All of PARTICLES & QUANTUM in 15 mins - AS & A-level Physics
Summary
TLDRThis script offers an in-depth exploration of particle physics, focusing on fundamental particles like leptons and hadrons. It delves into quarks, their charges, and the forces governing them, including the strong and weak nuclear forces. The script also discusses the conservation laws in particle interactions and the role of various bosons. It covers concepts like charge to mass ratio, radiation types, and nuclear decay, explaining processes like alpha and beta decay. Additionally, it touches on the wave-particle duality of light and matter, the photoelectric effect, and diffraction patterns, providing a comprehensive overview of key topics in physics.
Takeaways
- π¬ Particles are categorized into hadrons (including baryons and mesons) and leptons (like electrons, muons, and neutrinos).
- π Leptons are fundamental particles with an electron number of one, while their antiparticles have an electron number of minus one.
- 𧬠Hadrons are not fundamental; baryons are made of three quarks, and mesons are made of a quark-antiquark pair.
- π Quarks come in flavors like up, down, and strange, each with specific charges and properties like strangeness.
- π₯ The electromagnetic force affects charged particles and uses the photon as its exchange particle, while gravity uses the graviton.
- π The weak nuclear force, responsible for processes like beta decay, uses W and Z bosons as exchange particles and can affect any particle.
- π The strong nuclear force, mediated by gluons, only affects hadrons and is responsible for holding atomic nuclei together.
- βοΈ Conservation laws for charge, baryon number, and lepton number are fundamental in particle interactions and decays.
- π Gamma rays are high-energy electromagnetic radiation emitted by atomic nuclei, and they can be dangerous due to their ionizing properties.
- π Radioactive decay in heavier nuclei often involves alpha decay, emitting alpha particles, while lighter nuclei may undergo beta decay, emitting beta particles.
- π The study of emission and absorption spectra helps identify elements in stars and can reveal the expansion of the universe through redshift measurements.
Q & A
What are the two main categories of particles in the Standard Model of particle physics?
-The two main categories of particles in the Standard Model are hadrons and leptons.
What are the fundamental particles known as leptons, and what are some examples?
-Leptons are fundamental particles and include the electron, muon (which is a heavy electron), and neutrinos. These particles all have an electron number of one.
What is the difference between electron neutrinos and muon neutrinos?
-Electron neutrinos and muon neutrinos are different types of neutrinos distinguished by their lepton numbers; electron neutrinos are associated with electron interactions, while muon neutrinos are associated with muon interactions.
How are hadrons different from leptons, and what are the two types of hadrons?
-Hadrons are not fundamental particles; they are composite particles made up of quarks. The two types of hadrons are baryons, which are made of three quarks, and mesons, which are made of a quark-antiquark pair.
What are the three flavors of quarks commonly dealt with in particle physics?
-The three flavors of quarks commonly dealt with are up, down, and strange quarks.
What is the charge of an up quark, and how does it compare to the charge of a down quark and a strange quark?
-An up quark has a charge of +2/3, while a down quark and a strange quark each have a charge of -1/3.
What conservation laws must be considered in particle interactions?
-In particle interactions, charge, baryon number, and lepton numbers must be conserved.
What are the exchange particles for the electromagnetic force and gravity?
-The exchange particle for the electromagnetic force is the photon, while the exchange particle for gravity is the graviton.
What are the exchange particles for the weak nuclear force?
-The exchange particles for the weak nuclear force are the W+, W-, and Z0 bosons.
How can you determine if a particle interaction is a weak interaction based on strangeness?
-Any interaction involving leptons must be a weak interaction regardless of strangeness. If an interaction only involves hadrons and strangeness is conserved, it must be a strong interaction. If strangeness isn't conserved, it must be a weak interaction.
What is the charge-to-mass ratio of a particle, and how is it calculated?
-The charge-to-mass ratio of a particle is calculated by dividing the charge in coulombs (kums) by the mass in kilograms. The unit is coulombs per kilogram.
What are the two types of radiation emitted by atomic nuclei, and how do they differ?
-The two types of radiation emitted by atomic nuclei are alpha radiation, which consists of a bundle of two protons and two neutrons, and beta radiation, which is emitted when a neutron in the nucleus turns into a proton and an electron.
What is the significance of the anti-electron neutrino in beta decay?
-The anti-electron neutrino is added in a beta minus decay equation to balance the lepton number, ensuring that the conservation laws are maintained during the decay process.
Outlines
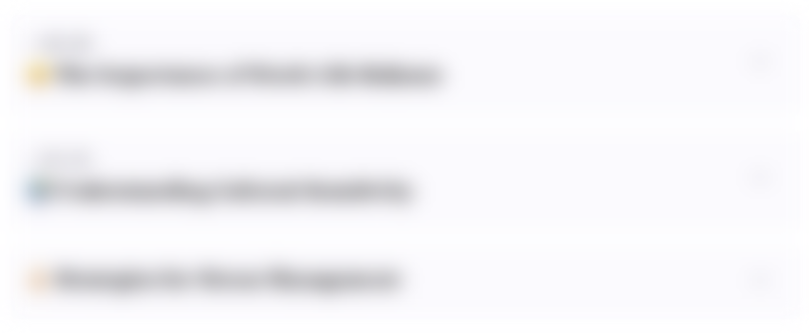
This section is available to paid users only. Please upgrade to access this part.
Upgrade NowMindmap
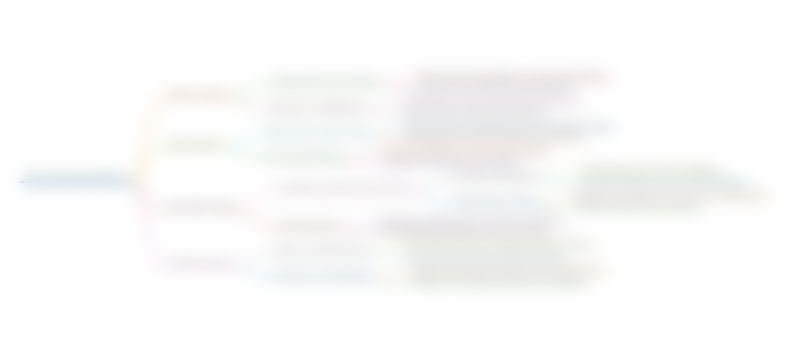
This section is available to paid users only. Please upgrade to access this part.
Upgrade NowKeywords
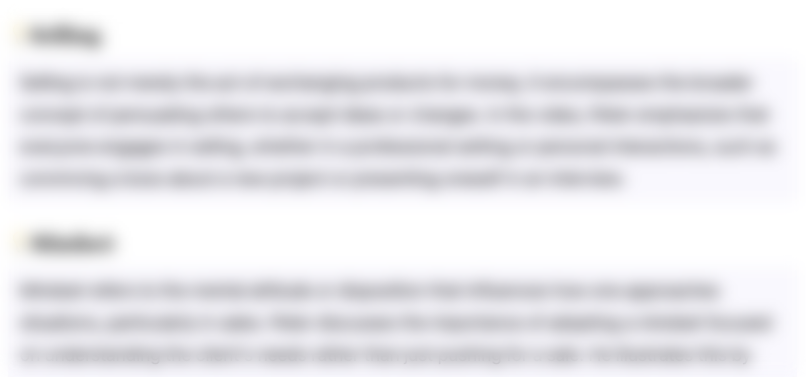
This section is available to paid users only. Please upgrade to access this part.
Upgrade NowHighlights
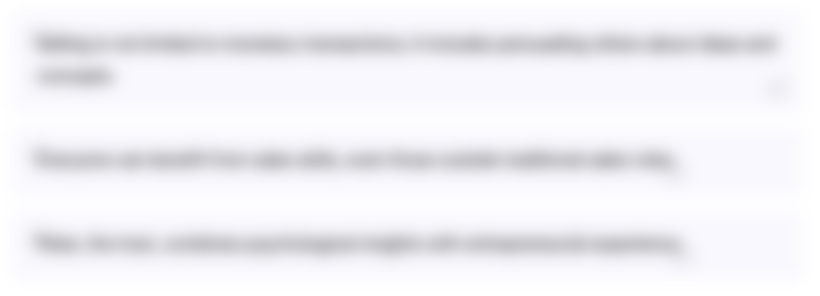
This section is available to paid users only. Please upgrade to access this part.
Upgrade NowTranscripts
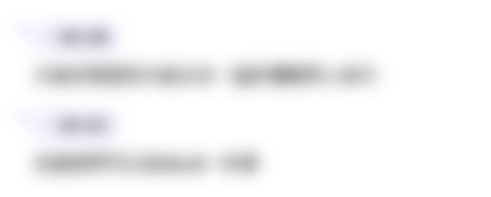
This section is available to paid users only. Please upgrade to access this part.
Upgrade NowBrowse More Related Video
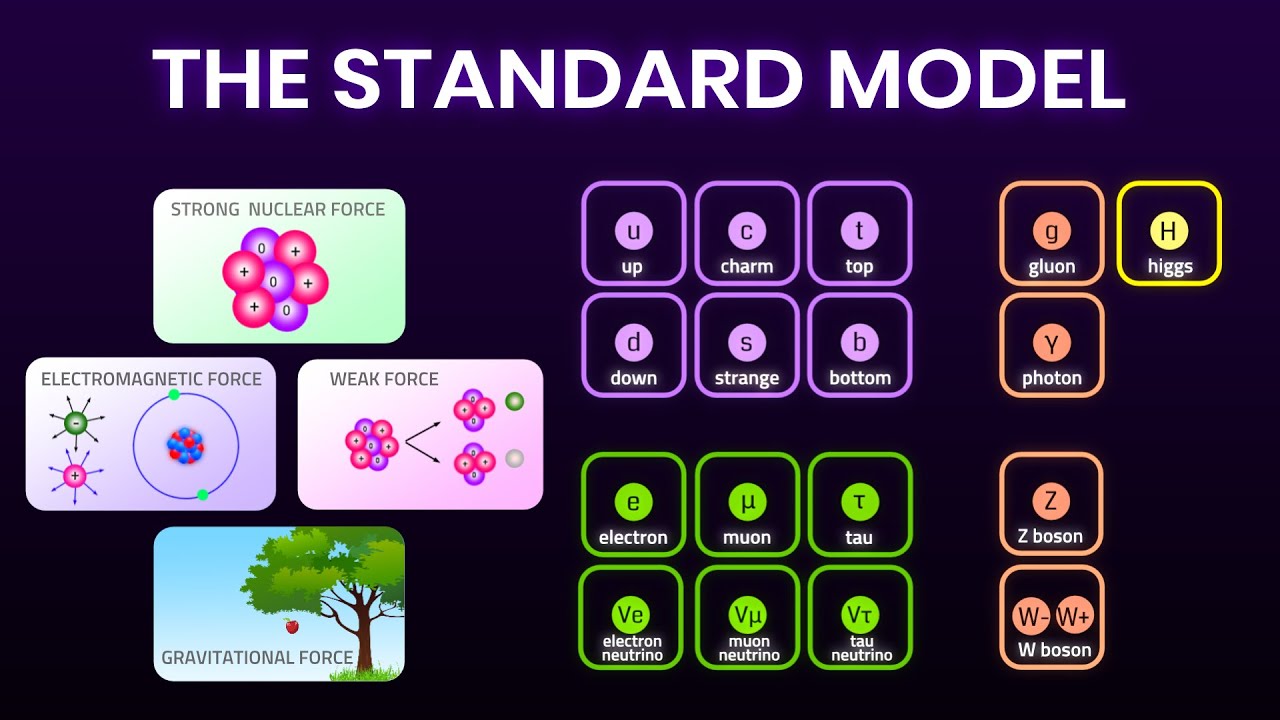
All Fundamental Forces and Particles Explained Simply | Elementary particles
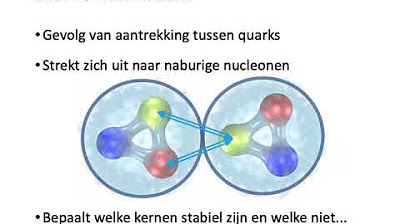
We1 sterke&zwakke ww
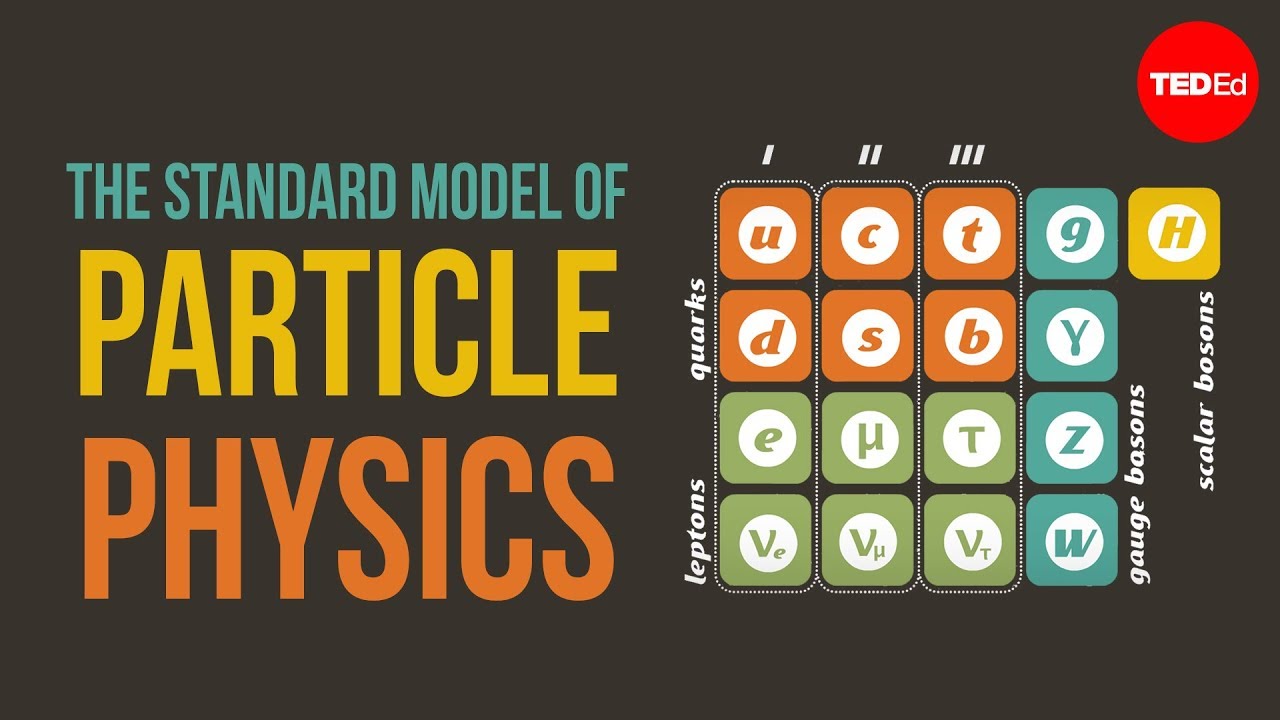
Whatβs the smallest thing in the universe? - Jonathan Butterworth
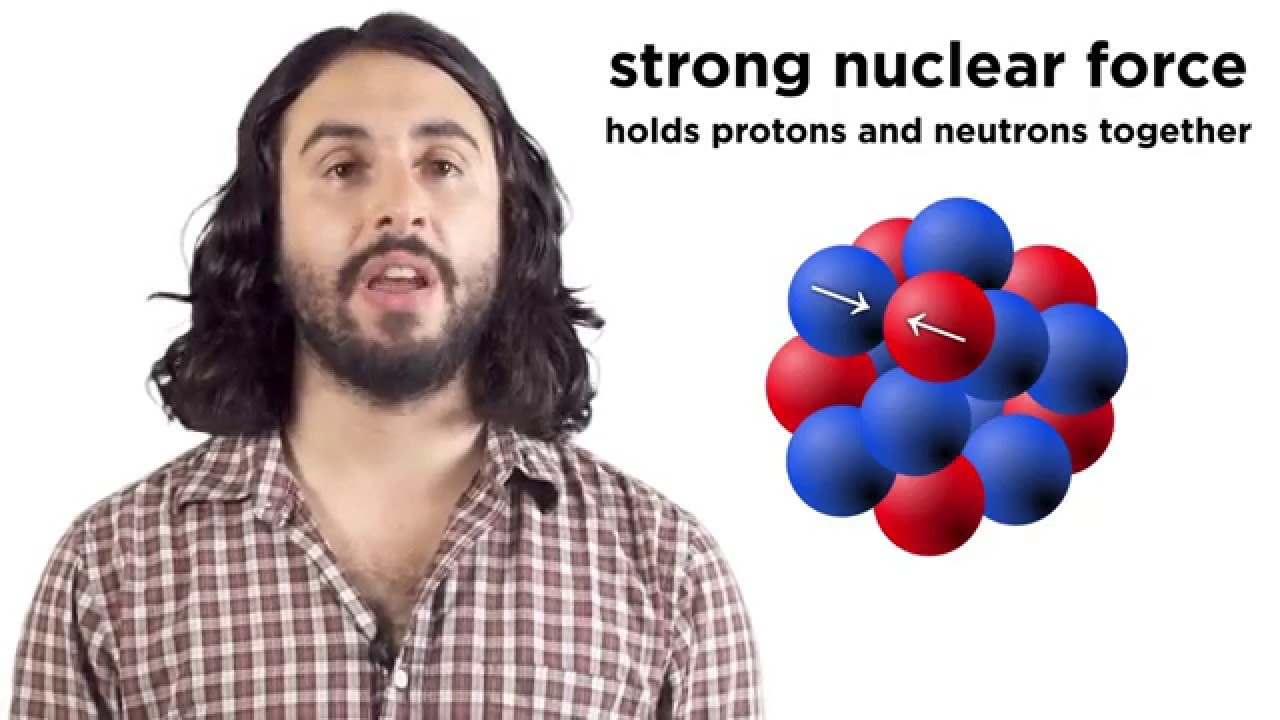
Nuclear Reactions, Radioactivity, Fission and Fusion

Kuantum 2 .1 Hipotesis de Brogglie (Apakah Partikel Mempunyai sifat Gelombang?)
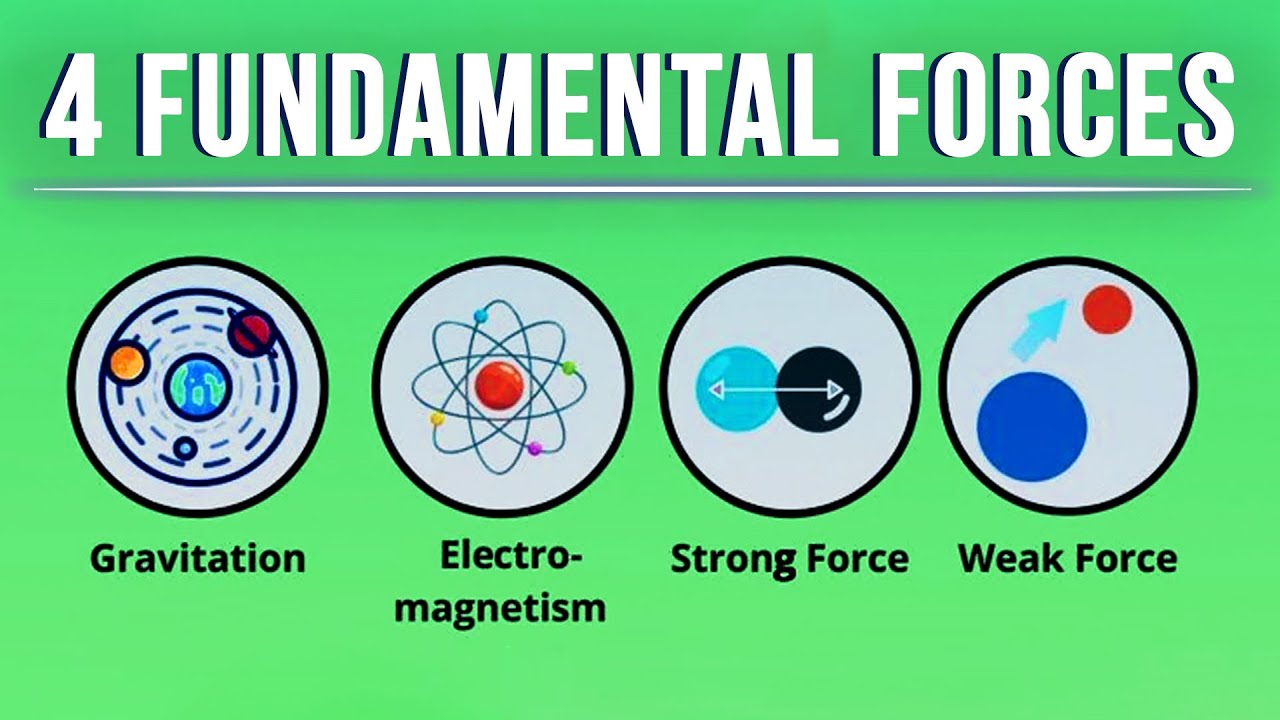
The 4 Fundamental Forces (Interactions) Of Physics Explained
5.0 / 5 (0 votes)