EMC tutorials - Magnetic field shielding
Summary
TLDRThis video delves into the nuances of electronic circuit shielding, focusing on magnetic field protection. It explores two primary shielding methods: magnetic flux deviation using permeable materials like ferrite, and magnetic field repulsion via eddy currents in conductive materials. The presenter conducts experiments to measure shielding effectiveness, emphasizing the importance of material properties like permeability and conductivity. The discussion highlights how these properties influence shielding at different frequencies, providing practical insights for designing effective magnetic shields.
Takeaways
- 🌐 Magnetic shielding is crucial for protecting electronic circuits from magnetic fields, especially in the near field where magnetic fields can have a more significant impact than electric fields.
- 🛡️ There are two primary methods for magnetic field shielding: magnetic flux deviation using magnetically permeable materials, and magnetic field repulsion using conductive materials that induce eddy currents.
- 🧲 Magnetically permeable materials, like ferrite, draw magnetic field lines through themselves, thereby reducing the field in the space behind the shield.
- 🔩 The effectiveness of magnetic shielding materials is influenced by their thickness and magnetic permeability, with thicker materials generally providing better shielding.
- 💧 Eddy currents, induced in conductive materials by varying magnetic fields, generate their own magnetic fields that oppose and can cancel out the original magnetic field.
- 🔌 The conductivity and permeability of conductive materials are key factors in their ability to shield against magnetic fields, with higher conductivity leading to stronger induced currents and better shielding.
- 📊 Shielding effectiveness can be calculated using the skin depth of a material, which is frequency dependent and affects how deep currents penetrate the material, influencing the shielding performance.
- 📉 As frequency increases, the attenuation (shielding effectiveness) also increases, but not at a constant rate due to the frequency-dependent nature of material properties like permeability.
- 📈 The permeability of materials like steel decreases with increasing frequency, which affects their shielding effectiveness at higher frequencies compared to lower frequencies.
- 🔄 The choice of shielding material and its thickness should be tailored to the specific frequency range and application, as there is no one-size-fits-all solution for magnetic shielding.
Q & A
Why is it important to consider magnetic fields separately from electromagnetic waves for shielding?
-Magnetic fields should be considered separately from electromagnetic waves because their effects can differ based on distance and wavelength. In the near field, where you are close to a noise source, the impedance of the electric and magnetic fields are different, which can lead to one being more damaging than the other. Therefore, it's important to create a shield specifically for the type of noise you want to shield against.
What are the two main methods of magnetic field shielding discussed in the script?
-The two main methods of magnetic field shielding discussed are magnetic flux deviation using magnetically permeable materials, and magnetic field repulsion by relying on eddy currents induced into conductive materials.
How does magnetically permeable material shield against magnetic fields?
-Magnetically permeable materials shield against magnetic fields by providing a path of least resistance or highest permeability for the magnetic field lines to follow. The field lines tend to go through these materials rather than the air around them, closing back to the source. If the material is thick and permeable enough, all field lines that touch it go through it, protecting the space behind it from magnetic fields.
What is the role of eddy currents in magnetic shielding?
-Eddy currents play a crucial role in magnetic shielding when using conductive materials. When a variable magnetic field hits the conductive material, it induces currents known as eddy currents. These currents generate their own magnetic field that opposes the original field, ideally leading to a cancellation of the magnetic fields on the other side of the conductive plate.
Why is the thickness of the shielding material important for its effectiveness?
-The thickness of the shielding material is important because it affects how much of the magnetic field is blocked. Thicker materials provide a longer path for the magnetic field lines to follow, which can lead to a more effective shielding. Additionally, the shielding effectiveness is related to the skin depth of the material, which is frequency dependent, and every skin depth provides an attenuation of 8.7 decibels.
How does the frequency of the magnetic field affect the shielding effectiveness?
-The frequency of the magnetic field affects the shielding effectiveness because the induced currents, which are responsible for creating the opposing magnetic field, are frequency dependent. Higher frequencies can induce stronger currents, leading to better shielding. However, the permeability of the material, which is crucial for the generation of the opposing field, can decrease with increasing frequency, affecting the overall shielding performance.
What is skin depth and how does it relate to magnetic shielding?
-Skin depth is a parameter that considers both electrical conductivity and magnetic permeability to calculate how deep currents run through a material. In the context of magnetic shielding, every skin depth of a material provides an attenuation of 8.7 decibels, meaning the thicker the material, the more skin depths it has, and the better the shielding it provides.
How does the permeability of a material affect its shielding effectiveness?
-The permeability of a material affects its shielding effectiveness because it determines how strongly the induced current can generate a magnetic field to oppose the original field. Higher permeability allows for stronger opposing fields, leading to better shielding. However, permeability is not constant and decreases with increasing frequency, which can impact the shielding effectiveness at higher frequencies.
What is the significance of the frequency-dependent behavior of permeability in shielding materials?
-The frequency-dependent behavior of permeability in shielding materials is significant because it influences the material's effectiveness at different frequencies. At lower frequencies, materials with high permeability like steel can provide better shielding. However, as frequency increases, the permeability of these materials decreases, and the most conductive materials become more effective for shielding.
What practical considerations are there for choosing a shielding material based on the script's discussion?
-Practical considerations for choosing a shielding material include the frequency range of the magnetic fields to be shielded against, the permeability and conductivity of the material, the thickness of the material, and the specific application or environment in which the shielding is needed. For example, for audio range frequencies, high permeability materials like steel might be necessary, while for higher frequencies, thinner conductive materials or even conductive paint might suffice.
Outlines
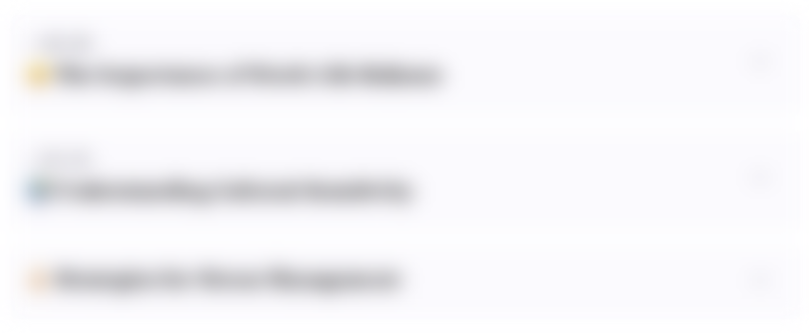
このセクションは有料ユーザー限定です。 アクセスするには、アップグレードをお願いします。
今すぐアップグレードMindmap
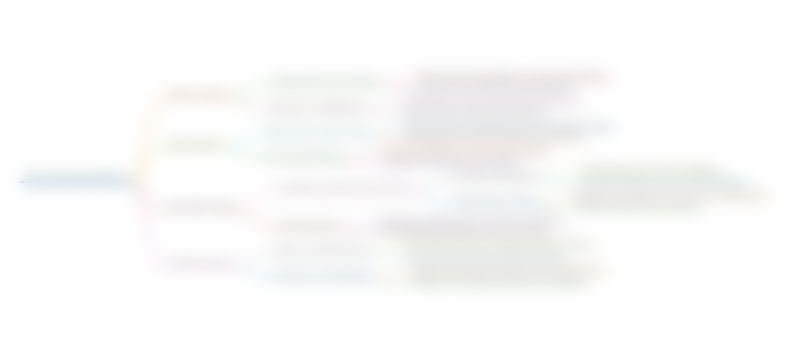
このセクションは有料ユーザー限定です。 アクセスするには、アップグレードをお願いします。
今すぐアップグレードKeywords
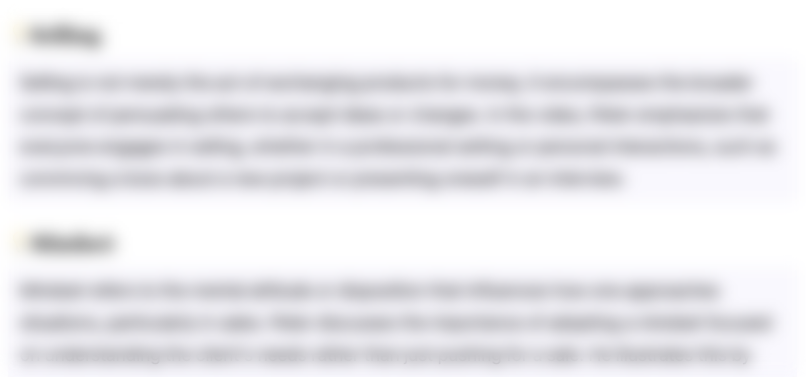
このセクションは有料ユーザー限定です。 アクセスするには、アップグレードをお願いします。
今すぐアップグレードHighlights
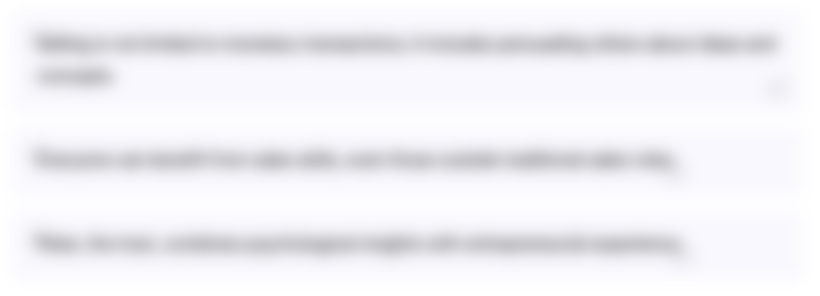
このセクションは有料ユーザー限定です。 アクセスするには、アップグレードをお願いします。
今すぐアップグレードTranscripts
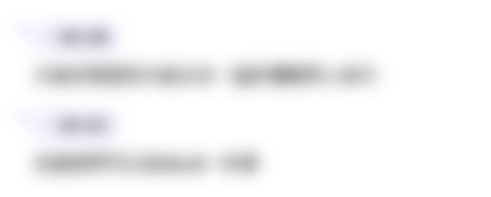
このセクションは有料ユーザー限定です。 アクセスするには、アップグレードをお願いします。
今すぐアップグレード5.0 / 5 (0 votes)