上面添加不同的组件。
合成生物学如何促进DIY社区的发展?
-合成生物学通过提供简单的生物实验和工具,使得业余爱好者和非专业人士能够参与到生物学的研究和实践中来。这种DIY(自己动手做)的方法有助于普及生物学知识,并激发公众对科学的兴趣。
合成生物学的全球市场价值预计会如何增长?
-根据2011年的一份报告,合成生物学的全球市场价值预计从2011年的16亿美元增长到2016年的100亿美元。这表明合成生物学被视为一个具有巨大潜力和重要性的市场。
Outlines
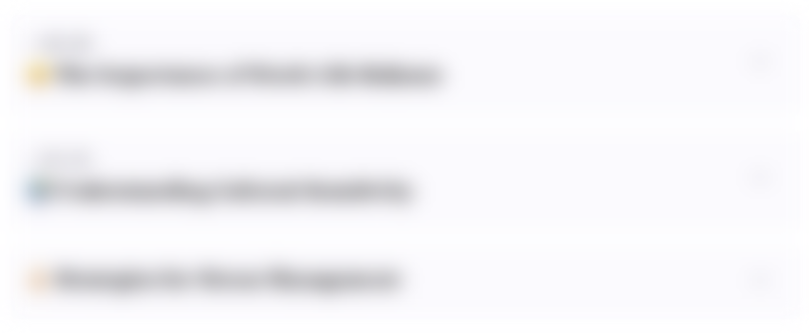
This section is available to paid users only. Please upgrade to access this part.
Upgrade NowMindmap
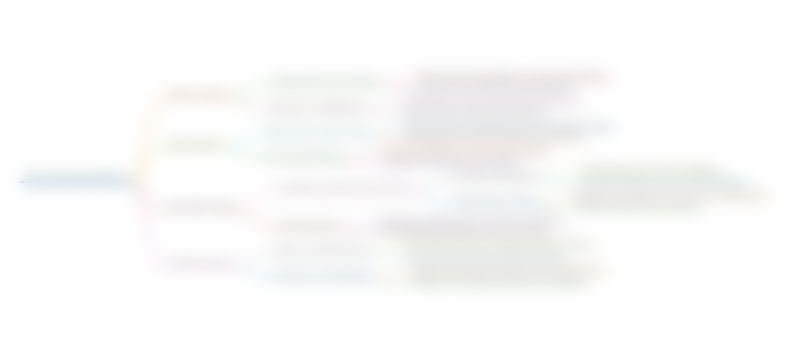
This section is available to paid users only. Please upgrade to access this part.
Upgrade NowKeywords
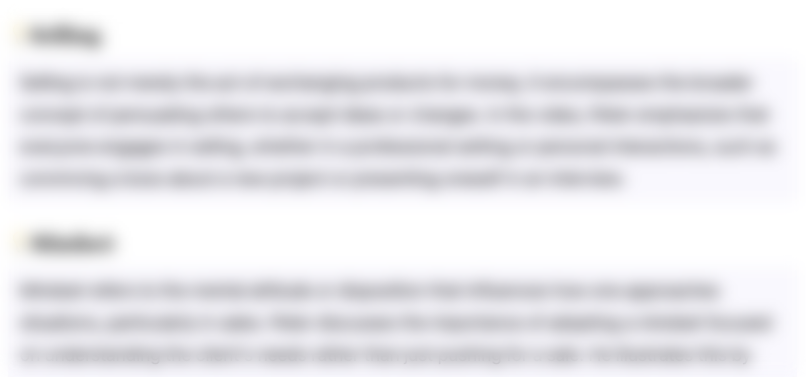
This section is available to paid users only. Please upgrade to access this part.
Upgrade NowHighlights
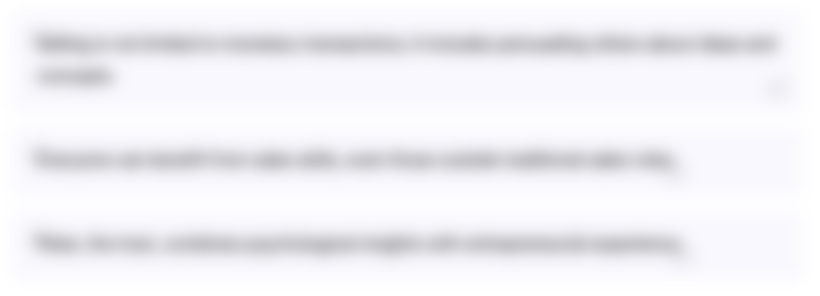
This section is available to paid users only. Please upgrade to access this part.
Upgrade NowTranscripts
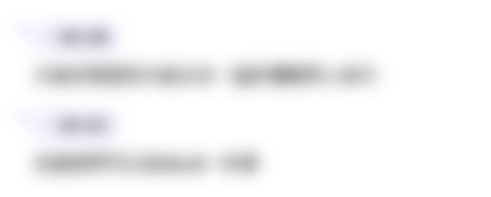
This section is available to paid users only. Please upgrade to access this part.
Upgrade NowBrowse More Related Video
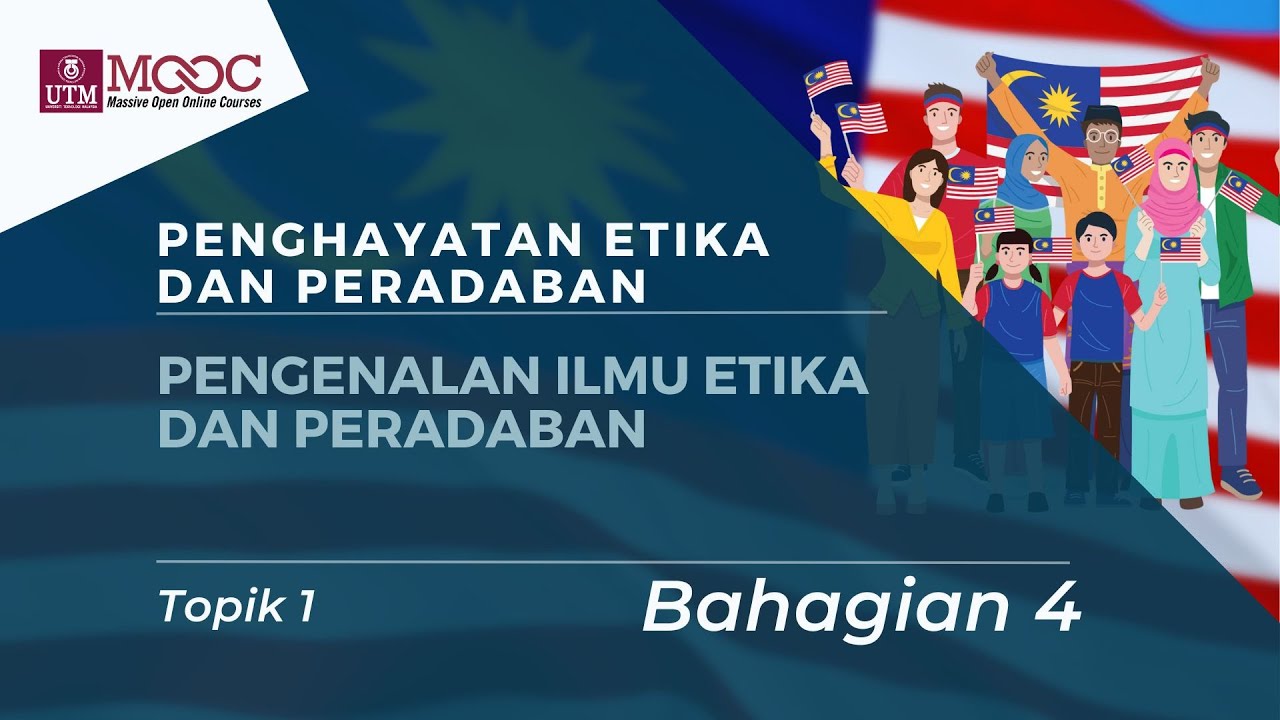
Mooc_Penghayatan Etika & Peradaban_ Topik 1- Bahagian 4
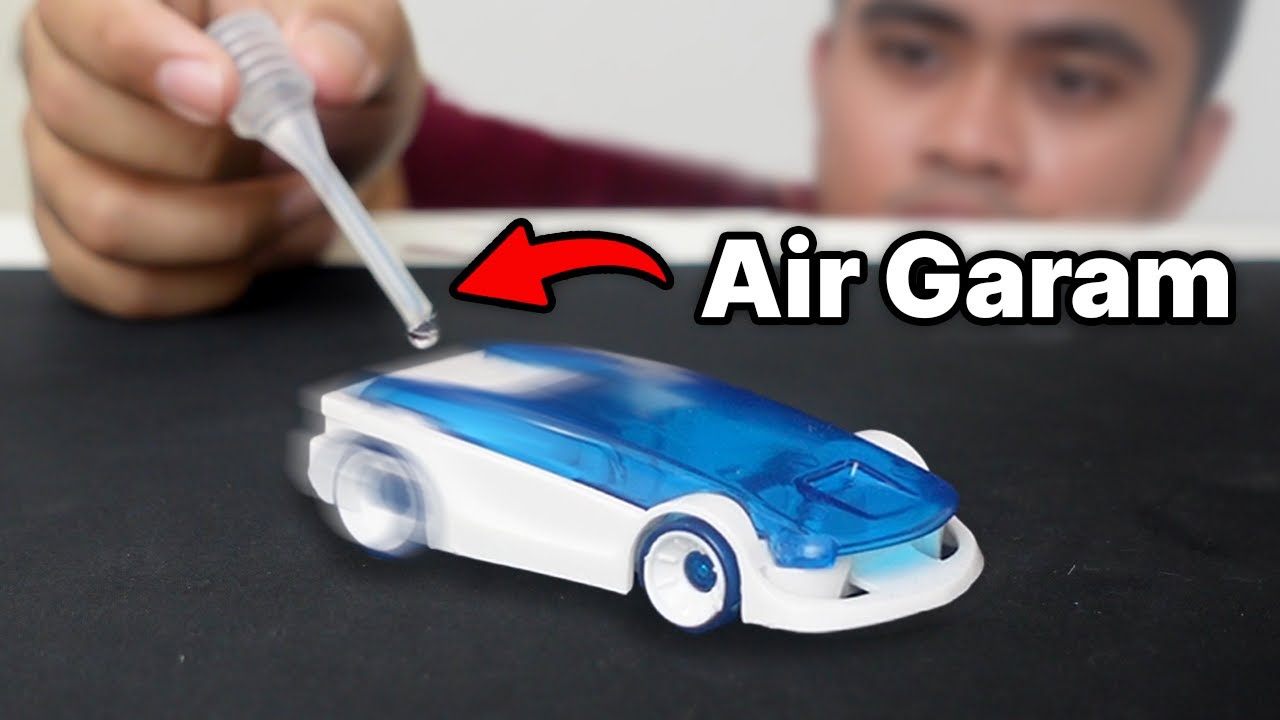
Mobil mainan ini bergerak dengan bahan bakar air. Mari kita buktikan.
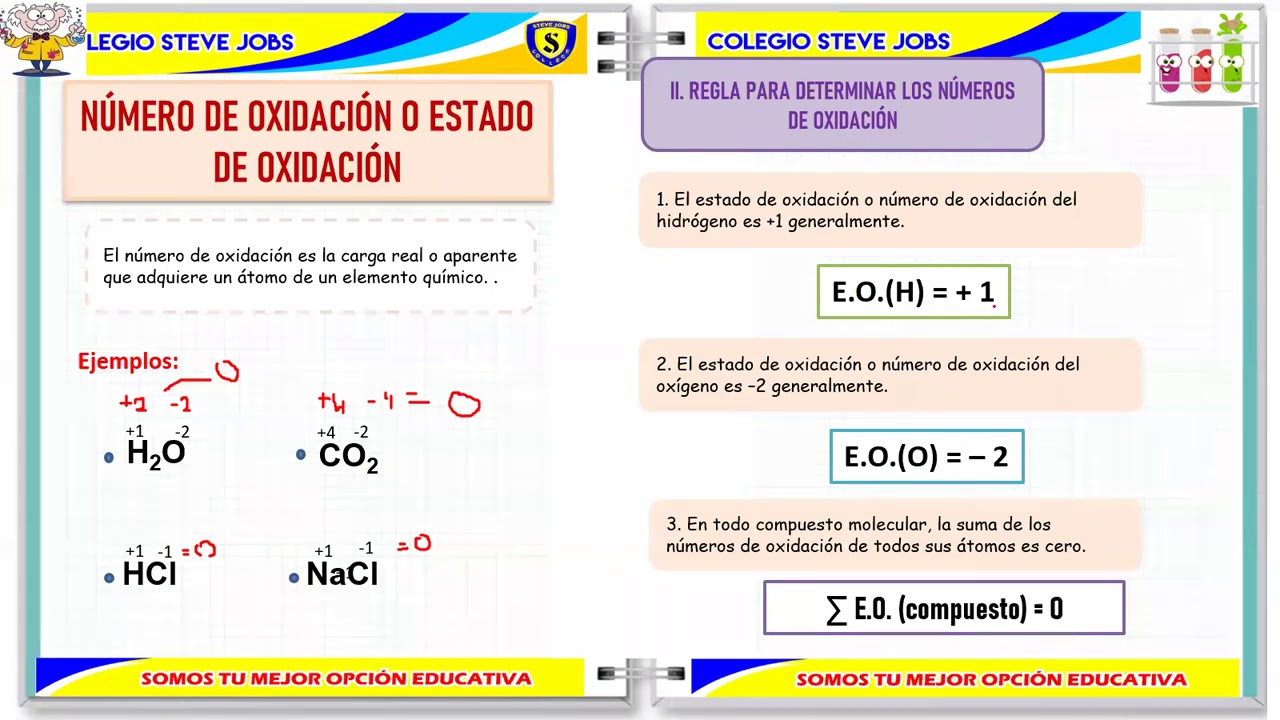
NOMENCLATURA INORGÁNICA- ESTADOS DE OXIDACIÓN
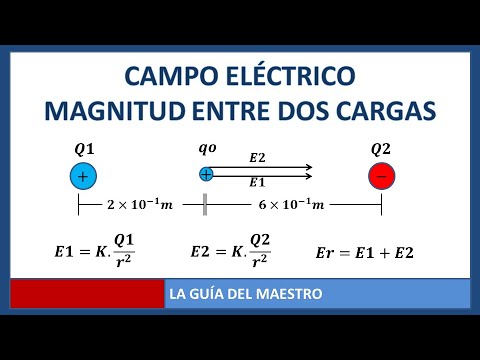
Electric field magnitude between two point charges

Geoffrey Hinton: The Foundations of Deep Learning
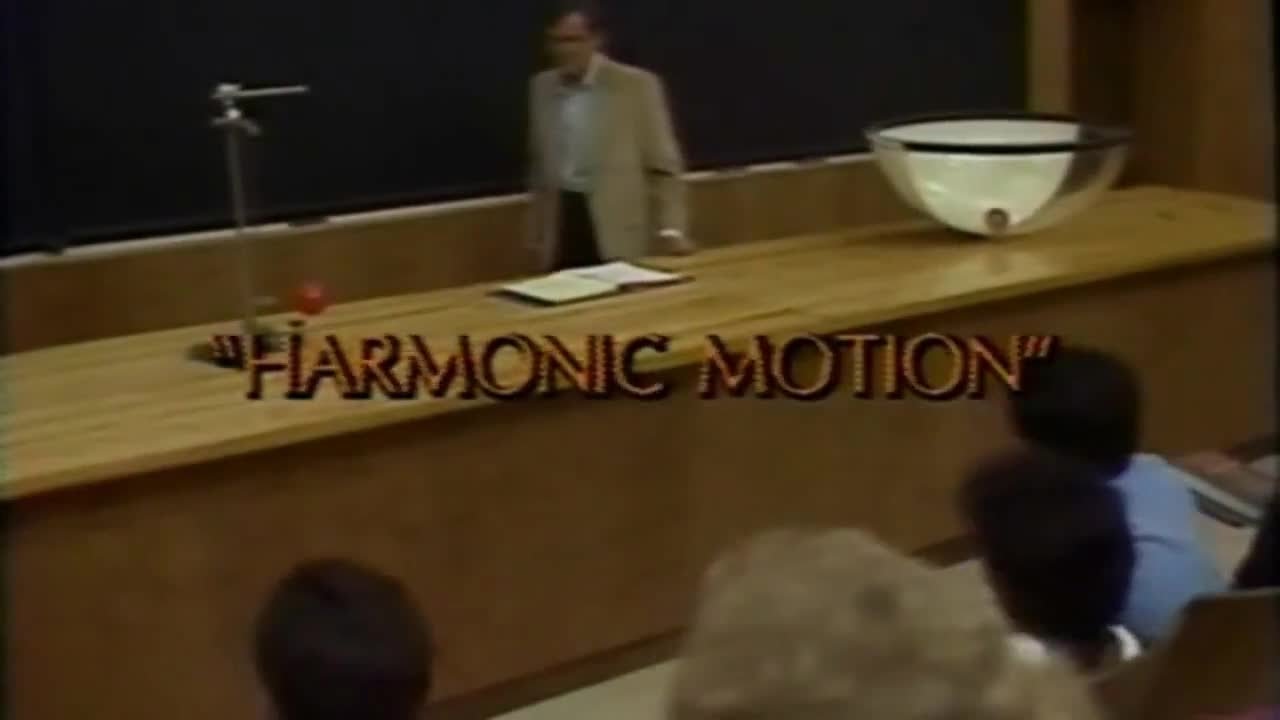
"Movimiento Armónico Simple" (Documental)
5.0 / 5 (0 votes)