ATPL Principles of Flight - Class 17: Stability II.
Summary
TLDRIn this educational video, Grant explores the principles of aircraft stability, focusing on the three axes: longitudinal, lateral, and directional. He explains how disturbances are corrected through the aircraft's design, such as the tail plane's role in pitch stability and the fin's influence on yaw. The video delves into static and dynamic stability, illustrating how these factors affect flight and can lead to issues like pilot-induced oscillations and Dutch roll. Grant also touches on how stability can vary with speed, center of gravity, and tail plane area, providing a comprehensive look at the aerodynamic forces that keep planes stable in the sky.
Takeaways
- 🛫 Static longitudinal stability is crucial for an aircraft's initial response to pitch disturbances, with the tailplane playing a key role in creating a corrective nose-up pitching moment.
- ⚖️ The restoring moment's strength in longitudinal stability is influenced by the length of the balance arm, which can be optimized by positioning the center of gravity further forward.
- 📈 The coefficient of moment is a useful tool for assessing an aircraft's longitudinal stability, with positive values indicating nose-up and negative values indicating nose-down tendencies.
- 📊 A graph of the coefficient of pitching moment versus angle of attack helps visualize an aircraft's static stability, with different line slopes indicating varying stability levels.
- 🔁 Dynamic stability in pitch can manifest as short period oscillations, which are rapid and can be dangerous, or as long period oscillations known as phugoid oscillations, which are slower and easier to correct.
- 🔄 Pilot-induced oscillation occurs when a pilot's corrective actions are out of sync with short period oscillations, exacerbating the problem.
- 🧭 Directional stability, or yaw stability, is maintained by the vertical stabilizer and is essential for consistent heading, with the force and distance involved in the yawing moment being critical.
- 📉 The coefficient of yawing moment is simplified for understanding by considering dynamic pressure, fin area, and wingspan, and is represented graphically against the angle of sideslip.
- 🤸♂️ Lateral static stability ensures an aircraft returns to level flight after a roll disturbance, with the lift difference between the wings creating a corrective rolling moment.
- 🔗 Roll and yaw are interconnected; uncoordinated rolling motions can lead to slipping or skidding, affecting the side slip angle and overall stability.
- 🔄 Dutch roll and spiral instability are stability issues that arise from imbalances between directional and lateral stability, leading to wobbling or spiraling flight paths.
Q & A
What is static longitudinal stability in aviation?
-Static longitudinal stability refers to the initial behavior of an aircraft in response to disturbances in pitch. It ensures that any disturbances cause a pitching moment that corrects the problem, such as using the tail plane to create a nose-up pitching moment to counteract a nose-down disturbance.
How does the position of the center of gravity affect stability?
-A further forward center of gravity increases the balance arm, which in turn strengthens the restoring moments, enhancing both longitudinal and directional stability.
What is the role of the tail plane in maintaining stability?
-The tail plane creates a downforce that counteracts disturbances causing a nose-down pitching moment. It helps restore the aircraft to a stable pitch attitude.
How does airspeed affect the corrective moment in an aircraft?
-The faster the aircraft travels, the stronger the corrective moment will be, as it is dependent on the dynamic pressure (related to the square of the airspeed).
What is the significance of the coefficient of moment in stability analysis?
-The coefficient of moment is a useful tool in stability analysis as it represents the moment per unit dynamic pressure, per unit area, and per unit mean aerodynamic chord, simplifying the evaluation of an aircraft's stability characteristics.
What are the two types of dynamic stability in terms of pitch?
-The two types of dynamic stability in terms of pitch are short period oscillation, which is a quick fluttering motion, and long oscillation or fugoid oscillation, which occurs over a few minutes and involves larger changes in altitude and airspeed.
What is pilot-induced oscillation and how does it relate to short period oscillation?
-Pilot-induced oscillation is a phenomenon where the pilot's attempts to correct for short period oscillations can inadvertently worsen the situation, causing the oscillations to grow more severe due to being out of sync with the quick changes in pitch.
How does directional stability differ from lateral stability?
-Directional stability, which involves the yawing motion, is the initial tendency of an aircraft to return to its original heading after a disturbance. Lateral stability, on the other hand, is the tendency of an aircraft to return to a wings-level state after a disturbance in roll.
What is the role of the fin in directional stability?
-The fin, or vertical stabilizer, provides the corrective yawing moment necessary for directional stability. It responds to the angle of side slip, creating a force that rotates the aircraft around its center of gravity to realign with the airflow.
How can the strength of the corrective moment in yaw be increased?
-The strength of the corrective moment in yaw can be increased by having a forward center of gravity, increasing airspeed, enlarging the fin area, or adding dorsal and ventral fins to increase the overall surface area contributing to the yawing moment.
What is the relationship between roll and yaw in terms of aircraft stability?
-Roll and yaw are linked in aircraft stability. An uncoordinated roll (without rudder input) can lead to a yaw due to the difference in lift on each wing, and a yaw can lead to a roll if not countered by coordinated control inputs.
What is Dutch roll and how does it relate to lateral and directional stability?
-Dutch roll is a wobbling motion that occurs when there is strong lateral stability but weak directional stability. It involves a combination of roll and yaw oscillations, and can be mitigated with better coordination between the rudder and ailerons or through the use of a yaw damper.
Outlines
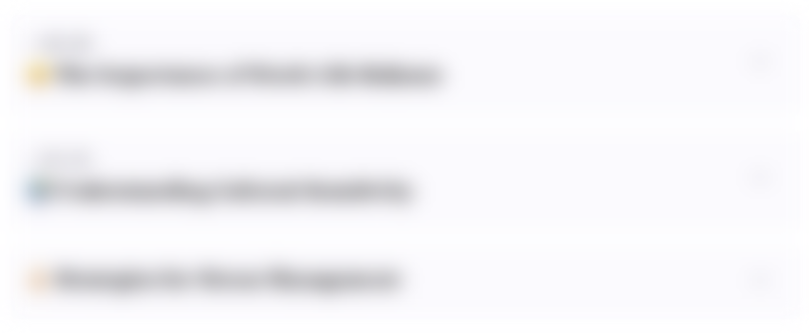
このセクションは有料ユーザー限定です。 アクセスするには、アップグレードをお願いします。
今すぐアップグレードMindmap
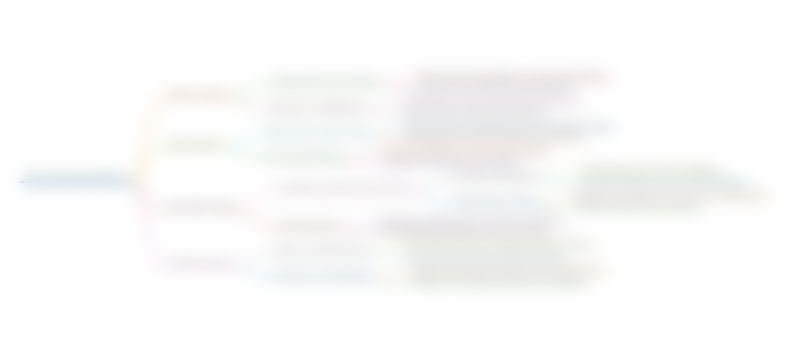
このセクションは有料ユーザー限定です。 アクセスするには、アップグレードをお願いします。
今すぐアップグレードKeywords
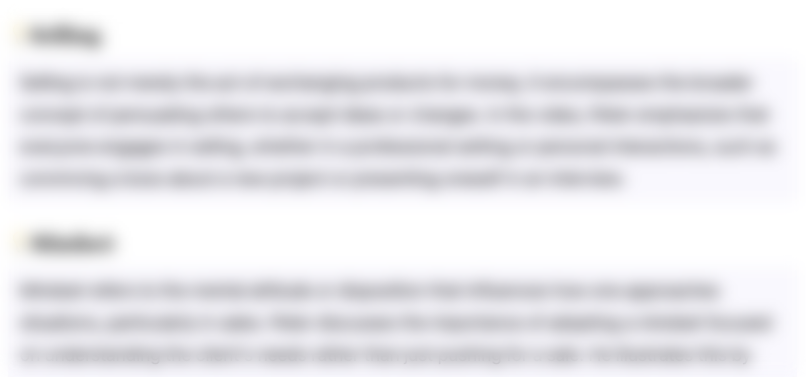
このセクションは有料ユーザー限定です。 アクセスするには、アップグレードをお願いします。
今すぐアップグレードHighlights
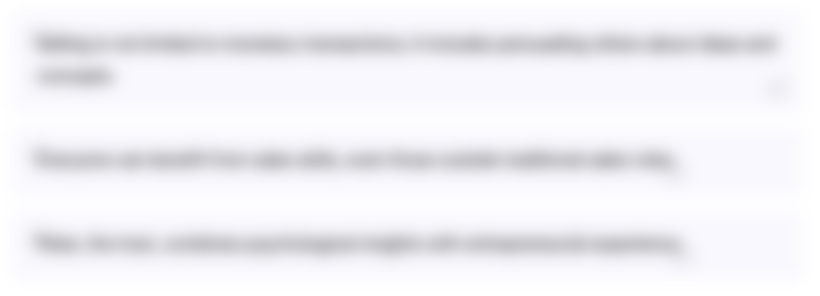
このセクションは有料ユーザー限定です。 アクセスするには、アップグレードをお願いします。
今すぐアップグレードTranscripts
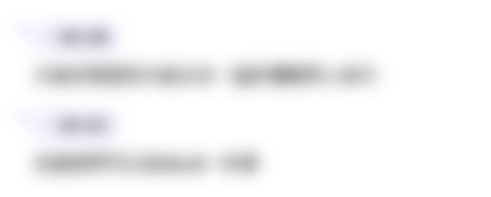
このセクションは有料ユーザー限定です。 アクセスするには、アップグレードをお願いします。
今すぐアップグレード関連動画をさらに表示

Lateral Stability Of Aircraft | Aircraft Lateral Stability | Lecture 41

ATPL Principles of Flight - Class 16: Stability I.
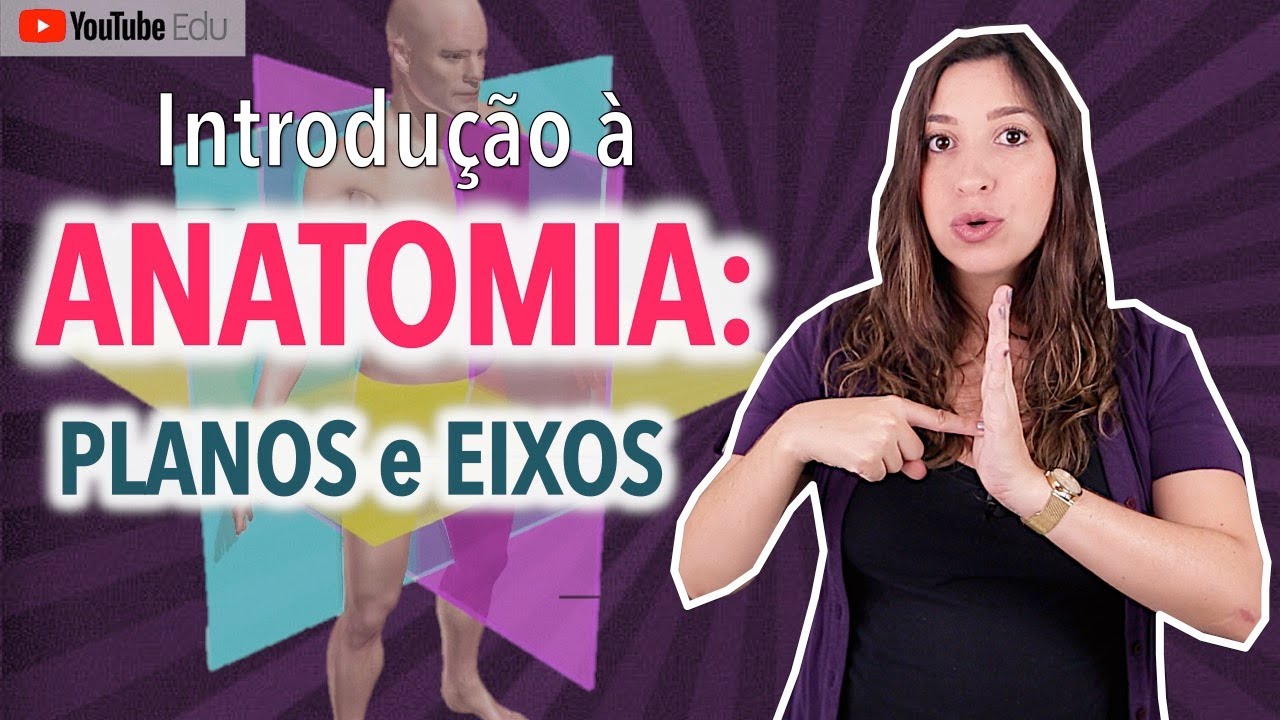
Planos Anatômicos e Eixos de Movimento: Introdução à Anatomia parte 2
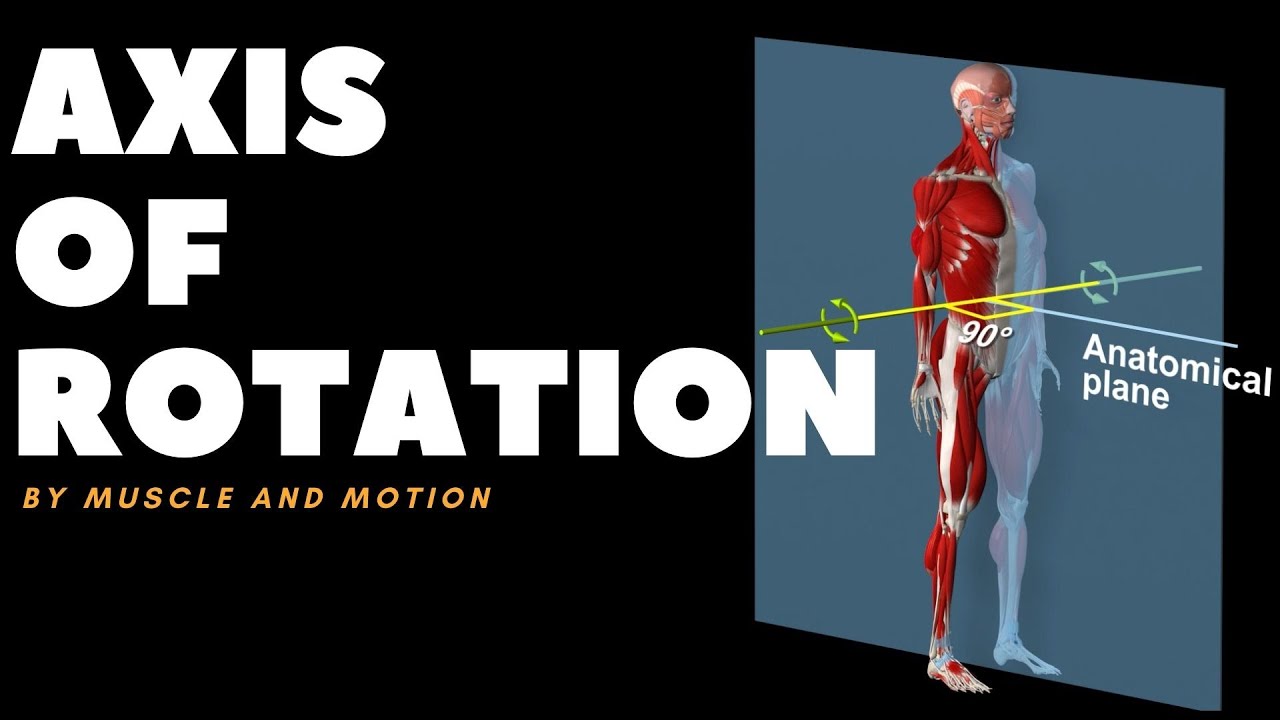
Understanding Axes of Movement/Rotation

ATPL Principles of Flight - Class 13: Controls.
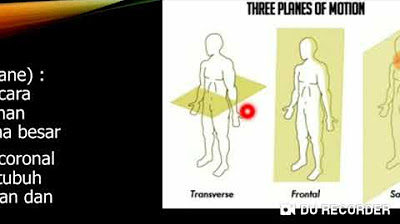
ANATOMI GERAK MANUSIA (BAG. 1) : ISTILAH ARAH, BIDANG ANATOMIS DAN SUMBU ANATOMIS.
5.0 / 5 (0 votes)