VSEPR Theory and Molecular Geometry
Summary
TLDRProfessor Dave's video script delves into molecular geometry, explaining how molecules arrange in 3D space due to electron cloud repulsion. The VSEPR model predicts molecular shapes based on the number of electron domains, which dictate hybridization types like sp, sp2, sp3, and sp3d. Examples like CO2, BF3, and water illustrate how electron domains, including lone pairs, influence molecular geometry, emphasizing the importance of understanding these shapes for chemical behavior.
Takeaways
- 🌐 Molecular geometry is crucial for understanding how molecules interact in 3D space and their chemical behavior.
- 🔍 The VSEPR model (Valence Shell Electron Pair Repulsion) is used to predict molecular shapes based on electron repulsion.
- 🌀 Electron clouds around atoms repel each other, causing molecules to arrange to minimize potential energy.
- 🔬 Carbon dioxide (CO2) is an example of a linear molecule with sp hybridization and a 180-degree bond angle due to two electron domains.
- 📐 BF3 (boron trifluoride) has a trigonal planar shape with sp2 hybridization and 120-degree bond angles, resulting from three electron domains.
- 🔮 Methane (CH4) demonstrates tetrahedral electron domain geometry with sp3 hybridization and 109.5-degree bond angles from four electron domains.
- 🧬 Five electron domains lead to trigonal bipyramidal geometry with sp3d hybridization, featuring both 90 and 120-degree bond angles.
- 🔢 Six electron domains result in octahedral geometry with sp3d2 hybridization, characterized by 90-degree bond angles.
- 👁 Lone pairs on atoms also count as electron domains and can affect the molecular geometry, as seen in ammonia and water.
- 📚 The number of electron domains dictates the hybridization and geometry of a molecule, which can vary with the presence of lone pairs.
- 📝 To determine molecular geometry, start with the Lewis dot structure, count electron domains, and match them to the corresponding hybridization and geometry.
Q & A
What is the significance of understanding molecular geometry in chemistry?
-Understanding molecular geometry is crucial because it affects how molecules interact and participate in chemical reactions, influencing their reactivity and properties.
What does the acronym VSEPR stand for, and what is its purpose in chemistry?
-VSEPR stands for Valence Shell Electron Pair Repulsion. It is a model used to predict the shape of a molecule based on the repulsion between electron pairs in the valence shell of its atoms.
Why do electron clouds in a molecule repel each other?
-Electron clouds repel each other because they are negatively charged, and like charges naturally repel each other in accordance with Coulomb's law.
How does the VSEPR model predict the shape of a molecule like carbon dioxide (CO2)?
-In CO2, the carbon atom has two electron domains, leading to sp hybridization and a linear electron domain geometry with a 180-degree bond angle between the oxygen atoms.
What type of hybridization and molecular geometry does BF3 exhibit, and why?
-BF3 exhibits sp2 hybridization and trigonal planar electron domain geometry due to the three electron domains surrounding the boron atom, resulting in 120-degree bond angles between the fluorine atoms.
How does the presence of lone pairs affect the molecular geometry compared to a molecule with only covalent bonds?
-Lone pairs take up less space than covalent bonds, which can alter the molecular geometry. For example, ammonia has a trigonal pyramidal geometry due to its lone pair, while methane, with a similar tetrahedral electron domain geometry, has a different shape.
What is the hybridization and molecular geometry of a water molecule?
-A water molecule has sp3 hybridization due to the oxygen atom's two bonds and two lone pairs. However, its molecular geometry is bent, not tetrahedral, because the lone pairs exert less repulsion than the bonding pairs.
How do the electron domains in a molecule determine its hybridization and geometry?
-The number of electron domains (both lone pairs and covalent bonds) dictates the hybridization of the central atom, which in turn correlates with a specific electron domain geometry. This geometry can vary with the presence of lone pairs.
What is the difference between electron domain geometry and molecular geometry?
-Electron domain geometry refers to the arrangement of all electron domains around a central atom, while molecular geometry specifically refers to the shape of the molecule, taking into account the space occupied by lone pairs versus bonding pairs.
How does the VSEPR model account for the three-dimensional arrangement of atoms in molecules with four electron domains?
-For molecules with four electron domains, such as methane, the model predicts sp3 hybridization and a tetrahedral electron domain geometry, which is the most stable arrangement in three dimensions to minimize electron repulsion.
What are the key steps in using the VSEPR model to determine the geometry of a molecule?
-The key steps are: 1) Draw the correct Lewis dot structure of the molecule, 2) Count the total number of electron domains (including lone pairs), 3) Determine the hybridization based on the number of electron domains, and 4) Predict the electron domain geometry and adjust for molecular geometry if there are lone pairs.
Outlines
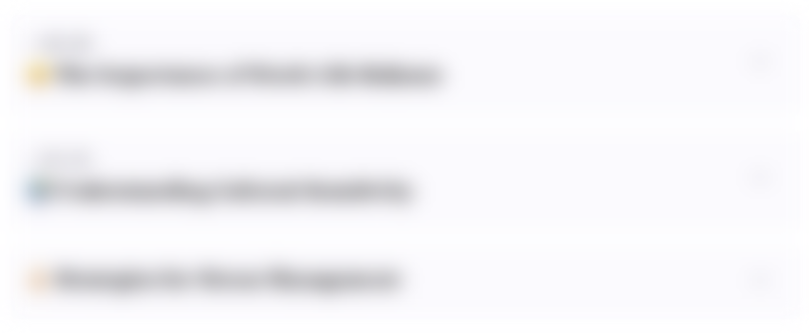
Этот раздел доступен только подписчикам платных тарифов. Пожалуйста, перейдите на платный тариф для доступа.
Перейти на платный тарифMindmap
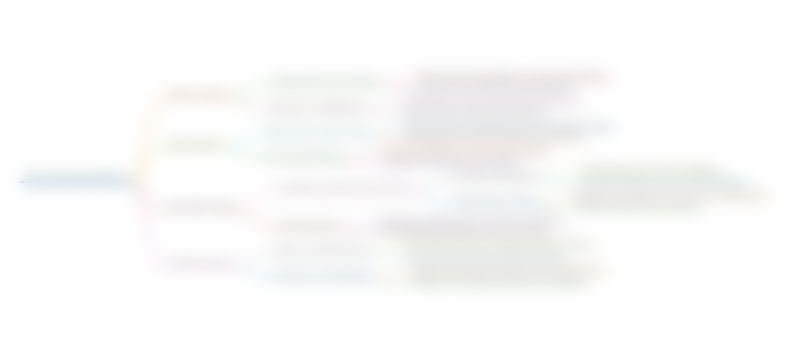
Этот раздел доступен только подписчикам платных тарифов. Пожалуйста, перейдите на платный тариф для доступа.
Перейти на платный тарифKeywords
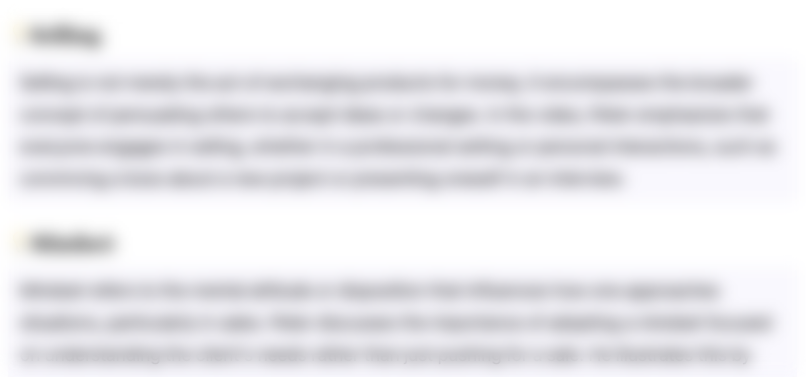
Этот раздел доступен только подписчикам платных тарифов. Пожалуйста, перейдите на платный тариф для доступа.
Перейти на платный тарифHighlights
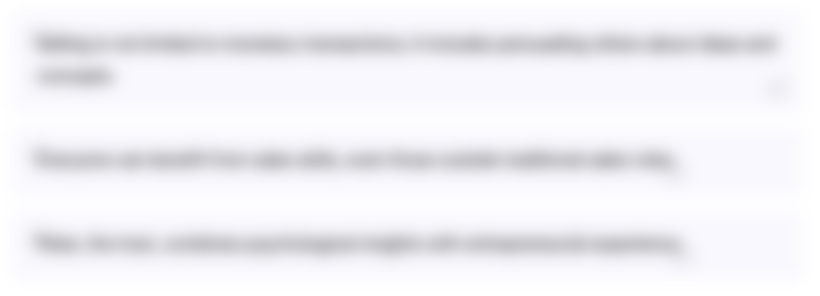
Этот раздел доступен только подписчикам платных тарифов. Пожалуйста, перейдите на платный тариф для доступа.
Перейти на платный тарифTranscripts
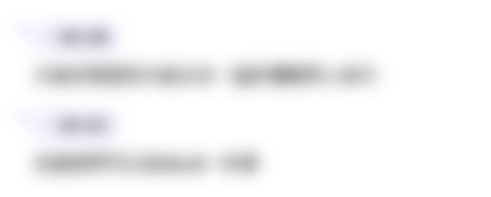
Этот раздел доступен только подписчикам платных тарифов. Пожалуйста, перейдите на платный тариф для доступа.
Перейти на платный тарифПосмотреть больше похожих видео
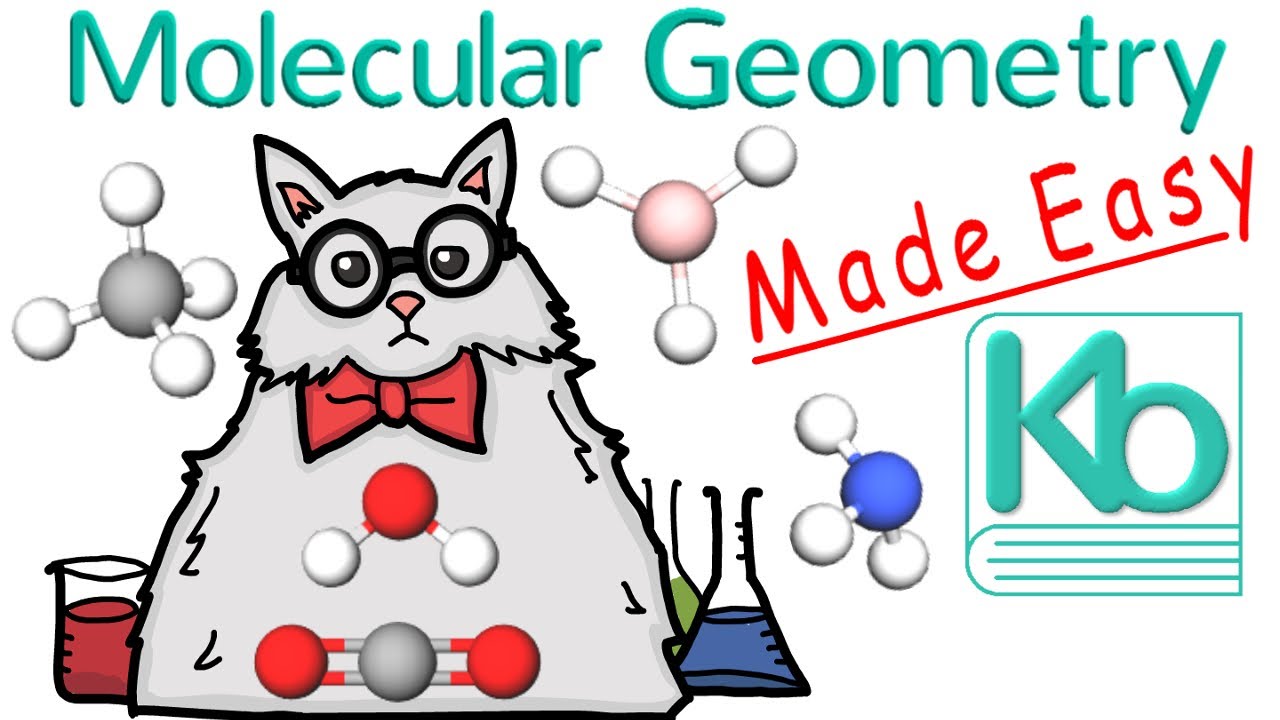
Molecular Geometry Made Easy: VSEPR Theory and How to Determine the Shape of a Molecule
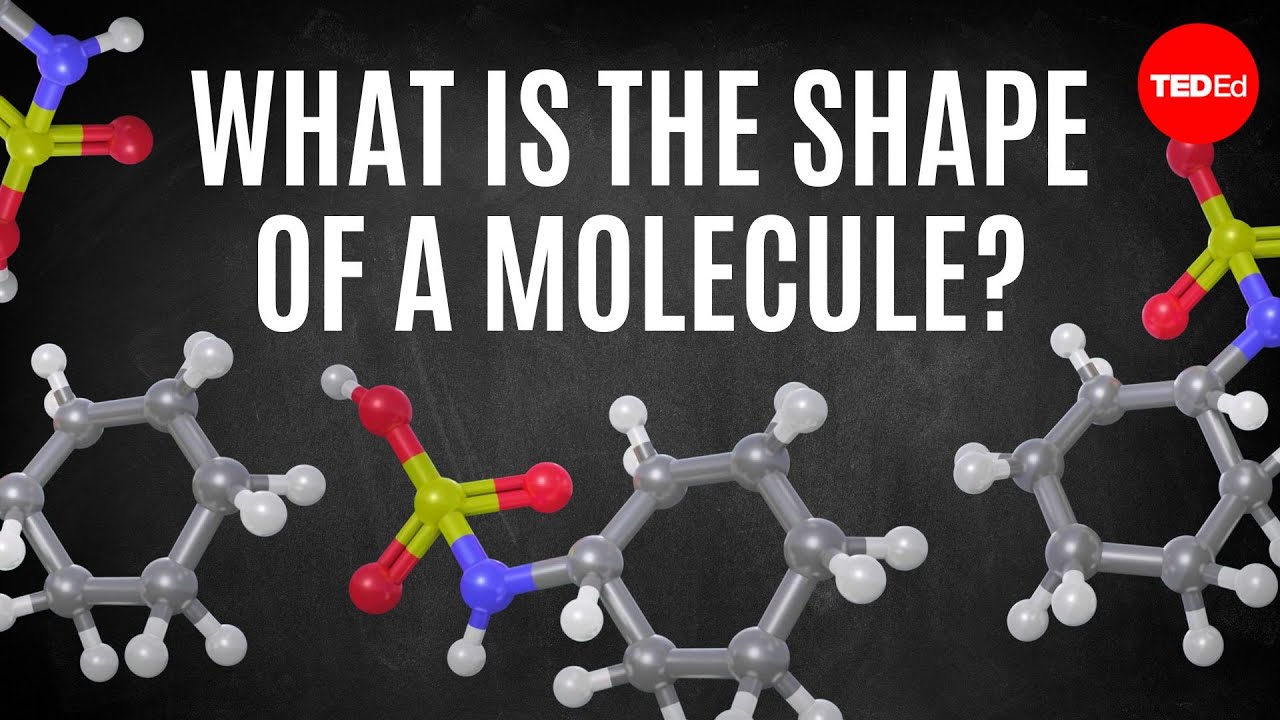
What is the shape of a molecule? - George Zaidan and Charles Morton

Chem Ch 6 Section 6.5: VSEPR Theory (part 1)
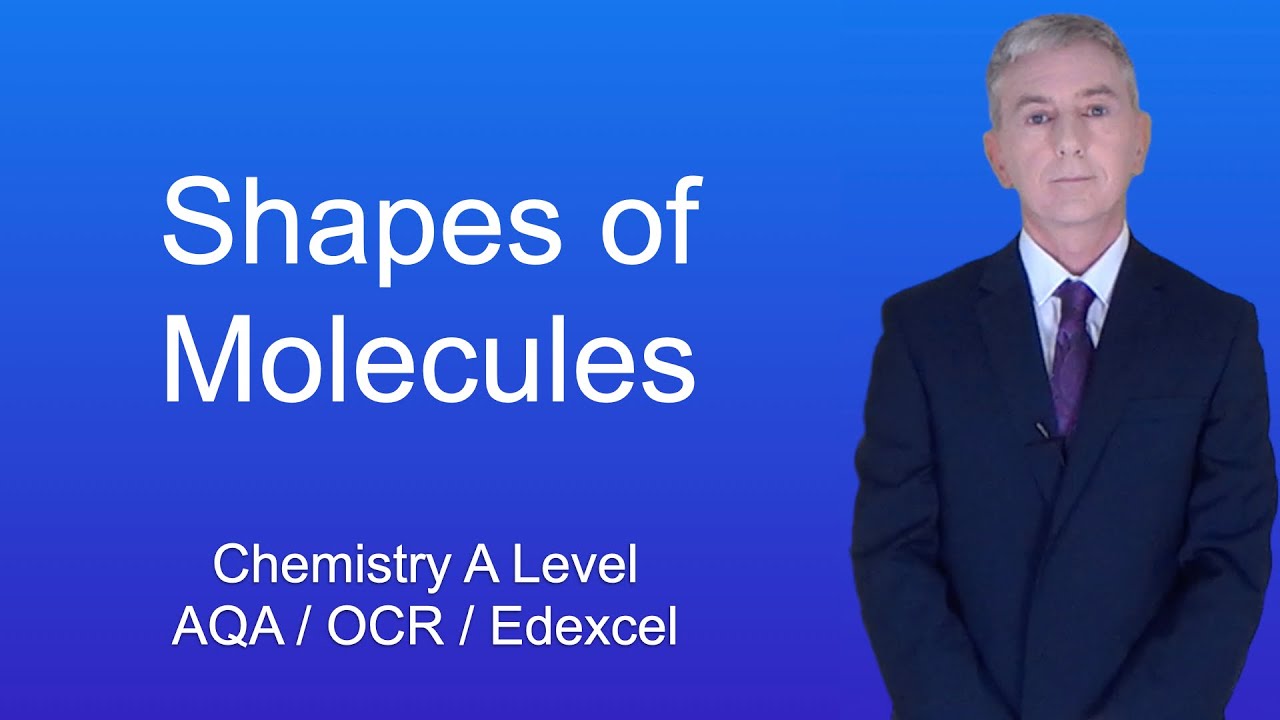
A Level Chemistry Revision "Shapes of Molecules".
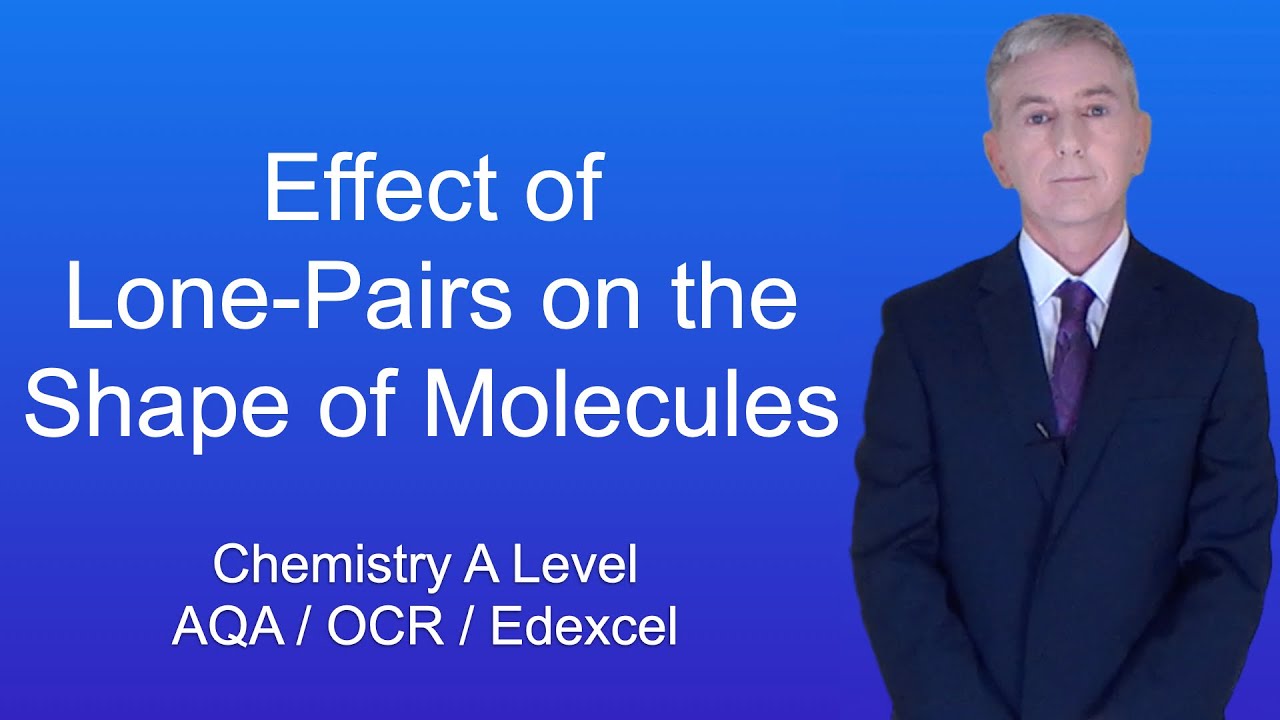
A Level Chemistry Revision "Effect of Lone Pairs on the Shape of Molecules".

geometri molekul bagian 3
5.0 / 5 (0 votes)