3D Structure and Bonding: Crash Course Organic Chemistry #4
Summary
TLDRIn this episode of Crash Course Organic Chemistry, Deboki Chakravarti explains the 3D structures of organic molecules using theories like VSEPR, valence bond theory, and orbital hybridization. The episode covers how these concepts explain molecular shapes and bond formation in molecules like methane, ethene, and ethyne. The difference between constitutional and geometric isomers is also explored, emphasizing the impact of bond structure on molecular behavior. Understanding these principles helps explain everything from basic organic compounds to the structure of DNA.
Takeaways
- 🧬 Understanding 3D molecular shapes is crucial for grasping how organic molecules interact and function in living organisms.
- 📚 VSEPR theory, introduced in 1957, explains the 3D shapes of molecules based on electron pair repulsion, which is key to understanding molecular geometry.
- 🔍 Organic chemistry frequently uses three of the five VSEPR geometries: linear, trigonal planar, and tetrahedral, which are essential for depicting molecular structures.
- 🌐 Molecular shapes are described by the arrangement of atoms, ignoring lone pairs, which is different from electron-pair geometries that consider both bonds and lone pairs.
- 🧠 The concept of orbital hybridization, where atomic orbitals mix to form hybrid orbitals, is fundamental for explaining the 3D geometries predicted by VSEPR.
- 🔗 Sigma and pi bonds, explained by valence bond theory, are critical for understanding the structure of molecules with double and triple bonds.
- 🔬 The structure of DNA was a significant discovery in organic chemistry, made possible by understanding orbital hybridization and the role of hydrogen bonds.
- 🔍 Isomers, molecules with the same molecular formula but different atom arrangements, are categorized into constitutional isomers and geometric isomers.
- 🛠 Constitutional isomers differ in the connectivity of atoms, while geometric isomers differ in the spatial arrangement of atoms around a bond.
- 📈 The study of isomers and their properties is vital for understanding chemical reactions and the behavior of molecules in biological systems.
Q & A
What is the significance of understanding 3D molecular shapes in organic chemistry?
-Understanding 3D molecular shapes helps explain how the structure of a molecule affects its function. Some compounds fit together like puzzle pieces, while others don't, depending on their 3D geometry. This knowledge is crucial for understanding interactions like molecular bonding and reactivity.
What role does Valence Shell Electron Pair Repulsion (VSEPR) theory play in molecular geometry?
-VSEPR theory predicts the 3D shape of molecules based on the repulsion between electron pairs surrounding a central atom. It helps explain geometries like linear, trigonal planar, and tetrahedral shapes, which are key in organic chemistry.
How does orbital hybridization contribute to molecular shape?
-Orbital hybridization explains how atomic orbitals combine to form new hybrid orbitals, which determine the geometry of molecules. For instance, sp3 hybridization creates a tetrahedral shape, while sp2 hybridization forms a trigonal planar structure.
What is the difference between sigma and pi bonds?
-A sigma bond is formed by the direct overlap of orbitals along the bond axis, while a pi bond is formed by the sideways overlap of orbitals. Sigma bonds are stronger and exist in single bonds, whereas pi bonds appear in double and triple bonds.
How does the hybridization of carbon atoms affect the molecular shape of ethene and ethyne?
-In ethene (C2H4), carbon atoms undergo sp2 hybridization, creating a trigonal planar shape with a double bond formed by a sigma and a pi bond. In ethyne (C2H2), sp hybridization occurs, resulting in a linear geometry with a triple bond composed of one sigma and two pi bonds.
What is the importance of hydrogen bonding in the structure of DNA?
-Hydrogen bonds between nitrogenous bases hold the DNA double helix together. The correct orbital hybridization of atoms in DNA was crucial in understanding how these bonds form, which was key in determining the 3D structure of DNA.
What are constitutional isomers, and how do they differ from geometric isomers?
-Constitutional isomers have the same molecular formula but different connections between atoms, while geometric isomers have the same connections but differ in the spatial arrangement of atoms, usually due to restricted rotation around double bonds.
How does free rotation affect molecular structure and isomerism?
-Free rotation occurs around single bonds, allowing atoms to rotate freely without breaking the bond. In contrast, double bonds restrict rotation, leading to the formation of geometric isomers, where atoms are locked in specific spatial arrangements (cis or trans).
What is the significance of isomers in organic chemistry?
-Isomers are important because they show that molecules with the same molecular formula can have different structures and properties. This variation impacts the molecule’s reactivity, stability, and function in biological systems.
How does valence bond theory explain the formation of bonds in organic molecules?
-Valence bond theory describes how atomic orbitals overlap to form bonds, such as sigma and pi bonds. The type of overlap determines the strength and orientation of the bonds, which in turn defines the molecule’s 3D structure and reactivity.
Outlines
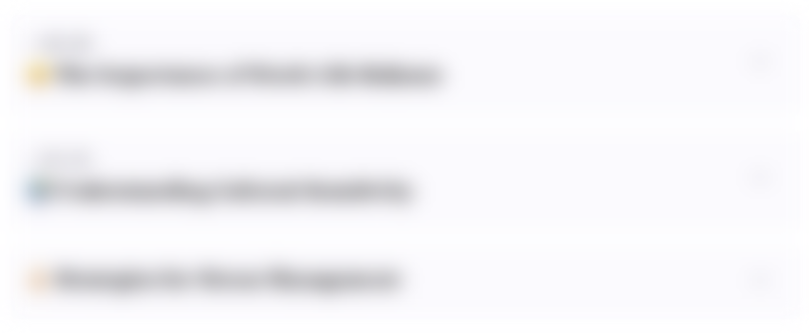
This section is available to paid users only. Please upgrade to access this part.
Upgrade NowMindmap
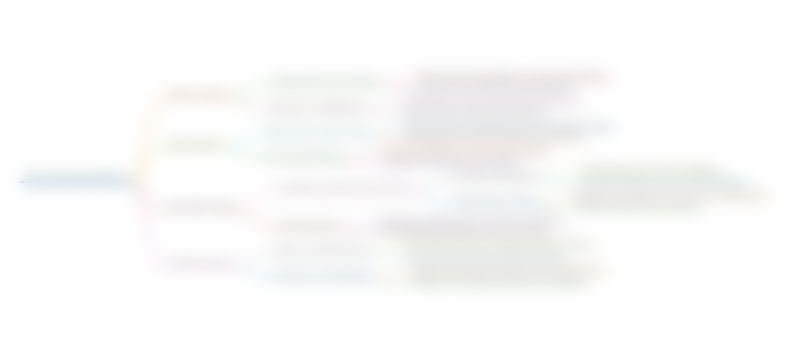
This section is available to paid users only. Please upgrade to access this part.
Upgrade NowKeywords
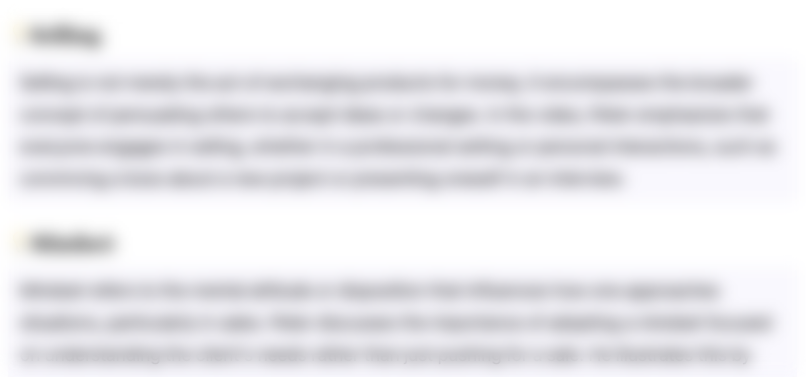
This section is available to paid users only. Please upgrade to access this part.
Upgrade NowHighlights
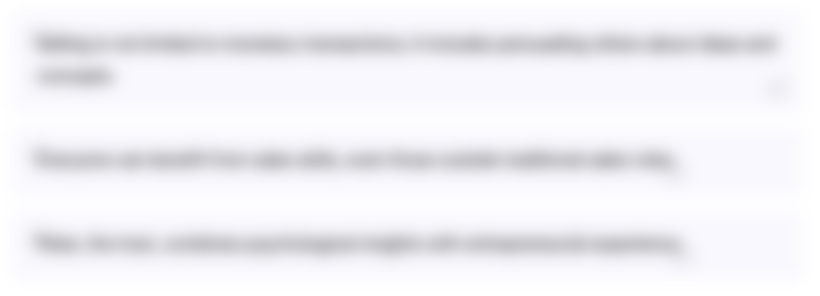
This section is available to paid users only. Please upgrade to access this part.
Upgrade NowTranscripts
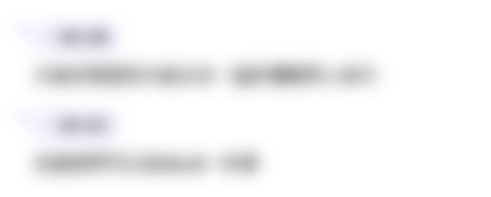
This section is available to paid users only. Please upgrade to access this part.
Upgrade NowBrowse More Related Video

Hybridization Theory (English)
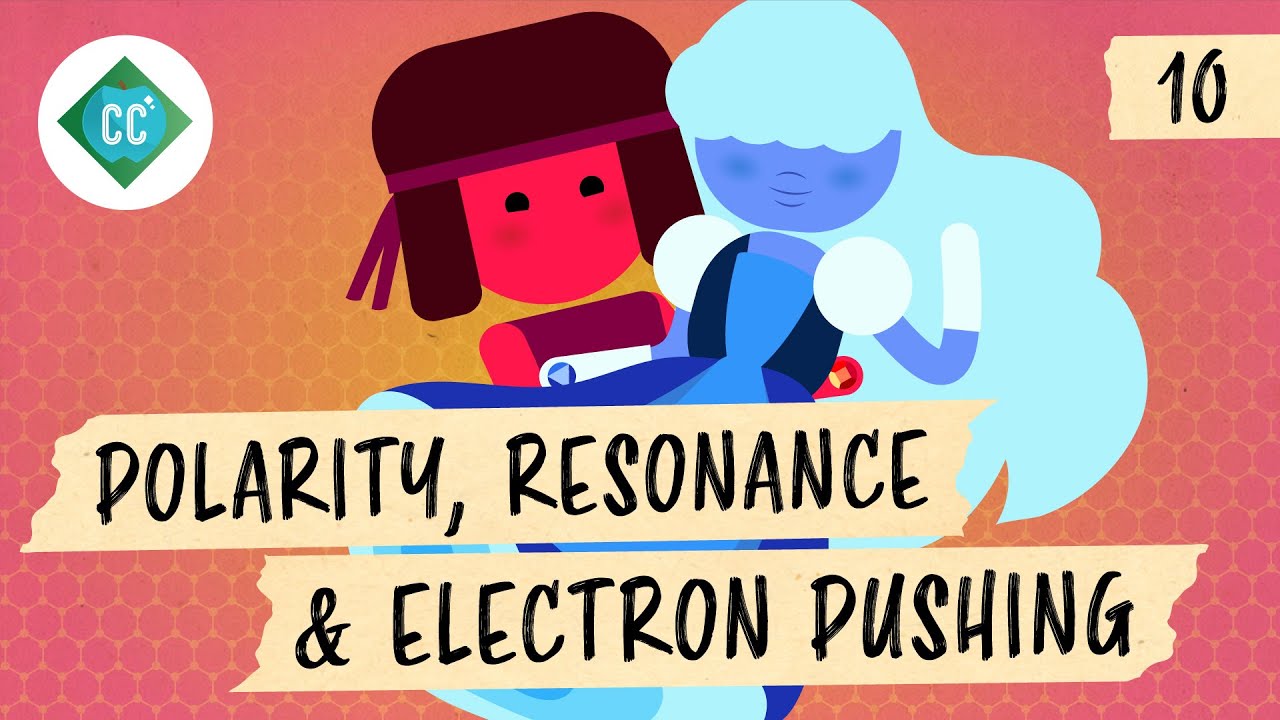
Polarity, Resonance, and Electron Pushing: Crash Course Organic Chemistry #10
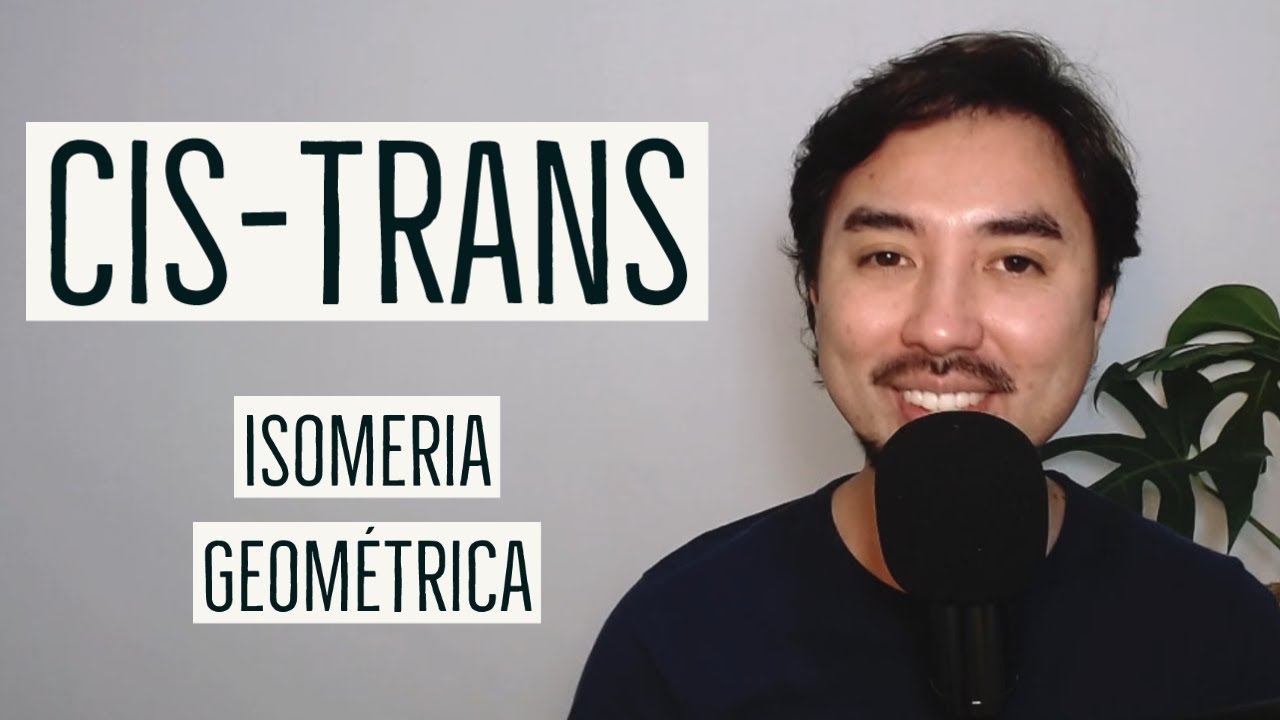
Isomeria Cis-Trans ou Geométrica | Aula 30
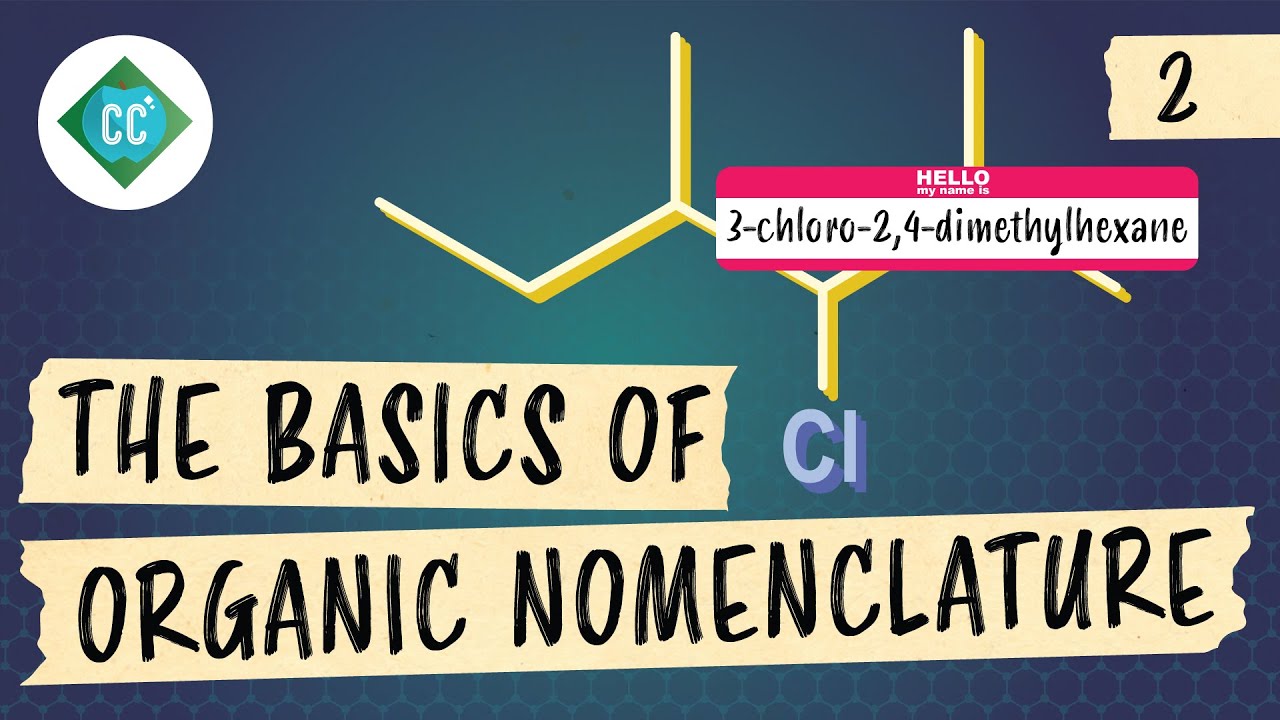
The Basics of Organic Nomenclature: Crash Course Organic Chemistry #2
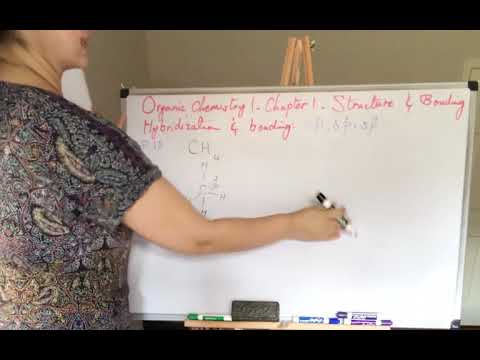
Organic Chemistry 1, Chapter 1-2: Structure & Bonding
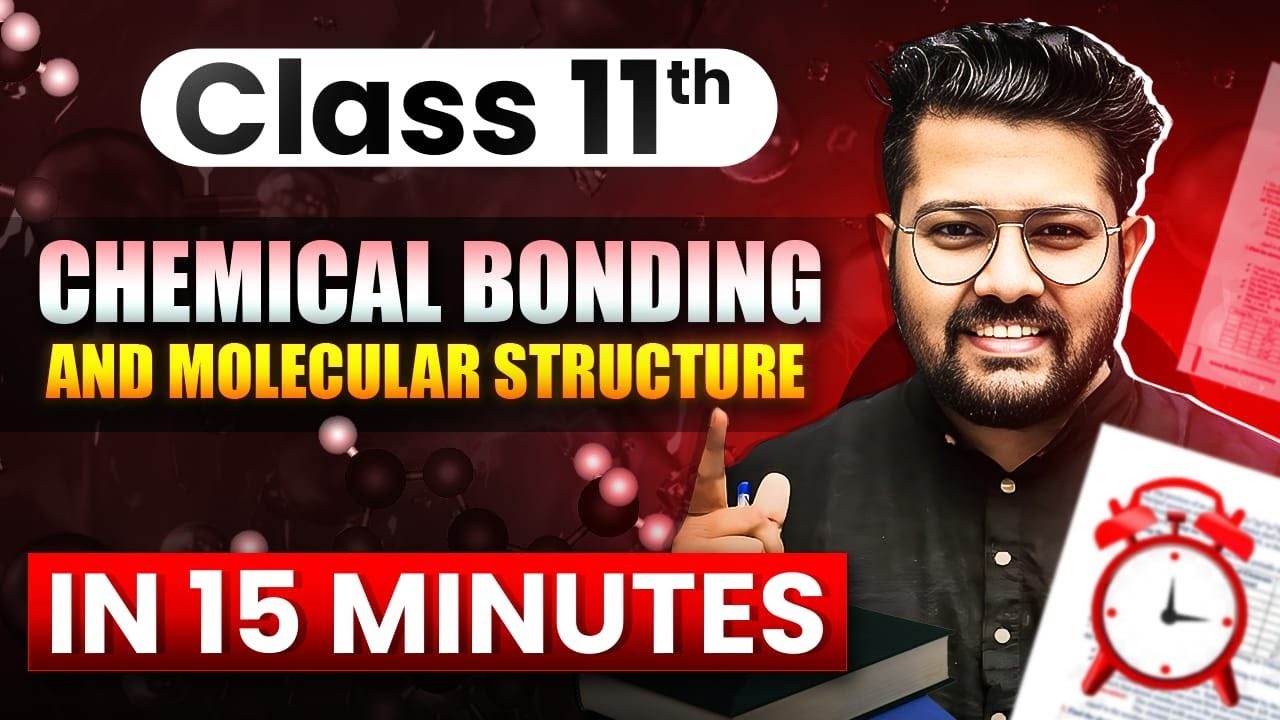
Class 11 Chemistry | Chemical Bonding and Molecular Str. in 15 Minutes | Rapid Revision by BP Sir
5.0 / 5 (0 votes)