SHM - Simple Harmonic Motion - Physics A-level Required Practical
Summary
TLDRIn this MSB science video, Mr. Reese demonstrates the practical steps for conducting A-level physics experiments on simple harmonic motion using a simple pendulum and a mass-spring system. He explains how to measure the time period of oscillations by changing the length of the pendulum string and the mass on the spring, ensuring accuracy with a ruler and a fiducial marker. The video guides viewers through the process of collecting data, calculating the time period for different lengths and masses, and plotting graphs to verify the relationship between time period and the physical properties of the systems.
Takeaways
- 🔍 Mr. Reese from MSB Science is demonstrating A-Level physics experiments on simple harmonic motion.
- 📏 For the pendulum experiment, the length of the string is varied to measure the period of oscillations.
- 📐 A high-resolution ruler is used to ensure accurate measurements, with measurements taken from the bottom of the wood to the center of the pendulum bob.
- 🔩 The string is clamped between pieces of wood to ensure the pivot point is fixed at the bottom.
- 🧷 A nail is used as a fiducial marker to track the pendulum's motion without touching it.
- 🔄 The pendulum is displaced about 10° to ensure accuracy, as larger amplitudes reduce the accuracy of the experiment.
- ⏱️ Time is measured for 10 oscillations and then averaged to find the period of one oscillation.
- 📉 The relationship between the square of the period (T^2) and the length of the string is graphed to find the gradient, which is proportional to the acceleration due to gravity (g).
- 🔗 The mass-spring system experiment is similar to the pendulum, using a spring and a clamp to create oscillation.
- 📊 The graph of T^2 against mass (m) should be a straight line, indicating that T^2 is proportional to m, allowing for the calculation of the spring constant (K).
- 🔍 The Hooke's Law experiment can be conducted to find the spring constant (K) and verify the results obtained from the mass-spring system.
Q & A
What is the purpose of the practical demonstrated in the script?
-The purpose of the practical is to study simple harmonic motion using a simple pendulum and a mass-spring system, by measuring the time period of oscillations for different lengths of the pendulum string and different masses on the spring.
Why is it important to ensure the pivot is at the bottom of the pieces of wood when setting up the pendulum?
-Ensuring the pivot is at the bottom of the pieces of wood is important to guarantee that the pivot is fixed at the bottom, which is necessary for accurate measurements of the pendulum's length.
What is the significance of using a ruler with 1 mm resolution in this experiment?
-A ruler with 1 mm resolution is used for more accurate measurements of the pendulum's length, which is crucial for the precision of the experiment.
Why is it necessary to measure from the bottom of the wood to the middle of the bob?
-Measuring from the bottom of the wood to the middle of the bob ensures that the measurement is taken to the center of mass, not to the top or bottom of the bob, which would introduce errors.
What is the role of the nail as a fiducial marker in the experiment?
-The nail serves as a fiducial marker to provide a reference point for the pendulum's position, allowing for accurate timing of the oscillations.
Why is it advised not to displace the pendulum too far from equilibrium?
-Displacing the pendulum too far from equilibrium can lead to a larger amplitude, which makes the equation used for calculating the time period less accurate.
How does measuring the time for 10 oscillations instead of one improve accuracy?
-Measuring the time for 10 oscillations and then averaging it reduces random errors and provides a more accurate estimate of the time period for one oscillation.
What is the equation relating the time period (T) to the length (L) of the pendulum string?
-The equation relating the time period (T) to the length (L) of the pendulum string is T = 2π√(L/g), where g is the acceleration due to gravity.
Why is it necessary to square the time period when plotting the graph for the pendulum?
-Squaring the time period allows for a linear relationship between T^2 and L, which is easier to analyze and graph, as T^2 is proportional to L.
How does changing the mass on the spring affect the time period of oscillation?
-The time period of oscillation for a mass-spring system is given by T = 2π√(m/k), where m is the mass and k is the spring constant. Changing the mass will affect the time period according to this relationship.
What is the significance of plotting T^2 against M for the mass-spring system?
-Plotting T^2 against M for the mass-spring system allows for the determination of the spring constant (k) by analyzing the gradient of the resulting straight line graph.
Outlines
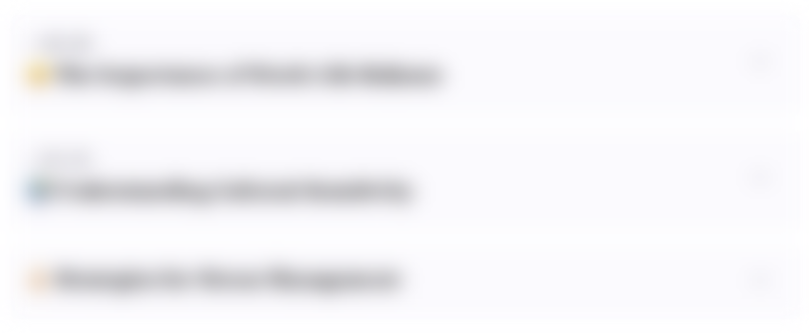
Этот раздел доступен только подписчикам платных тарифов. Пожалуйста, перейдите на платный тариф для доступа.
Перейти на платный тарифMindmap
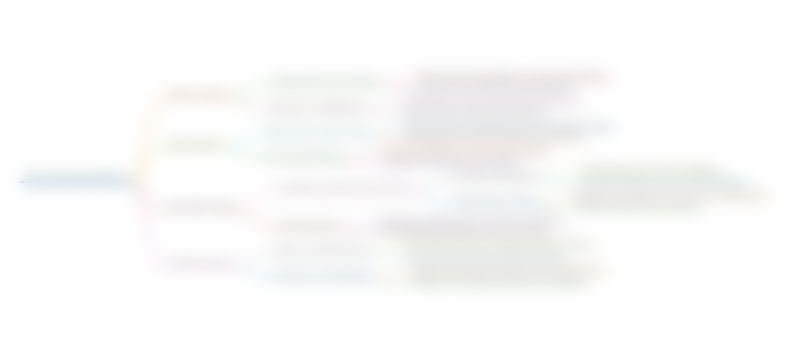
Этот раздел доступен только подписчикам платных тарифов. Пожалуйста, перейдите на платный тариф для доступа.
Перейти на платный тарифKeywords
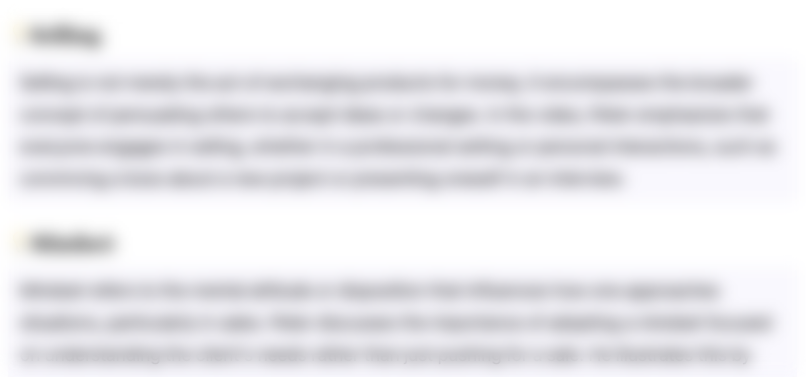
Этот раздел доступен только подписчикам платных тарифов. Пожалуйста, перейдите на платный тариф для доступа.
Перейти на платный тарифHighlights
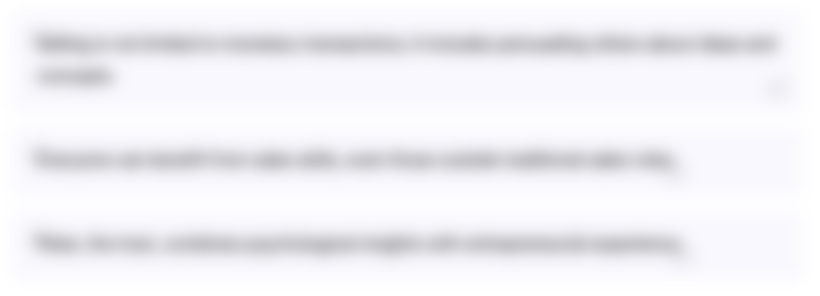
Этот раздел доступен только подписчикам платных тарифов. Пожалуйста, перейдите на платный тариф для доступа.
Перейти на платный тарифTranscripts
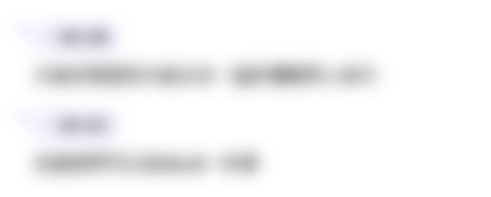
Этот раздел доступен только подписчикам платных тарифов. Пожалуйста, перейдите на платный тариф для доступа.
Перейти на платный тариф5.0 / 5 (0 votes)