First law of thermodynamics / internal energy | Thermodynamics | Physics | Khan Academy
Summary
TLDRThis educational video script delves into the first law of thermodynamics, highlighting the principle that energy cannot be created or destroyed but only transformed. The presenter uses the example of a ball thrown into the air to illustrate the conversion of kinetic energy to potential energy and back, and how air resistance results in a loss of kinetic energy due to heat transfer. The concept of internal energy as a macroscopic description of all energy within a system is introduced, with a focus on its components like kinetic and potential energy. The script concludes with a discussion on how changes in internal energy relate to heat transfer and work done, setting the stage for further exploration in upcoming videos.
Takeaways
- 🔄 The first law of thermodynamics states that energy cannot be created or destroyed, only transformed from one form to another.
- 🏋️♂️ Kinetic energy is the energy of movement, and as an object slows down, its kinetic energy decreases.
- 📈 Potential energy is the stored energy an object possesses due to its position or condition, which can be converted into other forms of energy.
- 🪁 When a ball is thrown upwards, its kinetic energy is converted into potential energy at its peak, and then back into kinetic energy as it falls.
- 💨 Air resistance can reduce the kinetic energy of a falling object, converting some of it into heat through friction with air molecules.
- 🌡️ Temperature is a measure of the average kinetic energy of the particles in a substance, reflecting their movement.
- 🔄 Internal energy (U) encompasses all the energy contained within a system, including kinetic and potential energies of particles.
- 🔧 The change in internal energy (∆U) can be calculated as the heat added to the system (Q) minus the work done by the system (W), or as Q plus the work done on the system, depending on the perspective.
- ⚖️ In thermodynamics, work and heat transfer are ways of energy exchange between a system and its surroundings, affecting the system's internal energy.
- 📚 The video script promises further exploration of these concepts, including practical examples and different notations used in thermodynamics.
Q & A
What is the first law of thermodynamics?
-The first law of thermodynamics states that energy cannot be created or destroyed, only transformed from one form to another.
How does the concept of kinetic energy relate to the first law of thermodynamics?
-Kinetic energy is a form of energy that is associated with the motion of an object. According to the first law of thermodynamics, when an object's kinetic energy decreases, it does not disappear but is transformed into another form of energy, such as potential energy.
What is potential energy and how does it connect to the first law?
-Potential energy is the stored energy of an object due to its position or condition. It can be converted into other forms of energy, like kinetic energy. The first law of thermodynamics ensures that this conversion does not create or destroy energy but merely transforms it from one form to another.
Can you explain the example of throwing a ball up in the air in terms of energy transformation?
-When a ball is thrown up, its kinetic energy is converted to potential energy as it rises and comes to a stop at its peak. On the way down, potential energy is converted back to kinetic energy. If air resistance is considered, some of the kinetic energy is transformed into heat due to friction with air molecules.
What happens to the energy when air resistance is present during the ball's motion?
-In the presence of air resistance, some of the ball's kinetic energy is converted into heat as the ball rubs against air molecules, causing them to vibrate faster. This results in the ball having less kinetic energy when it returns to the starting point compared to when it was initially thrown.
What is internal energy and how is it measured?
-Internal energy is the total energy contained within a system, which includes kinetic and potential energies of molecules, energy from molecular bonds, and other forms of energy present. It is typically measured as the change in internal energy (ΔU), which can be calculated from the heat added to the system (Q) and the work done by the system (W).
How does the first law of thermodynamics relate to the concept of internal energy?
-The first law of thermodynamics implies that the change in internal energy of a system is equal to the heat added to the system minus the work done by the system. This relationship shows that energy is conserved, with internal energy changes resulting from transfers of energy in the form of heat and work.
What is the significance of the equation ΔU = Q - W in thermodynamics?
-The equation ΔU = Q - W is a mathematical representation of the first law of thermodynamics. It shows that the change in internal energy (ΔU) of a system is equal to the heat added to the system (Q) minus the work done by the system (W), illustrating the conservation of energy principle.
Why is it important to understand the difference between heat (Q) and work (W) in thermodynamics?
-Understanding the difference between heat and work is crucial because they represent different modes of energy transfer. Heat is the transfer of energy due to a temperature difference, while work is the transfer of energy by a force moving an object. Recognizing these differences helps in accurately applying the first law of thermodynamics to various systems.
Can you provide an example of how internal energy can change in a system?
-An example of internal energy changing in a system is when a gas is heated. As the gas absorbs heat (Q is positive), its internal energy increases (ΔU is positive). If the gas expands and does work on its surroundings (W is positive), the increase in internal energy will be the sum of the heat added and the work done by the gas.
Outlines
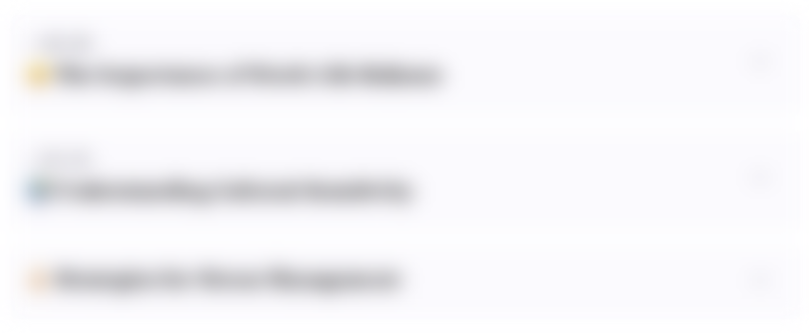
This section is available to paid users only. Please upgrade to access this part.
Upgrade NowMindmap
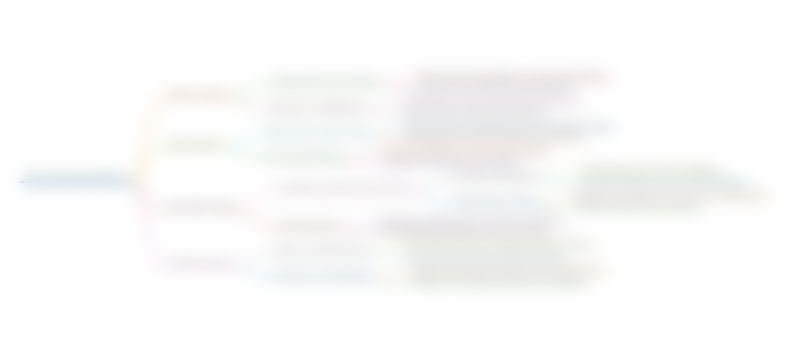
This section is available to paid users only. Please upgrade to access this part.
Upgrade NowKeywords
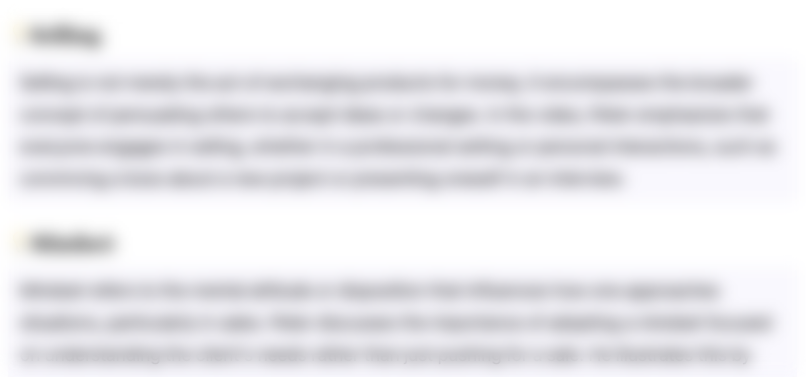
This section is available to paid users only. Please upgrade to access this part.
Upgrade NowHighlights
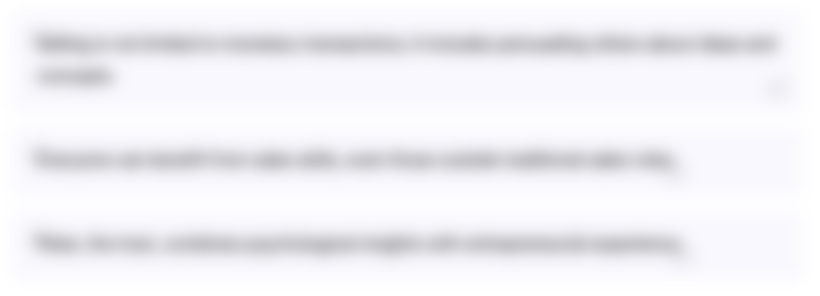
This section is available to paid users only. Please upgrade to access this part.
Upgrade NowTranscripts
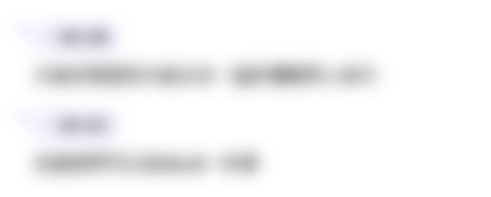
This section is available to paid users only. Please upgrade to access this part.
Upgrade NowBrowse More Related Video
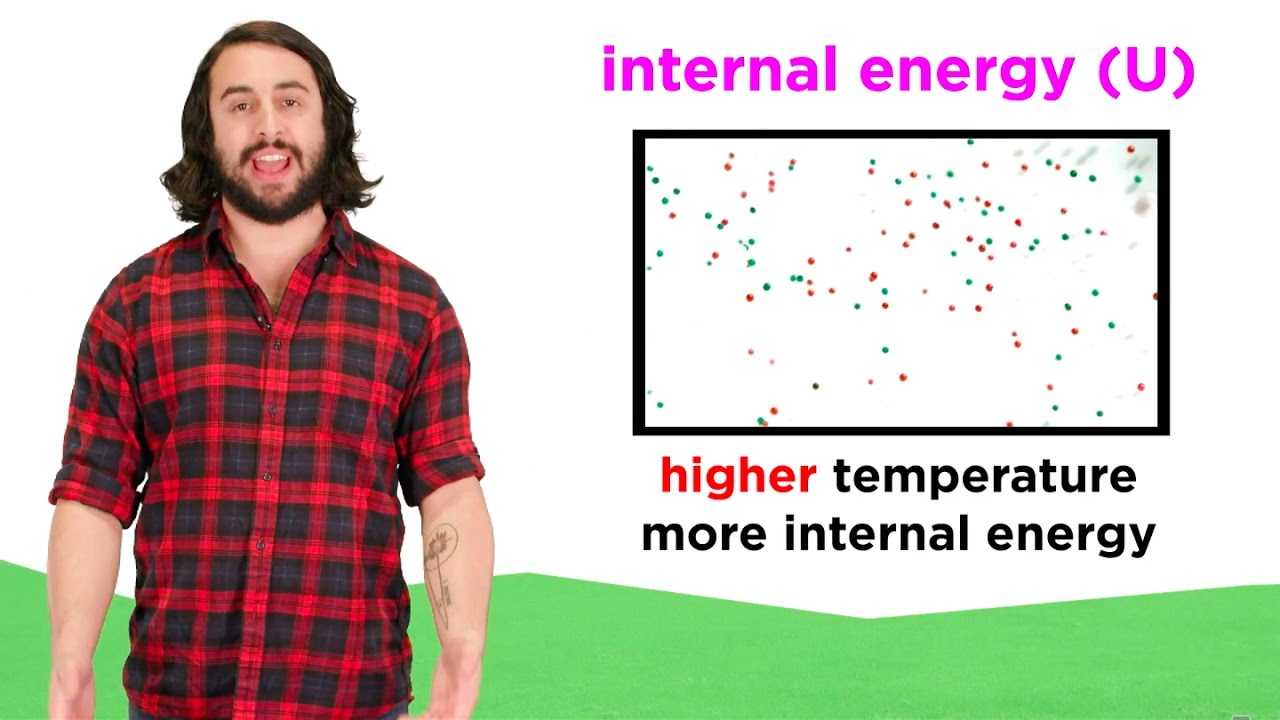
Internal Energy

Thermodynamics: Energy, Work and Heat (Animation)

O que é a energia cinética e a energia potencial? | 10F1.1 | Aula 1
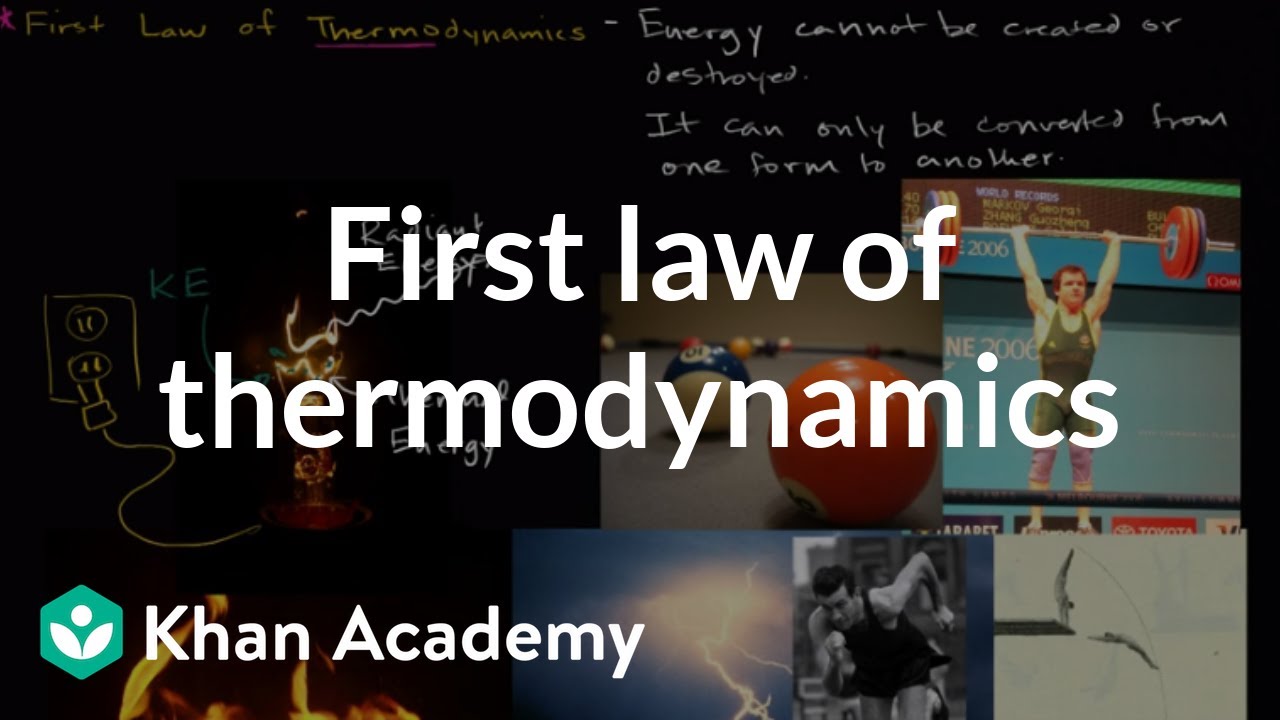
First Law of Thermodynamics introduction | Biology | Khan Academy
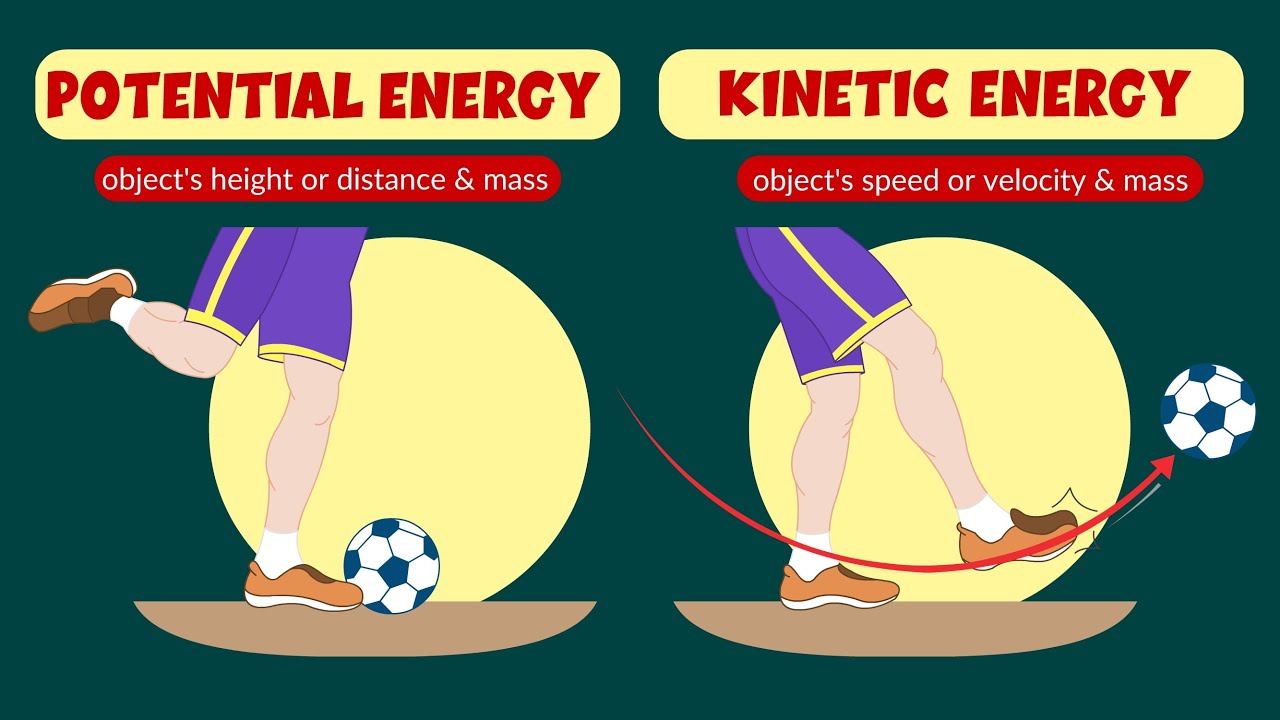
Potential and kinetic energy - Law of conservation of energy - Video for kids
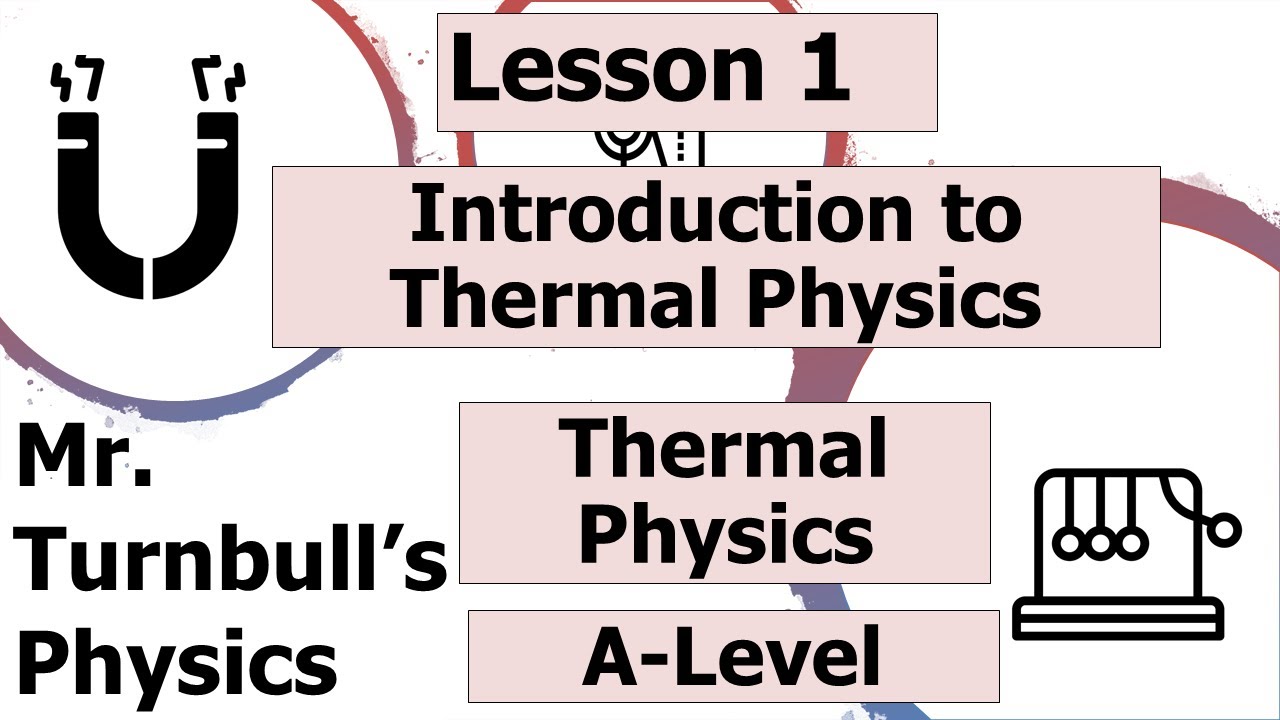
Introduction to Thermal Physics
5.0 / 5 (0 votes)