How lasers work
Summary
TLDRThis video script explores the principles of lasers, comparing electron orbits to planetary motion and delving into quantum mechanics. It explains how electrons occupy quantized energy levels and how lasers use stimulated emission to produce coherent light. The script covers various types of lasers, including gas, solid-state, semiconductor, and free-electron lasers, and discusses techniques like population inversion and Q-switching. The goal is to provide an engaging and informative overview of laser technology and its applications.
Takeaways
- 📚 Electrons orbiting an atom are described by quantum mechanics, with specific quantized orbits unlike the continuous orbits of planets around the sun.
- 🌌 The energy levels of electrons are fixed and can only take precise quantized values, with lower energy states closer to the nucleus on average.
- 🔄 The repulsive force between multiple electrons in an atom is strong, affecting the energy levels and complicating calculations compared to a single electron.
- 💡 An electron can be excited to a higher energy level by collision or by absorbing a photon with energy matching the energy gap between levels.
- 🕊 Spontaneous Emission is the process where an excited electron emits a photon after a random period, while Stimulated Emission is the synchronized emission of a new photon caused by an existing photon.
- 🚀 A laser operates on the principle of Stimulated Emission, where a chain reaction of photon emissions is initiated by a single photon, resulting in a coherent beam.
- 🌟 The Gain Medium in a laser is the material with atoms capable of emitting light through the process of Stimulated Emission, and it can be in various states of matter.
- 🔍 To produce a laser beam, the Gain Medium is often shaped into a cylinder to favor photons traveling the long axis, which can then be amplified by mirrors at either end.
- 🔄 Population Inversion is a crucial condition for lasing, where more atoms are in the excited state than the lower energy state, achieved through the process of 'pumping'.
- 🔧 Lasers can be Continuous Wave, maintaining a steady output as long as energy is supplied for pumping, or they can use techniques like Q-switching to produce powerful, short pulses.
- 🛠️ Various types of lasers exist, including solid-state, gas, semiconductor, and free-electron lasers, each with unique methods of pumping and applications.
Q & A
How does the electron orbital model in an atom differ from the planetary model of the solar system?
-Electrons in an atom are governed by quantum mechanics and can only occupy specific quantized orbits or quantum states, unlike planets which can orbit at any distance from the sun.
What is the significance of the term 'quantum states' in the context of electron orbits?
-Quantum states refer to the specific, discrete energy levels that an electron can occupy around an atomic nucleus, as opposed to the continuous range of orbits available to planets around the sun.
Why is it necessary for a photon to have nearly the exact energy as the gap between electron levels to excite an electron?
-The energy of the photon must match the energy difference between the electron levels to provide the necessary energy for the electron to transition to a higher state, which is a requirement for excitation.
What is the concept of 'population inversion' in lasers?
-Population inversion is a condition where there are more atoms in the higher energy state (upper laser level) than in the lower energy state, which is necessary for the amplification of light in a laser.
How does the mutual repulsion between electrons in an atom affect the energy levels?
-The repulsive force between multiple electrons in an atom is quite strong, which changes the energy levels due to their mutual repulsion and makes it more difficult to calculate them compared to a single electron.
What is the role of the 'gain medium' in a laser?
-The gain medium is the material that contains the atoms capable of producing the laser light through the process of stimulated emission of radiation. It is where the energy transfer and photon amplification occur.
How does a laser differ from a simple light source like a lamp?
-A laser produces a coherent beam of light with a single wavelength and direction due to the process of stimulated emission, whereas a lamp emits incoherent light with photons emitted in random directions and wavelengths.
What is the purpose of the mirrors at the ends of a laser's gain medium?
-The mirrors are used to reflect the light back and forth through the gain medium, allowing the light to interact with the excited atoms multiple times and amplifying the light through stimulated emission.
What is the process called 'pumping' in the context of lasers?
-Pumping is the process of energizing atoms into an excited state. It is necessary to continuously supply energy to the gain medium to replace the atoms that have emitted photons and returned to a lower energy state.
What is the difference between a continuous wave (CW) laser and a Q-switched laser?
-A continuous wave laser operates steadily as long as energy is provided for the pumping, emitting a constant beam of light. A Q-switched laser, on the other hand, temporarily blocks the laser beam to build up a large population of excited atoms and then releases a powerful, short pulse of light when the block is removed.
How do semiconductor or diode lasers produce light?
-Semiconductor lasers produce light through the recombination of electrons and holes at a junction between P-type and N-type materials. When a voltage is applied, the recombination process can be stimulated by existing photons, leading to the emission of new photons and the creation of a laser beam.
Outlines
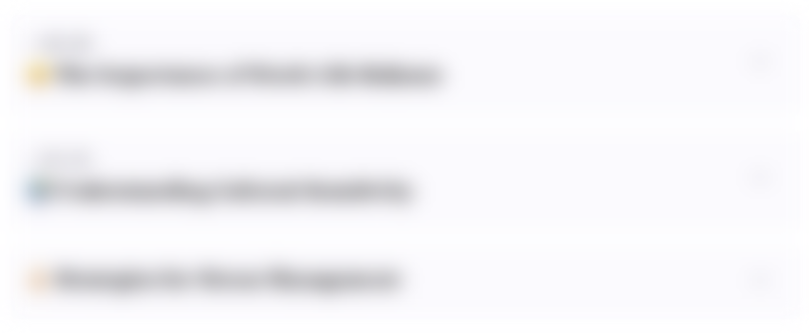
このセクションは有料ユーザー限定です。 アクセスするには、アップグレードをお願いします。
今すぐアップグレードMindmap
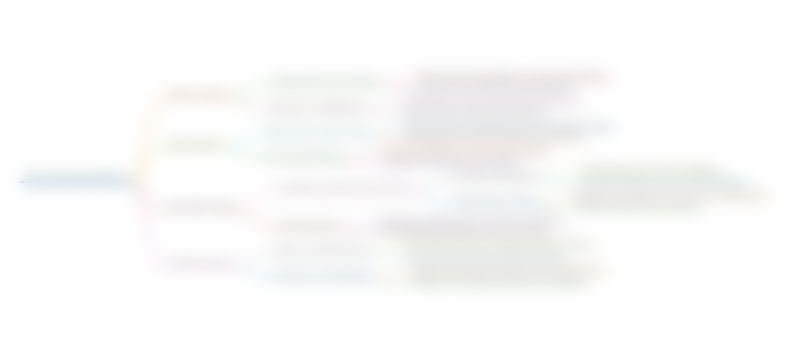
このセクションは有料ユーザー限定です。 アクセスするには、アップグレードをお願いします。
今すぐアップグレードKeywords
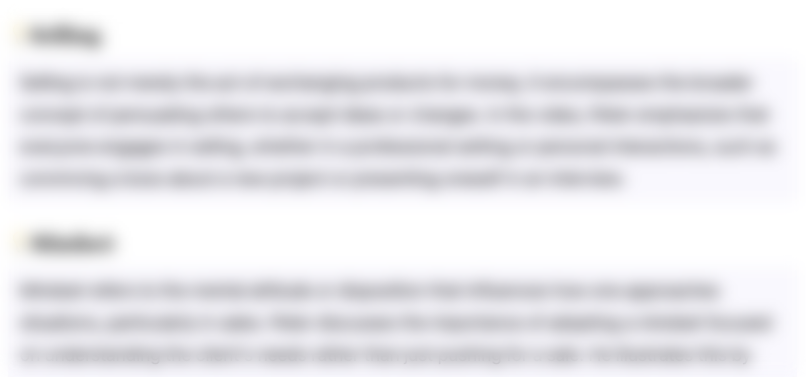
このセクションは有料ユーザー限定です。 アクセスするには、アップグレードをお願いします。
今すぐアップグレードHighlights
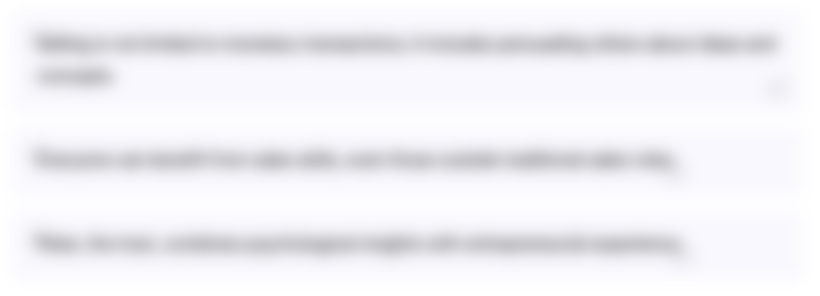
このセクションは有料ユーザー限定です。 アクセスするには、アップグレードをお願いします。
今すぐアップグレードTranscripts
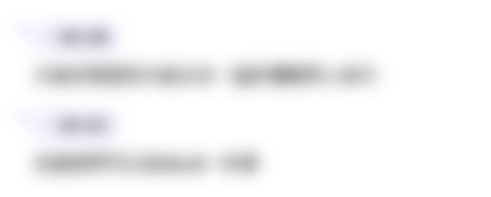
このセクションは有料ユーザー限定です。 アクセスするには、アップグレードをお願いします。
今すぐアップグレード5.0 / 5 (0 votes)