Ampere's circuital law (with examples) | Moving charges & magnetism | Physics | Khan Academy
Summary
TLDRIn this educational video, the concept of Ampere's Circuital Law is introduced as a counterpart to Gauss's Law in magnetism. The law is explained through a practical example involving three wires carrying currents. The video demonstrates how to calculate the magnetic field by taking the dot product of the magnetic field and a tiny length vector 'dl' around a closed loop. It emphasizes that the law applies only to closed loops and the result of the integral is the product of the permeability constant and the total current enclosed by the loop. The video also explains how to determine the direction of positive current using the right-hand rule and highlights the importance of attaching a surface to the loop to identify the enclosed current, a concept that becomes crucial in more complex scenarios.
Takeaways
- 🧲 Ampere's Circuital Law is the magnetic equivalent to Gauss's Law for electric fields, used to calculate magnetic fields in symmetric situations.
- 🔁 The law is applied by drawing a closed loop in space and calculating the line integral of the magnetic field (B) dot product with an infinitesimal length vector (dl) around the loop.
- ⚖️ The integral of B dot dl around a closed loop equals the product of the permeability of free space (μ₀) and the total current (I) enclosed by the loop.
- 🤔 The direction of the loop and the enclosed current can be determined using the right-hand rule, which aligns the thumb with the direction of positive current.
- 🔄 The enclosed current (I_enclosed) is defined as the total current passing through a surface attached to the loop, which can be visualized as a soap film.
- 📐 The shape of the loop and the attached surface can be any shape, including flat or blown into an open shape, but the enclosed current remains the same.
- ⚠️ Ampere's Law is only applicable for closed loops; it does not work for open paths.
- 🔗 The magnetic field considered in Ampere's Law is the total magnetic field due to all currents, both enclosed and non-enclosed by the loop.
- 🔄 The contributions of B dot dl by non-enclosed currents often cancel out, similar to how electric flux depends only on enclosed charges in Gauss's Law.
- 🔍 In complex scenarios, attaching a surface to the loop helps in determining the enclosed current, which might not be immediately obvious.
Q & A
What is Ampere's Circuital Law?
-Ampere's Circuital Law, also known as Ampere's Law, is a fundamental law of electromagnetism that relates the integrated magnetic field around a closed loop to the electric current passing through the loop. It is used to calculate the magnetic field created by electric currents.
How does Ampere's Law relate to the magnetic field and a closed loop?
-According to Ampere's Law, the closed line integral of the magnetic field (B) around a closed loop is equal to the product of the permeability of free space (μ₀) and the total current (I) enclosed by the loop.
What is the significance of the dot product in Ampere's Law?
-The dot product in Ampere's Law signifies the component of the magnetic field that is tangential to the path of integration along the closed loop. It ensures that only the magnetic field components along the direction of the loop contribute to the integral.
Why is it necessary to use a closed loop in Ampere's Law?
-A closed loop is necessary in Ampere's Law because the law is based on the concept of a circulation of the magnetic field around a current. An open path would not allow for a complete circulation, and thus, the law would not hold.
What does the enclosed current in Ampere's Law represent?
-The enclosed current in Ampere's Law represents the total current that passes through any surface bounded by the closed loop. This is determined by using the right-hand rule to determine the direction of positive current with respect to the direction of traversal around the loop.
How is the direction of the magnetic field related to the direction of the current in Ampere's Law?
-The direction of the magnetic field is related to the direction of the current through the right-hand rule. If you curl the fingers of your right hand in the direction of the current, your thumb points in the direction of the magnetic field.
What is the role of the surface attached to the loop in Ampere's Law?
-The surface attached to the loop, often imagined as a soap film, helps to visualize and calculate the enclosed current. The current that penetrates this surface is considered the enclosed current, which is used in the calculation of the magnetic field using Ampere's Law.
Can Ampere's Law be applied to non-symmetric situations?
-While Ampere's Law is particularly useful in symmetric situations due to its simplicity, it can be applied to non-symmetric situations as well. However, the calculations may be more complex and require a deeper understanding of the integration of the magnetic field around the loop.
How is Ampere's Law different from Biot-Savart Law?
-Ampere's Law is an integral law that calculates the total magnetic field around a closed loop due to all currents enclosed by the loop, while Biot-Savart Law is a differential law that calculates the magnetic field due to a small segment of current. Ampere's Law is often more convenient for symmetric situations.
Why does the magnetic field from non-enclosed currents not contribute to the integral in Ampere's Law?
-In Ampere's Law, the magnetic field contributions from non-enclosed currents tend to cancel out due to their opposite directions as you go around the loop, resulting in a net contribution of zero. This is similar to how electric flux through a Gaussian surface is contributed only by the enclosed charge.
Outlines
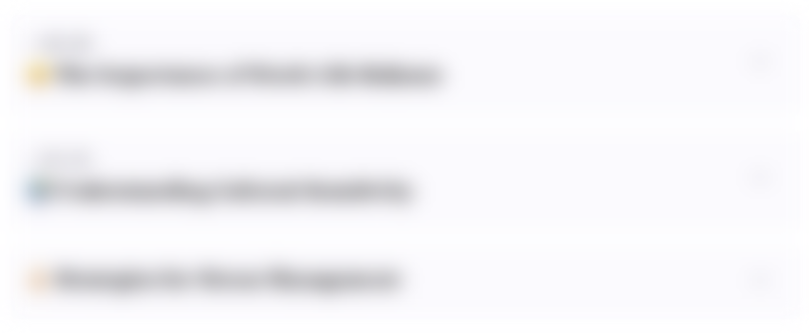
This section is available to paid users only. Please upgrade to access this part.
Upgrade NowMindmap
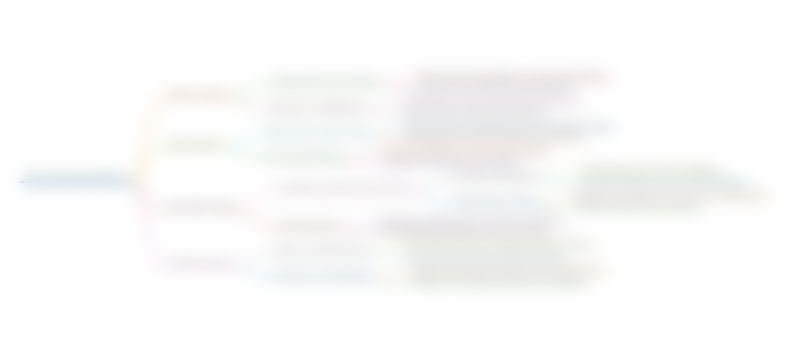
This section is available to paid users only. Please upgrade to access this part.
Upgrade NowKeywords
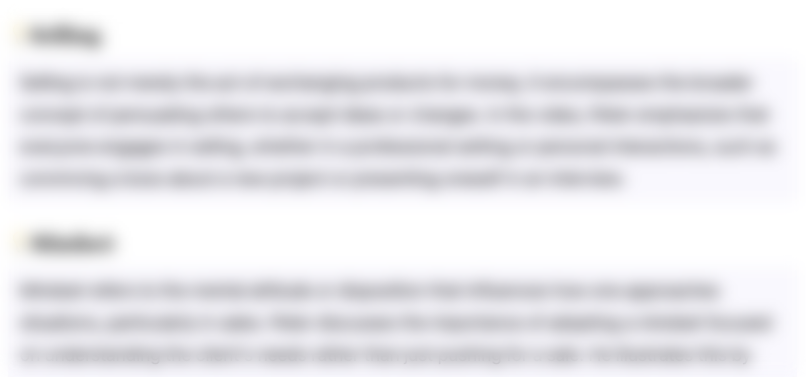
This section is available to paid users only. Please upgrade to access this part.
Upgrade NowHighlights
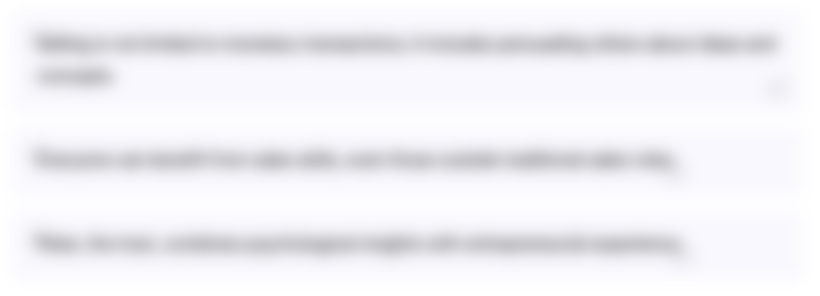
This section is available to paid users only. Please upgrade to access this part.
Upgrade NowTranscripts
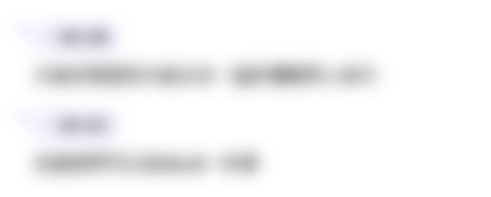
This section is available to paid users only. Please upgrade to access this part.
Upgrade NowBrowse More Related Video
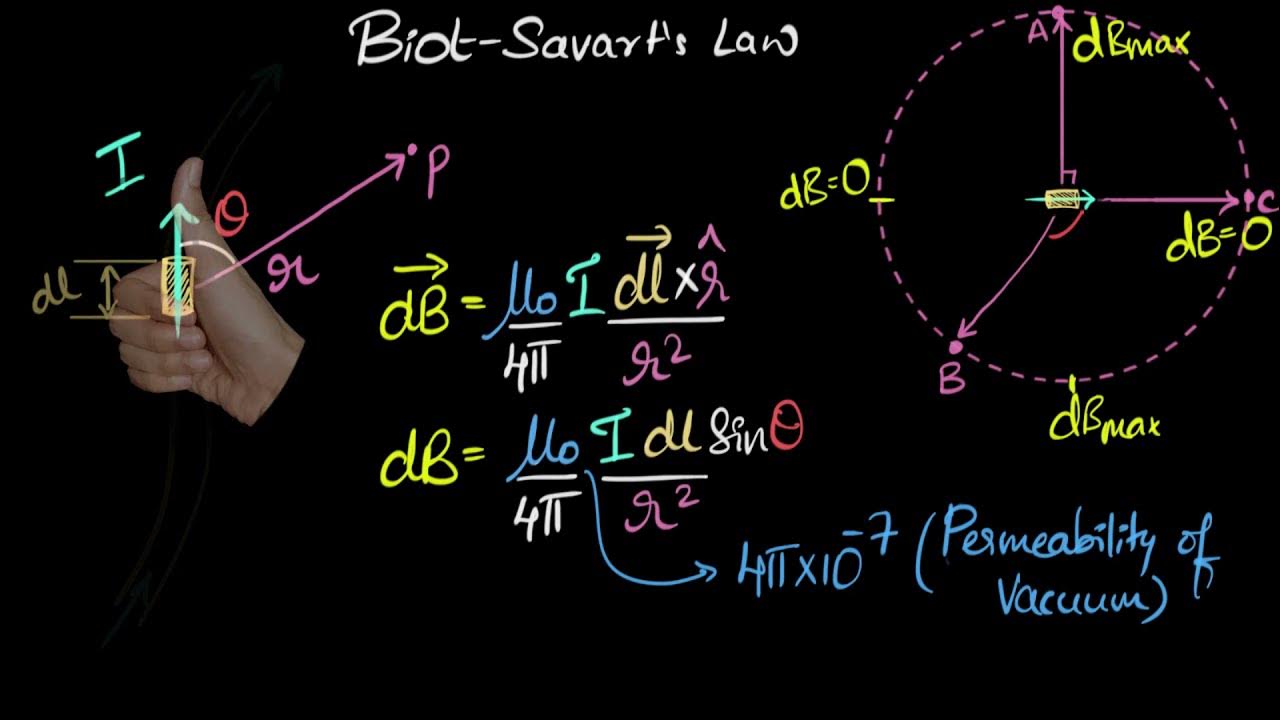
Biot Savart law (vector form) | Moving charges & magnetism | Khan Academy
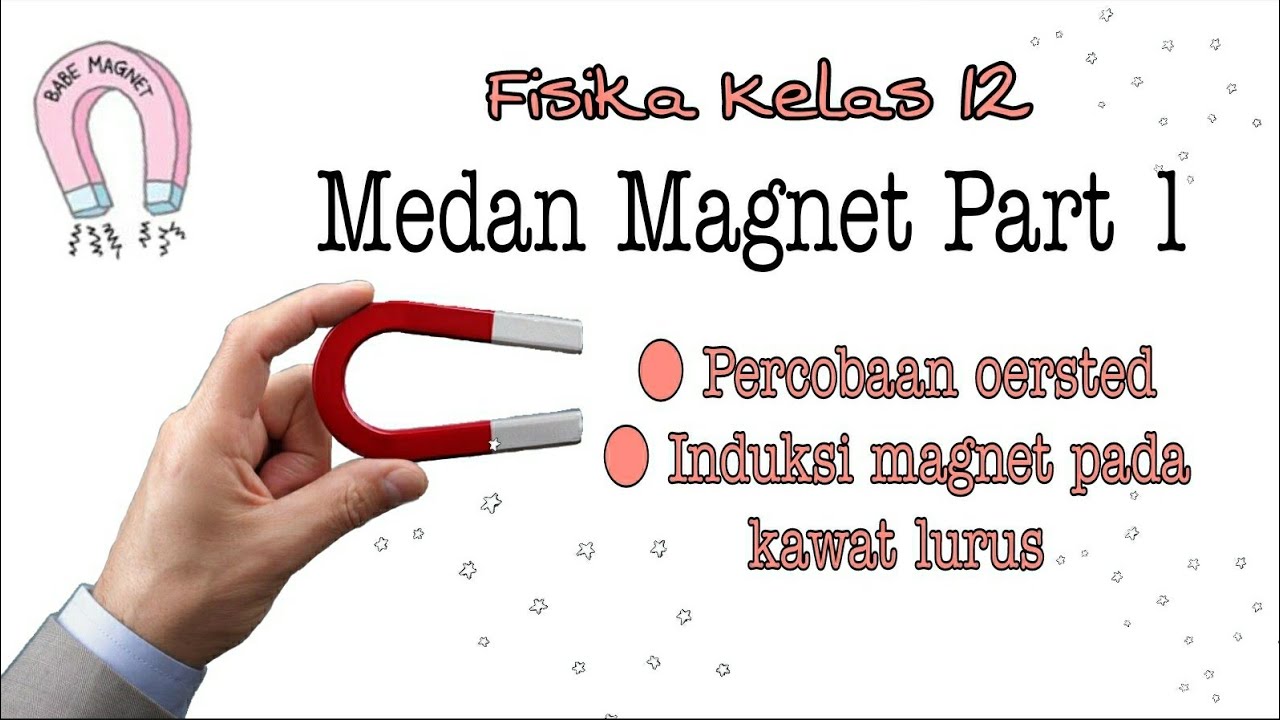
Fisika kelas 12 | Medan magnet #part1

FISIKA Kelas 12 - Gaya Magnetik | GIA Academy

GGL ( GAYA GERAK LISTRIK ) PADA KAWAT LURUS

Gaya pada Kawat Sejajar | Medan Magnet | Part 3 | Fisika Dasar
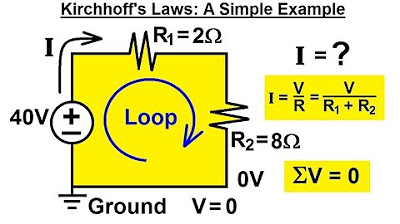
Electrical Engineering: Basic Laws (9 of 31) Kirchhoff's Laws: A Simple Example
5.0 / 5 (0 votes)