Electron Transport Chain
Summary
TLDRThis script delves into the intricate workings of mitochondria, the energy powerhouses of eukaryotic cells. It explains how mitochondria use a proton gradient across their inner membrane, maintained by protein complexes I-IV, to drive ATP synthesis. The electron transport chain, facilitated by these complexes, transfers electrons, generating the proton gradient essential for ATP production. The script also highlights the critical role of oxygen as the final electron acceptor, underscoring the importance of respiration for cellular energy.
Takeaways
- 🌐 All eukaryotic cells, including those in humans, contain membrane-bound organelles with specialized functions.
- 🔋 Mitochondria, with their double-membraned structure, are the primary energy suppliers for cells.
- ⚡ The inner mitochondrial membrane is crucial for energy production, acting as a barrier to maintain a proton gradient.
- 🔄 The F1F0 ATP synthase complex uses the proton gradient to synthesize ATP, providing energy for cellular reactions.
- 💡 The electron transport chain, composed of four protein complexes (I-IV), is responsible for electron transfer and proton pumping.
- 🚫 Without a proton gradient, ATP production ceases, potentially leading to cell death due to energy starvation.
- 🔬 Complex I initiates the electron transport chain by accepting high-energy electrons from NADH and pumping protons using the released energy.
- 🔄 Complex II, unlike I, does not pump protons but transfers electrons from FADH2 to coenzyme Q, promoting proton pumping in complexes III and IV.
- 🔋 Coenzyme Q acts as a mobile electron carrier, shuttling electrons from complexes I and II to complex III.
- 🌀 The final electron acceptor in the chain is oxygen, which combines with protons to form water, thus completing the electron transport and ATP synthesis cycle.
- 🔬 The inner mitochondrial membrane's dense packing of protein complexes creates an efficient cellular power plant.
Q & A
What are the primary functions of mitochondria in eukaryotic cells?
-Mitochondria are double-membraned organelles that harness most of the energy cells need for growth and reproduction, primarily through reactions taking place at the inner mitochondrial membrane.
How does the inner mitochondrial membrane contribute to energy production?
-The inner mitochondrial membrane acts as a barrier to protons, allowing a concentration gradient to be maintained, which is essential for ATP synthesis.
What is the role of the F1F0 ATP synthase in the mitochondria?
-The F1F0 ATP synthase uses the proton gradient to drive the synthesis of ATP molecules, providing energy for most of the cell's reactions.
What happens if the proton gradient is not maintained?
-If the proton gradient is not maintained, the synthase subunits stop rotating, leading to a quick energy starvation and potential cell death.
What are the four protein complexes at the heart of the electron transport chain?
-The four protein complexes at the heart of the electron transport chain are numbered I through IV, with complexes I, III, and IV directly pumping protons.
How does complex I contribute to the electron transport chain?
-Complex I receives high-energy electrons from NADH, passes them along a chain of redox centers, and uses the released energy to pump protons.
What is the role of redox centers in complex I?
-Redox centers in complex I are clusters of atoms with different electron affinities that facilitate the transfer of electrons, releasing energy used to pump protons.
How does complex II differ from complex I in its function?
-Complex II, like complex I, transfers electrons between redox centers but does not use the energy liberated to pump protons. Instead, it promotes proton pumping in complexes III and IV.
What is the significance of coenzyme Q in the electron transport chain?
-Coenzyme Q molecules receive electrons from complexes I and II and donate them to complex III, playing a crucial role in the electron transport process.
Why is oxygen essential for the electron transport chain?
-Oxygen is the final electron acceptor in the electron transport chain, and its absence halts electron transfer, stopping ATP synthesis.
How are the protein complexes of the electron transport chain spatially arranged?
-In reality, the protein complexes of the electron transport chain are densely packed, effectively making the entire surface of the inner mitochondrial membrane a cellular power plant.
Outlines
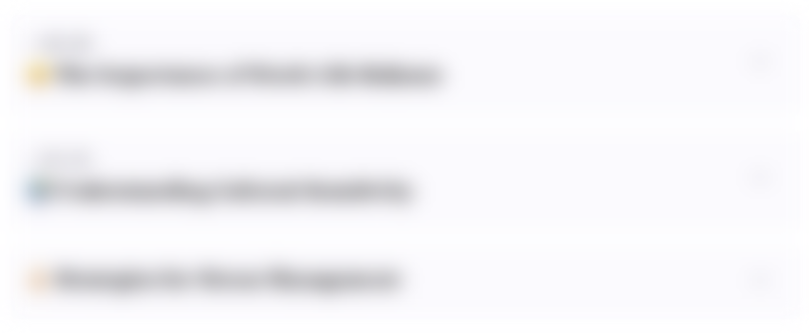
This section is available to paid users only. Please upgrade to access this part.
Upgrade NowMindmap
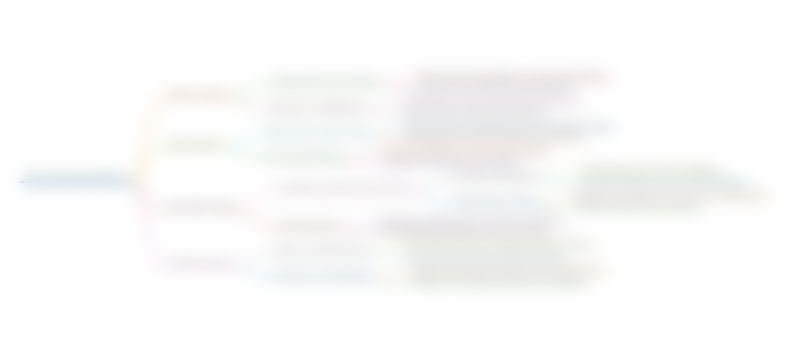
This section is available to paid users only. Please upgrade to access this part.
Upgrade NowKeywords
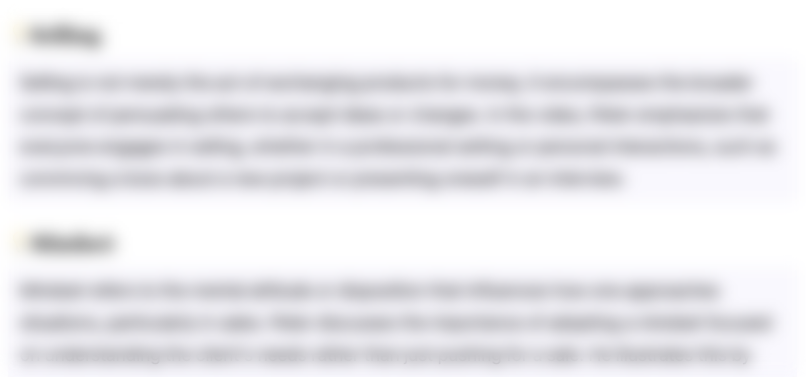
This section is available to paid users only. Please upgrade to access this part.
Upgrade NowHighlights
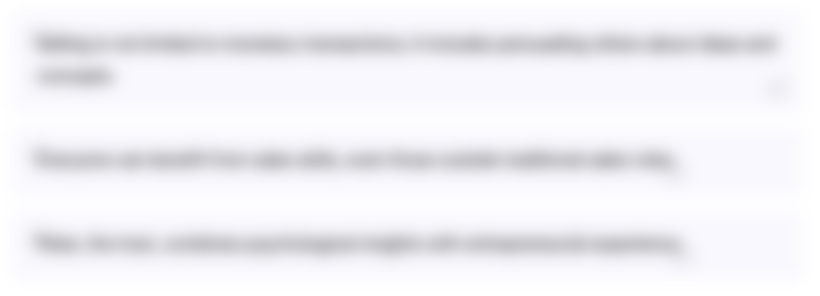
This section is available to paid users only. Please upgrade to access this part.
Upgrade NowTranscripts
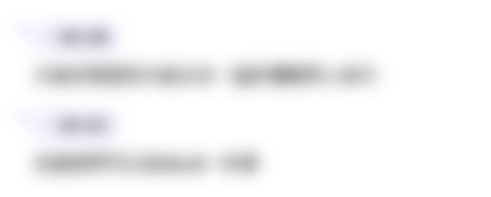
This section is available to paid users only. Please upgrade to access this part.
Upgrade Now5.0 / 5 (0 votes)