Regulation of Gene Expression: Operons, Epigenetics, and Transcription Factors
Summary
TLDRProfessor Dave explores the intricacies of gene expression regulation, diving into the molecular processes of transcription and translation. He explains the role of post-transcriptional modifications, the central dogma of molecular biology, and how cells selectively express genes through regulatory mechanisms. The tutorial covers feedback inhibition, operons in bacteria, and both negative and positive gene regulation. It also touches on the role of histones, transcription factors, and enhancers in gene expression, providing a foundational understanding of these complex biological systems.
Takeaways
- 🧬 Gene expression is regulated at the molecular level through processes like transcription and translation, which convert genetic information into functional molecules within an organism.
- 📜 During transcription, DNA is transcribed into mRNA, which then undergoes modifications like the addition of a 5-prime cap and a poly-A tail, and splicing to remove introns.
- 🔄 mRNA moves from the nucleus to the cytoplasm where translation occurs, facilitated by ribosomes and tRNAs, resulting in the formation of polypeptides.
- 🔄 Post-translational modifications such as folding in the endoplasmic reticulum and modifications in the Golgi apparatus can occur, influencing the final protein structure.
- 🔄 Gene expression is regulated by various mechanisms to ensure that cells express only the necessary genes for their specific functions.
- 🔄 Feedback inhibition is a form of metabolic control where the accumulation of a metabolite can slow down its production pathway, conserving energy.
- 🔬 Bacterial cells use operons, which are groups of genes transcribed together and regulated by a repressor protein, to control gene expression.
- 🔬 Eukaryotic cells do not have operons but use other mechanisms like histone modification and transcription factors to regulate gene expression.
- 🔬 Positive and negative gene regulation involve signaling molecules interacting with DNA-binding proteins to either stimulate or inhibit gene expression.
- 🧠 Complex gene regulation is crucial for cellular differentiation and development, allowing cells with the same genetic material to take on distinct roles and functions.
Q & A
What is the central dogma of molecular biology?
-The central dogma of molecular biology illustrates how gene expression generates all of the proteins in an organism's body. It involves the processes of transcription, where DNA codes for mRNA, and translation, where the mRNA is used to produce a polypeptide.
What are the post-transcriptional modifications that occur to mRNA?
-Post-transcriptional modifications include the addition of a 5-prime cap, which is a modified guanine, and the poly-A tail, which consists of 50 to 250 adenines. Additionally, a spliceosome cuts out sections called introns, and the remaining sections, called exons, come together to form a smaller mRNA.
How does translation differ from transcription?
-During translation, a ribosome and many tRNAs work together with the mRNA to produce a polypeptide. This process occurs in the cytoplasm, whereas transcription takes place in the nucleus and involves the creation of mRNA from DNA.
What is feedback inhibition and how does it work?
-Feedback inhibition is a form of metabolic control where the products of certain enzymatic pathways act as inhibitors for those pathways. If a certain metabolite accumulates in a cell, it slows down the pathway by which it is generated, thus saving energy.
What is an operon and how does it function in gene regulation?
-An operon is a unit of DNA that includes a promoter, an operator, and several genes. It functions as an on-off switch for the transcription of those genes. A repressor can bind to the operator, blocking access to the promoter and thus inhibiting gene expression.
How does the presence of tryptophan affect the operon in E. coli?
-When tryptophan binds to the active site of the repressor in E. coli, it changes the repressor's shape, making it active and increasing its affinity for the operator. This causes the repressor to bind and stay on the operator, turning the operon off and inhibiting gene expression for tryptophan synthesis.
What is the role of allolactose in the regulation of lactose metabolism genes in E. coli?
-Allolactose, an isomer of lactose, binds to the repressor of the lactose metabolism genes in E. coli, deactivating it. This allows for transcription of the genes, leading to enzyme production and increased lactose metabolism.
What is the difference between negative and positive gene regulation?
-Negative gene regulation involves the repression of gene expression, either by a repressor binding to the operator or by deactivating a repressor. Positive gene regulation, on the other hand, involves the activation of gene expression through the binding of signaling molecules to activators, which then bind to DNA and stimulate transcription.
How do histones play a role in gene expression?
-Histones wrap DNA to form nucleosomes, and when genes are bound to histones, they cannot be expressed. For a gene to be expressed, it must become accessible, which can happen if an enzyme modifies a histone, decreasing its affinity for DNA and allowing the gene to be transcribed.
What are transcription factors and how do they contribute to gene regulation?
-Transcription factors are proteins necessary for transcription to proceed. Some bind to a promoter, often in a region called a TATA box, while others have activation domains that bind to other regulatory proteins to enhance transcription. The presence or absence of these factors can regulate the expression of other genes.
How do enhancers and activators interact to influence gene expression?
-Enhancers are control elements far away from the gene that interact with proteins called activators. When activators bind to the enhancer, another protein can bend DNA, bringing the activators closer to the promoter where transcription factors are located, thus influencing gene expression.
Outlines
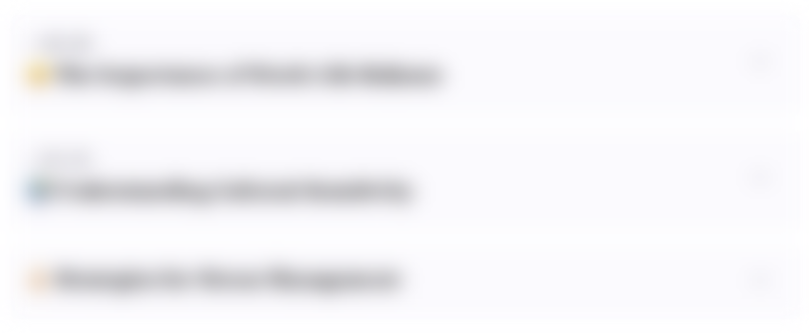
This section is available to paid users only. Please upgrade to access this part.
Upgrade NowMindmap
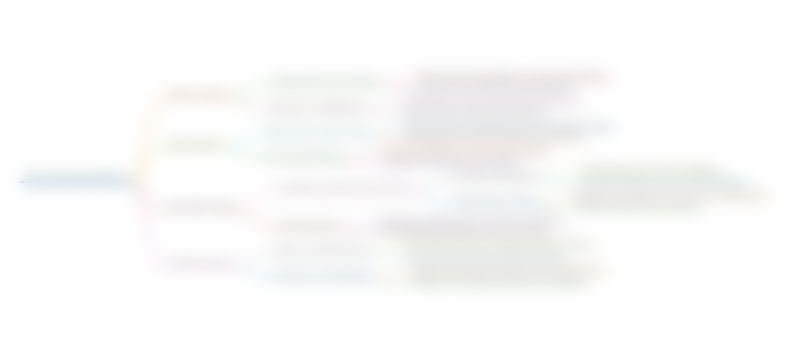
This section is available to paid users only. Please upgrade to access this part.
Upgrade NowKeywords
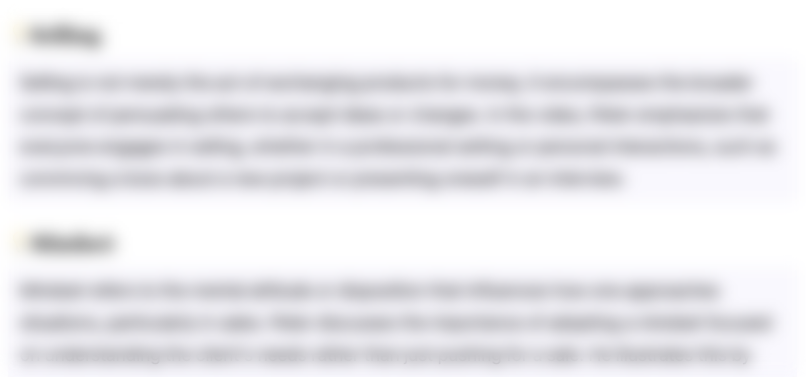
This section is available to paid users only. Please upgrade to access this part.
Upgrade NowHighlights
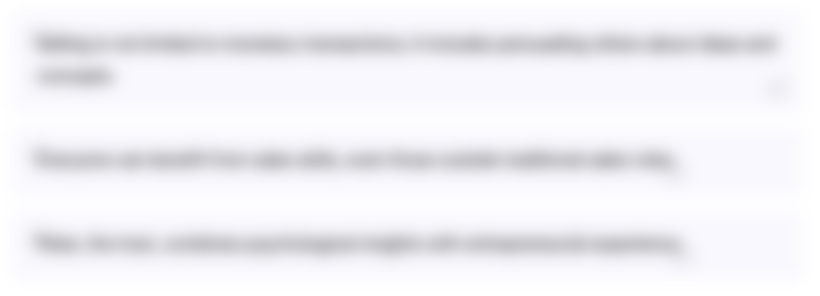
This section is available to paid users only. Please upgrade to access this part.
Upgrade NowTranscripts
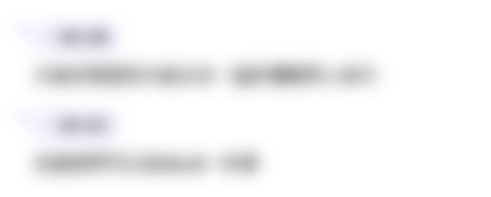
This section is available to paid users only. Please upgrade to access this part.
Upgrade Now5.0 / 5 (0 votes)